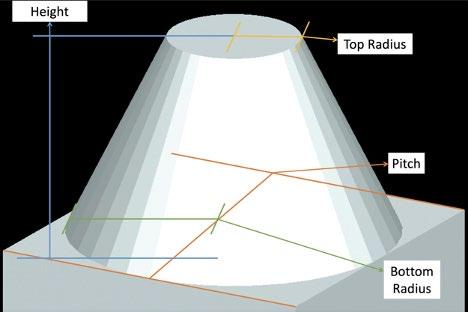
8 minute read
u Simulating the effect of different structures and materials on OLED extraction efficiency
Simulating the effect of different structures and materials on OLED extraction efficiency
Benjamin Bailey, Paul Leu
Department of Industrial Engineering
Ben Bailey Ben Bailey was born and raised in Mount Pleasant, Pennsylvania. His research interests are nanostructures and using them to increase efficiency in OLEDs.
Dr. Leu is an Associate Professor in the Department of Industrial Engineering and the Department of Mechanical Engineering and Materials Science. He received his BS in Mechanical Engineering at Rice University in 2002, his MS from Stanford University in 2004, and his PhD from Stanford UniversityPaul Leu, Ph.D. in 2008. Dr. Leu’s lab research focuses on designing and understanding advanced materials by computational modeling and experimental research.
Significance Statement
Organic light-emitting diodes (OLEDs) have lower efficiency than other types of lights. With a lower efficiency, more power is required to use OLEDs. Using microstructures on the surface of the OLED increases efficiency by decreasing the reflectivity of the light generated within the structure.
Category: Computational Research
Keywords: OLED, EE, Nanocone, FDTD
Abstract
In order to increase the extraction efficiency (EE), the transmission of light generated in the structure must be maximized. With a maximized EE, there is less power required to produce the same amount of light. This goal is achieved by using surface structures on top of the glass layer of an organic light-emitting diode (OLED) device. Among all the available structures, we focus on the nanocones in this research, because the nanocone structures in previous studies displayed more extraction enhancement than other simple structures. Also, this research is a basis to begin a larger project, with more complicated structures, that time limitations prevented us from going deeper into. Using finite-difference time-domain (FDTD) analysis, the size of the nanocones can be optimized to maximize EE. It was found that minimizing the surface area not covered by nanocones, as the distance between nanocones was the largest factor in the simulations.
1. Introduction
Organic light-emitting diodes (OLEDs) are devices that emit light when electricity is transferred from a cathode, through an organic emissive layer, to an anode. Light is emitted from the emissive layer and extracted from the device. The efficiency of the OLED describes how much power is output by light compared to the electrical input. High efficiency OLEDs are desirable as less electricity, or energy, required to light anything from a smart card with an OLED display to lighting devices for homes and businesses. The purpose behind this project is to maximize the efficiency of OLED devices by optimizing nanocone structures in a square lattice symmetry.
Lim et al. studied the efficiency of different nanostructures on the surface of OLEDs; they found that the external quantum efficiency (EQE) of nanocones in a square lattice symmetry is fourteen to thirty-five percent [1]. Kim et al. corroborated the results of the nanocone structures, reaching 31.8 percent extraction efficiency (EE) [2]. This study will begin the study of EE using various nanostructures to continue increasing the efficiency of OLEDs.
Since light is reflected back into the device when entering a flat layer, it could be hypothesized that the angle of the sides of the nanocones will be the most important factor. This angle is from changing the top radius, the bottom radius, and the height of the cone. The larger the difference between the two radii along with a low height would give a smaller angle, while decreasing the difference between the two radii and increasing the height would increase the angle.
This study differs from other studies by comparing the different sizes of nanocones used to increase EE. Also, the majority of past research on this topic was done in a lab, while the work shown in this paper is simulated using FDTD analysis.
2. Methods
The study consisted of using the computer software Lumerical to run the finite difference time domain simulations [3]. Within Lumerical, a script was written and used to optimize a set of variables: pitch, cone height, radius at the top of the cone, radius at the bottom of the cone. The script is stopped when the radius at the top of the cone reaches the radius of the bottom of the cone, since the size of top cannot exceed the size of the bottom. The script is also stopped when the diameter at the bottom of the cone reaches the size of the pitch. The source type used was a planar source at the point (0, 0, 0.01), the FDTD is an auto non-uniform mesh, with all PML boundary conditions.. Table 1 below shows the positions and dimensions of each layer. Figure 1 below shows the different variables.
Layer x y z x span y span z span Material
Cathode 0 0 0.05 Pitch Pitch 0.1 Aluminum
Organic Layer 0 0 0.19 Pitch Pitch 0.18 No specification
Anode 0 0 0.38 Pitch Pitch 0.2 ITO Dielectric
Backfill 0 0 0.78 Pitch Pitch 0.6 SiN Dielectric
Glass 0 0 2.58 Pitch Pitch 3 SiO2
FDTD 0 0 2.5 Pitch Pitch 4.3
dx, dy dz x,y z x span y span z span
Mesh 0.025 0.015 0 2.5 Pitch Pitch 4.3
Table 1. Positions and dimensions of different layers, all in micrometers, as well as mesh information.
Figure 1. Variables of the cone. The shown figure is “Trial 1” from Table 2.
Using Lumerical, the internal structure of the OLED is represented by different refractive indexes within the software. Therefore, the specific materials used in the simulation are not important, as the only efficiency loss tested is light lost after the organic layer.
The efficiency stated is from the transmission monitor at 550 nanometers; the center of visible light on the electromagnetic spectrum, as this is the middle of the visible range of the electromagnetic spectrum. The EE is calculated by the ratio of the total energy emitted from the source and the total energy transmitted through the surface of the device, as seen in the appendix.
3. Results
The most efficient results were found with a pitch of 100 nanometers, a cone height of 100 nanometers, a top radius of 10 nanometers, and a bottom radius of 50 nanometers. With a pitch and bottom radius equal, the surface area covered is minimized. At a wavelength of 550 nanometers, the maximum efficiency reached in the study was 15.1 percent, compared to 13.0 percent efficiency (a 16.2 percent increase) for flat glass with no nanostructures. This matches perfectly with the findings of Lim et al. when the study has a 13 percent efficiency- the nanocones increase the efficiency to 15 percent [1]. Figure 2 shows the EE across the entire electromagnetic spectrum, while Table 2 shows results comparing different extraction enhancements.
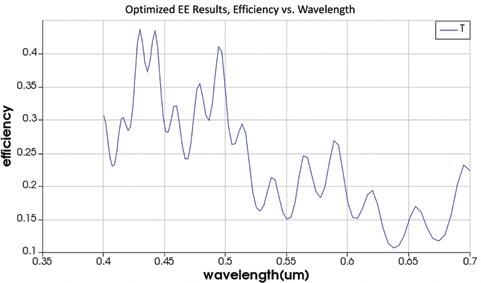
Figure 2. Efficiency vs. Wavelength. Shows the graph of the efficiency against the wavelength of light.
Trial Pitch (nm) Height (nm) Top Radius (nm) Bottom Radius (nm) EE (%) Enhancement (%)
No cone N/A N/A N/A N/A 13 N/A
1 100 100 10 50 15.1 16.2
2 100 100 10 49 14.7 13.1
3 100 100 10 48 14.2 9.2
Table 2. Various trials with percent increase
The light that comes out of the OLED device is at its highest intensity at the center of the nanocone, while it is at its lowest at the uncovered glass surface. Figure 3 shows the energy field that is emitted from the device.
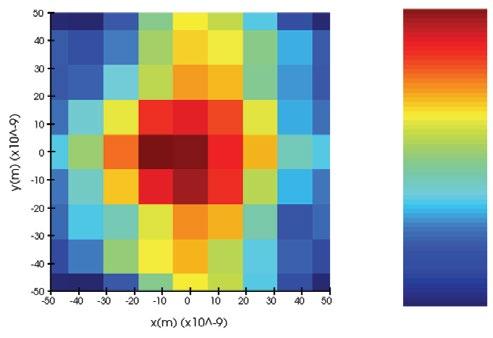
Figure 3. Energy field. This shows the intensity of energy that is coming out of the surface of the OLED. The red is where the intensity is the highest (in the cone), while the blue is where the intensity is the smallest (uncovered glass surface).
4. Discussion
Throughout the simulations, data suggests that efficiency is lost where there is more space not covered by the nanocone. When the pitch is much larger than the diameter- a cone with an equal diameter and pitch would touch at the base- there is a large amount of space uncovered by the nanocone. Also, the best results were all from the same height- 100 nanometers. This suggests that the angle of the incline combined with the amount of uncovered surface area are the greatest factors on efficiency. As the wavelength increases, the EE decreases. This is due to stress relaxation, piezoelectricity-induced quantum confined Stark effect, and stress relaxation [4]. Although, the first half of the visible light spectrum has a much higher EE- a result from a lack of these phenomena. This explains the disparity in results from Kim et al. and this study- all wavelengths tested at once against each wavelength individually across the spectrum.
5. Conclusions
In summary, using nanocones to increase the EE of an OLED device is effective. Eliminating the uncovered glass surface with nanocones was the largest factor on EE, because that is where the most light gets reflected back into the device. This was determined because the EE was the greatest when the diameter of the bottom radius of the cone was equal to the length of the pitch, so the area of uncovered glass was minimized.
6. Acknowledgements
Funding for this project was provided by the Swanson School of Engineering, the Office of the Provost, and Dr. Paul Leu.
7. References
[1] Lim, Tae-Bin, Kwan Hyun Cho, Yong-Hoon Kim, and Yong-Cheol Jeong, ‘Enhanced Light Extraction Efficiency of OLEDs with Quasiperiodic Diffraction Grating Layer’, Optics Express, 24.16 (2016), 17950–59 <https://doi.org/10/gg686t> [2] Kim, Yangdoo, Kwan Kim, Daehong Huh, and Heon Lee, ‘Improvement of Light Extraction Efficiency of OLED Using Various Optical-Functional Nano-Structures’, Ceramist, 21 (2018), 64–79 <https://doi.org/10.31613/ceramist.2018.21.1.06> [3] ‘Nanophotonic FDTD Simulation Software - Lumerical FDTD’, Lumerical, Last visited 11 November 2020 <https://www.lumerical.com/products/fdtd/> [4] ‘External Quantum Efficiency - an Overview | ScienceDirect Topics,’ Last visited 11 November 2020 <https://www.sciencedirect.com/topics/engineering/external-quantum-efficiency>
8. Appendix
Eq. 1: EE = (Total Energy Transmitted)/(Total Energy Created)