
9 minute read
Genetically engineering ocular probiotics to manipulate ocular immunity and disease
Yannis Rigas a, b , Benjamin Treat b , Anthony St. Leger b, c a Department of Bioengineering, University of Pittsburgh Swanson School of Engineering, Pittsburgh, PA, USA b Department of Ophthalmology, University of Pittsburgh Medical Center, Pittsburgh, PA, USA c Department of Immunology, University of Pittsburgh Medical Center, Pittsburgh, PA, USA
Yannis Rigas
Dr. Anthony St. Leger
Yannis Rigas is a current bioengineering major on the track of cellular engineering. His current research interests include microbiology, immunology, genetic and microbial engineering. Yannis plans to attend graduate school upon graduating from the University of Pittsburgh.
Dr. St. Leger is an ocular immunologist in the Department of Ophthalmology at the University of Pittsburgh. Since joining Pitt in late 2017, his lab has focused on understanding the relationships between the microbiome and ocular immune system during health and disease. Specifically, his lab currently investigates how the microbiome may be manipulated to alleviate ocular surface disease.
Significance Statement
Every year eye-related diseases account for a huge financial and medical burden. For example, in the U.S., approximately 1 million doctor visits and $175 million are spent annually on treating keratitis and contact lens disorders [1]. In an effort to characterize and manipulate the interactions between ocular immunity and microbiome, our lab is developing a model to characterize and manipulate the relationship between the microbiome and ocular immunity. To study this, we are developing a model that allows the study of commensal bacterium, Corynebacterium mastitidis, and its interactions with ocular immunity. The long-term aim of these studies is the development of a vehicle for long-term therapy delivery to the ocular surface in order to help treat ocular diseases.
Category: Methods Keywords: Ocular immunity, genetic/microbial engineering, probiotic, and microbiology Abbreviations: C. mast (Corynebacterium mastitidis), IL-17 (Interleukin 17), γδTC (gamma delta T-cells), WT (Wild Type)
Abstract
Recently, our lab discovered that the eye harbors a microbiome that includes Corynebacterium mastitidis, which can stimulate local immunity to protect the eye from more serious infections. From our previous work, we know that C. mast can remain on the ocular surface indefinitely. Therefore, the goal of this project is to genetically engineer C. mast, so that it can act as a natural vehicle to deliver therapeutics locally to alleviate or prevent ocular surface diseases. Here, we took initial steps towards this goal by genetically modifying the C. mast genome so that a fluorescent protein is selected for by utilizing an antibiotic resistance cassette. This fluorescence will allow for real-time in vivo detection of genetically modified C. mast. In this study we have discovered four possible mutants that are resistant to the antibiotic, kanamycin, and fluoresce with varying degrees intensity in the red channel. We hypothesized that these mutants would retain the ability to colonize the eye and induce immunity similar to wild type C. mast. Indeed, we observed that all four mutants were able to colonize the eyes of mice and elicit immune responses similar to wild type C. mast. We further demonstrated that genetically engineered strains of C. mast can effectively colonize the ocular mucosa and elicit an immune response similar to WT C. mast.
1. Introduction
Previously, research on the ocular surface has shown that Corynebacterium mastitidis is stably present on the conjunctiva, an ocular mucosal immune tissue, while displaying commensal properties by eliciting an immune response from γδT cells. The presence of this commensal bacterium, and its accompanying immune response were previously shown to protect the eye from pathogenic eye infections caused by Pseudomonas aeruginosa and Candida albicans. [2]. Through a series of experiments, we were able to link ocular colonization of C. mast with an induced antimicrobial immune response [2].
Due to the ability of this microbe to asymptomatically thrive at the ocular surface for indefinite periods of time, C. mast is an attractive candidate to engineer as a long-term drug delivery vehicle for inflammatory diseases like keratitis, Dry Eye Disease, and Sjogren’s Syndrome. A similar technique was used to deliver the immune regulating cytokine, interleukin (IL)-10, in mice with inflammatory bowel syndrome (IBS). More specifically, when Lactococcus lactis was engineered to express IL-10, disease associated with colitis was reduced compared to controls in two separate mouse models [3].
In this current study, we successfully genetically engineered C. mast by electroporation with a novel plasmid, and showed that C. mast can stably remain on the ocular surface and elicit an immune response similar to the WT strain. This discovery will pave the way for future modifications that will one day allow for a longterm delivery of therapeutics to the ocular surface.
2. Methods
We modified C. mast using a custom synthetically designed plasmid constructed from gBlocks (Integrated DNA Technologies, Coralville, Iowa) containing genes for the codon-optimized expression of mCherry, kanamycin resistance, and a mariner transposase. We then tested the candidates for the ability to colonize C57BL/6 mice and induce an immune response that is similar to the WT strain of C. mast. Mice were inoculated three times over 6 days, and three weeks after the final inoculation, mice were sacrificed and flow cytometry was performed in order to determine if mice exhibited stimulation of IL-17 and neutrophils.
Figure 1: Visual representation of plasmid with transposase, mCherry, and Kanamycin resistance
Further iterations of this plasmid, containing a native origin of replication, were derived from the native cryptic plasmid already present in C. mast. We were able to integrate our plasmid into C. mast through both electroporation and conjugation. For electroporation, the induced pore formation in the bacterial cell wall allowed for the entry of the plasmid into the cytosol of the cell. From there, the transposase coordinates random transposon integration into the bacterial genome. For conjugation, a cell to cell interaction allowed for the transfer of our plasmid from the “donor” S17 E. coli to our target, C. mast. After successful uptake of plasmid DNA, the mariner transposase coordinated insertion of both the mCherry and kanamycin resistance gene. We screened for successful integration of our transposon by incorporating stringent selection with kanamycin (25 µg/mL) that would select for our transposon insertions. Candidates were further screened under a fluorescent microscope to detect red fluorescence which would indicate mCherry expression. Since mCherry expression will depend on genomic location of the transposon, we reasoned that the level of fluorescence would positively correspond with the activity of the promoter that would guide the expression of these genes. This system will allow us to identify novel promoters and genomic locations for future genetic modifications. Colony polymerase chain reaction for the mCherry gene eliminated any candidates that may have been autofluorescent due to stress or unwanted adaptive mutations and ensured only transposon mutants were chosen. In addition, flow cytometry was used in order to observe the amount of fluorescence in order to quantitatively analyze how bright our mutants are compared to WT C. mast.
86 Undergraduate Research at the Swanson School of Engineering Figure 2: Timeline of mouse inoculation.
Flow cytometry was performed by isolating single cell suspensions of mouse conjunctiva and lymph node tissues, staining fixed and permeabilized cells with an antibody panel (that includes α-CD45, α-γδTCR, α-Ly6C, α-Ly6G, and α-IL-17 [Biolegend]) each labeled with unique fluorophores. Samples were run on a Cytoflex LX (check) and analyzed for cell populations. This response was compared to a control group of mice inoculated with WT C. mast to observe if the mutants had a similar impact. A one-way ANOVA was used to test whether any of our results were significant.
3. Results
Despite all the candidates maintaining kanamycin resistance, there were variable levels of fluorescence. Although all mutants had variable levels of fluorescence, each candidate expressed more fluorescence than the wild type C. mast. In fact, one candidate expressed 5-fold more fluorescence compared to other candidates as detected by flow cytometry for mCherry expression (Figure 3B-C).
Upon inoculation of mice, all four mutants were able to successfully colonize the eye, suggesting that the transposon insertion did not affect any genes absolutely necessary for colonization. The decreased level of colonization correlates with the lower number of neutrophils and IL-17 producing cells as seen in Figure 4. In addition, mutants obtained from mice two weeks postcolonization were not able to colonize as efficiently.
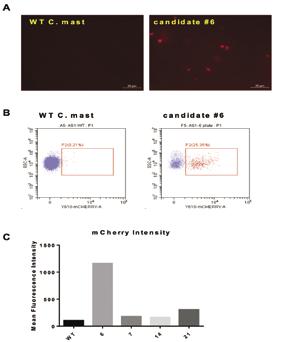
Figure 3: Screening C. mast transposon insert candidates for mCherry expression. A. Fluorescence microscopy of individual WT C. mast vs transposon candidate bacteria. Flow cytometry measuring WT or transposon candidate mCherry expression levels (B) and mean fluorescence intensity (C) in WT and candidate strains (6,7,14,21) of C. mast. Experiment was repeated with similar results.

Figure 2: Flow cytometry measure amount of IL-17-producing γδTCR and neutrophils present due to inoculation of 4 candidates as well as WT C. mast. Dots represent individual values with value bars representing mean and standard deviation. Signifigance was determined by One-way ANOVA with Dunnett’s multiple comparisons test. * represents p<0.05, ** represents p<0.005 as compared to WT C. mast
4. Discussion
The varying amounts of fluorescence was to be expected as transposon insertions are notoriously variable, and fluorescence is more present when in an actively transcribed section of the genome. Although fluorescence was variable between the four mutants, it is promising that all candidates harbored more fluorescence than the WT C. mast. In addition, the fact that all four samples were able to colonize and induce an immune response means that we have not interrupted genes required for colonization and/or ocular immunogenicity. However, the reduced colony size and dampened immune response may correlate with changes to genes of intermediate importance. Genomic sequencing of transposon insertions will allow us to determine this.
5. Conclusion
In this study, we have genetically modified C. mast to express mCherry and Kanamycin resistance and showed that even with these modifications the ability to colonize the ocular mucosa and elicit an immune response in mice is still present. After obtaining these four mutants, we are attempting to optimize the integration of genetic material by altering the native plasmid present in C. mast in order to more efficiently introduce genes for mCherry and kanamycin resistance. This will allow us to screen a greater number of transposon mutants, and will further aid in rational design of future constructs. By genetically engineering C. mast we have also paved the way for further genetic manipulations that can lead to the development of future therapeutics for ocular diseases.
6. Acknowledgments
Anthony St. Leger, PhD, Dana Previte, PhD, Kate Carroll, PhD, Hongmin Yun, PhD, Heather Buresch, the Swanson School of Engineering, and the Office of the Provost.
7. References
[1] Sarah A. Collier, MPH, Michael P. Gronostaj, MD, PharmD, Amanda K. MacGurn, MPH, Jennifer R. Cope, MD1 Kate L. Awsumb, MA, MPH, Jonathan S. Yoder, MPH, MSW, Michael J. Beach, PhD, Estimated Burden of Keratitis – United States, 2010, Morbidity and Mortality Weekly Report. 63(45) 1027-1031. [2] Anthony J. St. Leger, Jigar V. Desai, Rebecca A. Drummond, Abirami Kugadas, Fatimah, Almaghrabi, Phyllis Silver, Kumarkrishna Raychaudhuri, Mihaela Gadjeva, Yoichiro Iwakura, Michail S. Lionakis, Rachel R. Caspi, An Ocular Commensal Protects against Corneal Infection by Driving an Interleukin-17 Response from Mucosal γδ T Cells, Immunity, 47(1), 148-158. e5. [3] Lothar Steidler, Wolfgang Hans, Lieven Schotte, Sabine Neirynck, Florian Obermeier, Werner Falk, Walter Fiers, Erik Remaut, Treatment of Murine Colitis by Lactococcus lactis Secreting Interleukin-10, Science, 289(5483) 1352-1355.