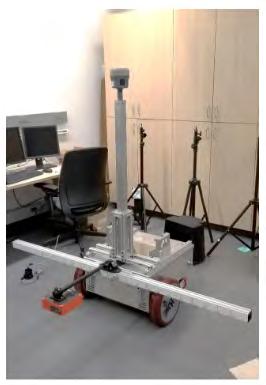
6 minute read
Figure 27: A prototype of MnDOT remotely operated rolling asphalt density meter
Figure 27: A prototype of MnDOT remotely operated rolling asphalt density meter
Specifically, within the DPS system, GOR sensors send and receive the EM waves while recording the amplitudes and frequency of the signals. The signal is processed using a concentration box to determine the necessary amplitudes and the on-board computer uses the amplitudes to compute the dielectric constant of the pavement in real time. Dielectric constant is a measure of a material’s ability to store electrical energy. In the case of pavement engineering, this constant can be directly related to air void content and therefore density. A low dielectric value means the pavement will have a higher air void content and therefore a lower density. As a reference, the dielectric constants of air and water are 1 and 81, respectively, meaning EM waves travel much slower underwater. Asphalt is typically between 4 – 8, depending on the air content.
Ensuring accurate dielectric constants is crucial to predicting the air void percentage across the pavement. A slight change in dielectric constant can lead to a large change in air content. The AC surface reflection method is a popular way to determine the bulk dielectric constant of the HMA. It utilizes the ratio of the amplitude of the GPR signal reflection from air to the HMA surface, A0, to the incident amplitude (represented by the reflection from the metal plate), Ai. The dielectric constant of the surface is determined, using the Equation 1 [76]:
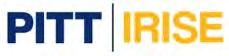
41
=#1+&'" '# 1−&'" '#
$* (1)
The dielectric constant values determined from Equation 1 can be empirically related to the relative ratio of pore volume to the total volume of the newly placed HMA lift because air has a lower dielectric constant than the surrounding HMA components, and the aggregate type and volumetric proportion are typically uniform [77, 78]. This requires a regression analysis to be performed using cores from each new specific HMA mix location. The equation format shown in Equation 2 is the common relation with A and B being calibration coefficient and d being the measured dielectric constant. This method has been shown to have a good relation and provide reasonable estimated of air content and density.
'+,./01!01(%)='!
%&( (2)
3.2.1.1 Advantages and Disadvantages
Using GPR systems for pavement analysis presents many benefits due to its nondestructive character. A SHRP2 study demonstrated positive potential of DPS use in projects for providing real-time density feedback after final mat compaction. Yet, the project also pointed out several needed improvements in the procedure for data collection, processing, and evaluation. The study required multiple passes at various transverse locations withing a lane because the study used GPR system with a single antenna. This increased survey time while also limiting the coverage area for some locations [77]. Geophysical Survey Systems, Inc. (GSSI) recently developed a three-sensor version of DPS to address this issue. These additional sensors can be placed from 1 to 2.5 feet away to cover significant areas of interest [63]. The three-sensor DPS increases testing productivity by being able to cover joints and wheel paths in a single survey pass.
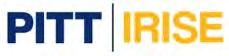
42
Using this updated version, a study done at Highway 52 near Zumbrota, Minnesota, was able to collect the compaction data using 3D GPR that was equivalent to taking over 1 million cores [79].
Using GPR systems also can provide safety advantages because data collection is much faster than coring and other methods. This allows workers to be removed from the presence of live traffic more often. A remote-controlled GPR would remove any need for a worker in the right of way. DPS also has collects compaction data for the entirety of the pavement surface which is beneficial for improved QA/QC in the field. Another strong benefit of DPS is the real time data evaluation. This provides knowledge of when and where additional roller passes are needed for immediate correction, or if there are any other great deficiencies [63].
Current disadvantages that arise from the use of air-coupled GPR technology include the following [79]:
• For layers < 1”, accuracy is affected by the underlying layer • For layers > 2.5”-3”, accuracy is affected by varying density gradients throughout layer • Effect of surface moisture • Change in aggregate source along pavement section • Temperatures below 40℉
The advantage of AC surface reflection method for determining dielectric constants is that if the upper lift is sufficiently thick (thicker than 1.2 inches (30 mm)), then the measured AC surface reflection depends only on the properties of the upper layer [63]. However, this advantage only applies to a tight range of thicknesses. If the upper layer is too thick the results may not be representative of the entire layer [79]. Surface moisture has shown to be detrimental to results because it interferes with GPR signals. Research involving different types of GPR and algorithms is being investigated to overcome this [70, 80]. This shortcoming makes hand driven devices preferred to those attached to rollers as rollers wet the asphalt as they compact to minimize adhesion of the asphalt to the roller [80]. The dielectric constant relationship also depends on the aggregates used in the pavement. Any major deviations in aggregate will significantly reduce the accuracy.
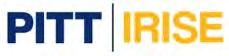
43
Reaching an accurate correlation between the dielectric constant and the HMA air voids is one of the biggest challenges of DPS use. Examples of poor correlations are common in the technical literature. The beforementioned SHRP2 study reported a poor correlation between the DPS-measured dielectric constants and air void contents for one implementation project. However, other non-destructive testing like nuclear density and infrared have also shown poor correlation with density under inadequate circumstances. The study concluded that heavy rains that occurred in the asphalt mix plant and the use of an experimental fiber-containing mix might have contributed to the poor results [77].
A 2015 study also reported poor correlations between measured dielectric constants and core-measured air void. However, in this particular project, measured air void content varied significantly (3 to 4.6 %). This variation, besides the use of a thicker layer, might have contributed to the poor correlation according to the authors [81].
3.2.1.2 Past and Present Experience
Past experience of using DPS to survey HMA air void content was compiled for a SHRP2 project in cooperation with Minnesota, Maine, and Nebraska DOTs [63]. This section summarizes the main findings of these field surveys conducted in projects representing a variety of construction techniques, HMA mix designs, and paving conditions and requirements.
Highway 52 near Zumbrota, Minnesota
This MnDOT project was divided into four test sections differed by mix design and number of compaction roller passes. Using DPS, the study was able to fully analyze the pavement surface producing millions of data points over the course of several weeks. In this study, results from the GPR dielectric data at the core locations were correlated with the air void measurements for all sections. Results of this survey produced mostly good correlations as seen in Figure 28 where the model explains almost 70% of variation in the air void content for Section 1. For these surveys, the data collection was taken on different days, different lanes, and at several weather conditions. In addition, the location of DPS measurements did not match the exact locations where cores were taken for some points. This testing variability can have an
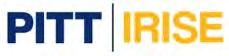
44