BIOCHAR GREEN IRON RESEARCH
Insights from the Luleå University of Technology.
Green iron corridors: a new advent of steel production.
The future of US Steel remains uncertain.
Potential impacts of a global green iron trade.
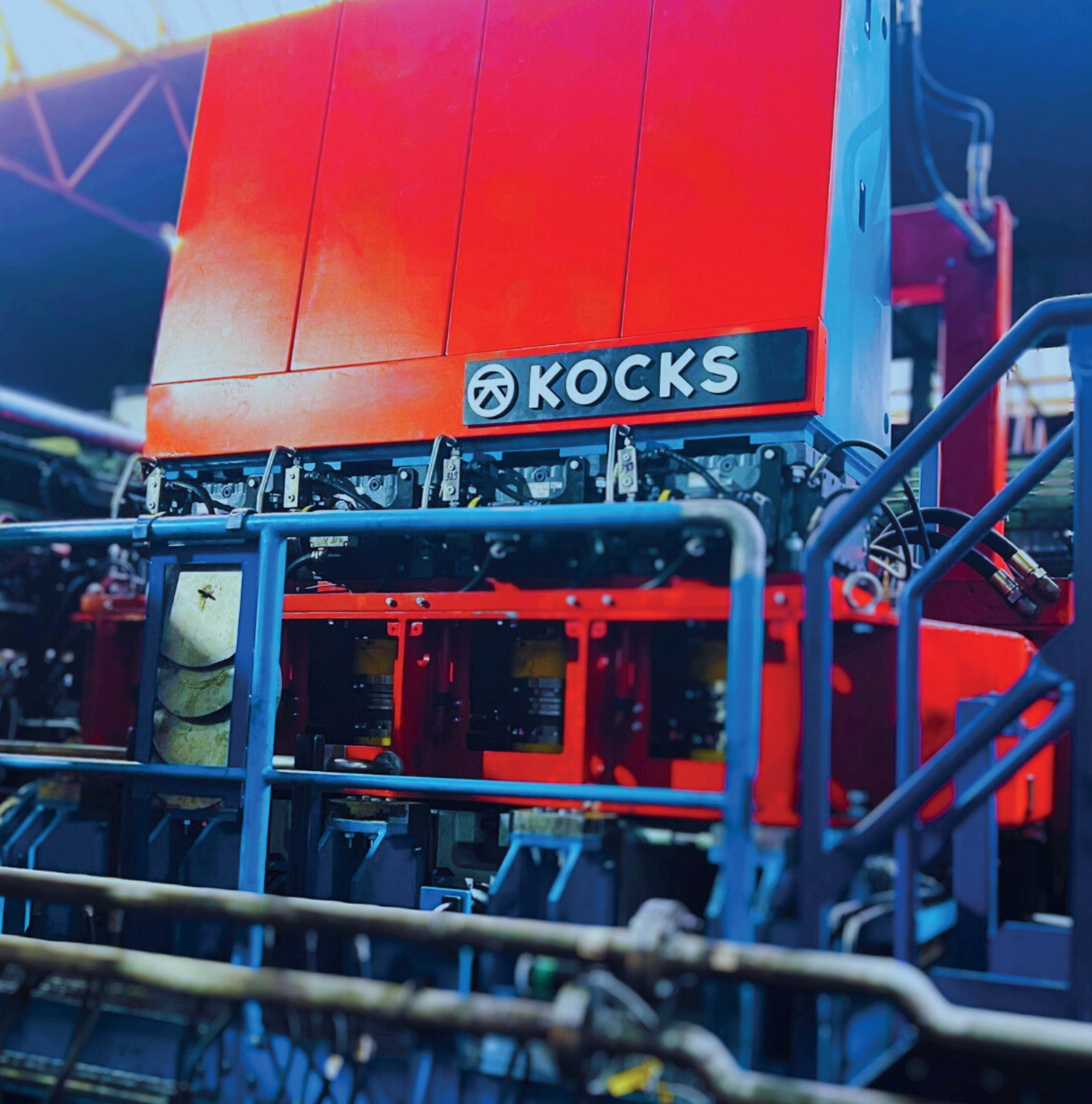
Insights from the Luleå University of Technology.
Green iron corridors: a new advent of steel production.
The future of US Steel remains uncertain.
Potential impacts of a global green iron trade.
Metals offers the entire steel value chain an exceptional opportunity for curbing their CO2 emissions.
The unique combination of its historic and recently developed product portfolio, make John Cockerill one of the industry’s most relevant suppliers of equipment for both the hot and cold phase of the steelmaking and processing industry.
Our three distinct business segments are addressing todays and tomorrow’s challenges supporting sustainable and green steel production:
Our new upstream offering related to DRI (Direct Reduced Iron), EAF (Electric Arc Furnaces) technologies and the use of hydrogen in steelmaking. Next to offering indirect electrification (DRI-EAF&H2-DRI-EAF),John Cockerill is also working on Volteron®: A first-of-a-kind iron reduction and steel processing route via direct cold electrolysis. This CO2 free steelmaking process, has been co-developed with the world’s leading steelmaker ArcelorMittal.
Regrouping our historical downstream product portfolio, this segment also includes:
¡ the Jet Vapor Deposition (JVD®) technology set to replace today’s hot-dip or electro galvanizing processes. This novel high-productivity vacuum coating technology provides previously unknown coating flexibility and possibilities, all while offering lower CAPEX and OPEX.
¡ our E-Si® equipment & processing lines specifically designed to produce high-quality Non-Grain Oriented (NGO) steel in response to the need for electrical steel meeting precise metallurgical properties, essential to support the shift towards green mobility.
This segment not only embraces all services and after-sales activities but will be strongly focusing on downstream furnace electrification (reheating and processing line furnaces), as well as hydrogen combustion, and the optimization of plant operations, including energy audits and the modernization of steel production equipment and installations.
EDITORIAL
Editor
Matthew Moggridge
Tel: +44 (0) 1737 855151
matthewmoggridge@quartzltd.com
Assistant Editor
Catherine Hill
Tel:+44 (0) 1737855021
Consultant Editor
Dr. Tim Smith PhD, CEng, MIM
Production Editor
Annie Baker
Advertisement Production Carol Baird
SALES
International Sales Manager
Paul Rossage
paulrossage@quartzltd.com
Tel: +44 (0) 1737 855116
Sales Director
Ken Clark
kenclark@quartzltd.com
Tel: +44 (0) 1737 855117
Managing Director
Tony Crinion
tonycrinion@quartzltd.com
Tel: +44 (0) 1737 855164
Chief Executive Officer
Steve Diprose
SUBSCRIPTION
Jack Homewood
subscriptions@quartzltd.com
Tel +44 (0) 1737 855028
Fax +44 (0) 1737 855034
Front cover photo courtesy of KOCKS
The KOCKS PWW 530/4, featuring 4-roll stands, installed at voestalpine Tubulars for the precise production of seamless hollow sections.
Catherine Hill Assistant editor catherinehill@quartzltd.com
In 1995, PhD student Suzanne Simard published her doctoral thesis: ‘Interspecific carbon transfer in ectomycorrhizal tree species mixtures’, which investigated the fungal webs of communication encountered in paper birch and Douglas fir trees. Two decades later, and now firmly established as a leading ecologist in the field of forestry and plant intelligence, Simard resides in the digital subterranea of Google as the woman who found out trees could talk. ‘Mother trees’, says Simard, act as central hubs, controlling vast underground mycorrhizal networks, sending warning signals and transferring their nutrients to neighbouring plants in their final months of life. Simard’s investigations into the interconnectivity of forests formed the basis of her book, ‘Finding the Mother Tree: Uncovering the Wisdom and Intelligence of the Forest’, published three years ago. In interviews, Simard has stressed that the usage of the word ‘mother’, which not only anthropomorphises its subject, but leans into the expansive cultural implications of ‘mothering’ and ‘motherhood’, is very much intentional. ‘‘If we can relate to it,
then we’re going to care about it more’’. Activists and conservationists like Simard, are left to toe a blurred line; between using interrelation as a weaponised tool to enact empathy, and the acceptance to view the disquieting reality of climate change as a viewpoint that doesn’t require weighted rhetoric, and is instead, compelling on its own scientific terms. In any case, shifts in thinking are taking place. Steelmakers are increasingly turning to more holistic approaches of production to establish a ‘green value chain’ that extends beyond the processes used in the mill, to the Romanesque ochre trenches of iron ore mines, and the ways in which material is extracted, converted, and applied. The finite nature of iron ore poses existential questions to the current configurations of the industry. Much like the networks that bind together Simard’s birch and fir trees, we are confronted by complex systems that simultaneously mimic the profound basis of connection so crucial to our own relationships, and expose our failure to protect them. ‘‘We’re at the last stems’’, Simard said recently. What remains, is our responsibility to defend.
Just as athletes rely on their teammates, we know that partnering with our customers brings the same level of support and dependability in the area of manufacturing productivity. Together, we can overcome challenges and achieve a shared goal, optimizing processes with regards to economic efficiency, safety, and environmental protection. Let’s improve together.
Do you want to learn more? www.endress.com
To be the premier partner in the metals industry, driving advanced technologies adoption and ensuring success throughout their lifecycle with dedicated and innovative services
The Towers & Structures business unit of Nucor Corporation, a Charlotte, North Carolina-based recycledcontent electric arc furnace steelmaker, will build its fourth overall and third new utility structures production facility in Brigham City, Utah. According to Nucor, the Utah plant will join two others it currently is completing in Decatur, Alabama, and Crawfordsville, Indiana.
Source: Recycling Today, 12 January 2025.
The Steel Authority of India and John Cockerill India will be investing nearly Rs 6,000 crore to set up a downstream plant for cold-rolled grainoriented and cold-rolled non-oriented electrical steel. Operations are slated to begin between 2027-2029 with a production target of 1.5Mt/ yr. The project is a result of a MOU signed in November 2022 between SAIL and John Cockerill India, with a joint goal to work on green steel technologies.
Source: Manufacturing Today, 13 January 2025.
Thyssenkrupp has said that a planned green steel site worth around €3 billion could go ahead even if government ambitions to build up a worldleading hydrogen industry fail. German opposition leader Friedrich Merz, tipped to become chancellor in next month's election, said that a fast shift to hydrogen
was unrealistic. While the government is in charge of ensuring a timely ramp-up of hydrogen infrastructure and supply to secure Europe's steel sector, the group's planned green steel site in Duisburg was not dependent on it, Thyssenkrupp said.
Source: Reuters, 14 January 2025
Ukraine has stopped production at its coking coal mine in Pokrovsk, which feeds the country’s steel industry, because of the proximity of advancing Russian forces, two industry sources told Reuters on Monday. The facility in the embattled city of Pokrovsk is Ukraine’s only mine that produces coking coal needed for the country’s once giant steelmaking industry, which has withered since Russia’s February 2022 invasion.
Source: Mining.com, 13 January 2025.
Swedish steelmaker SSAB has pulled out of $500 million green steel award negotiations. The Swedish company sought to make iron using green hydrogen in Mississippi, as part of the Biden administration’s $6 billion Industrial Demonstrations programme. While SSAB ‘continues the
Nord Steel, which operates three steel fabrication units in Lithuania, has committed to designing, manufacturing, and delivering three advanced green hydrogen storage tanks to the Klaipėda port for €1.4 million. These include a 40-bar green hydrogen buffer tank and two hydrogen storage tanks. "This project, which we undertook with a clear vision for the future, is not only an important step in the development of Klaipėda Port's infrastructure, but also a significant investment in long-term sustainable solutions that will shape a more modern, cleaner and innovative transport and industrial ecosystem’’, said Algis Latakas, general director of Klaipeda State Seaport Authority.
Source: Baltic Times, 13 January 2025.
technical development of decarbonization projects in the US, including Hybrit,’ it is no longer negotiating with the Department of Energy’s Office of Clean Energy Demonstrations to complete its funding agreement, the company said in a statement to Canary Media. Source: Fuel Cell Works, 14 January 2025.
Brazilian mining company Vale and the Jubail and Yanbu Royal Commissions have signed an agreement to reserve land for a green steel
megahub in the industrial city of Ras Al Khair in Saudi Arabia. The project will be implemented in two stages. It has the potential to produce
ArcelorMittal Nippon Steel India, a joint venture between ArcelorMittal and Nippon Steel, has said that it is set to commission two production lines at its Gujarat-based facility this year to produce advanced automotive steel products. The company said that once operational, the production of the two units will substitute imports of high-end steel required by the entire automotive sector, promoting 'Atmanirbhar Bharat', a slogan coined by the current government of India, meaning ‘self-reliance’.
Source: The Economic Times, 19 January 2025.
ArcelorMittal Duisburg will stop purchasing pig iron from Thyssenkrupp Steel due to environmental concerns, Kallanish has reported. ArcelorMittal has decided not to extend the current contract, which runs until September 2027. The company cited high carbon costs associated with the supply. This is another negative factor in addition to the challenging market conditions for wire rod, the main product produced by the plant. ArcelorMittal is currently testing a number of alternative options after the contract expires.
Source: GMK Center, 20 January 2025.
ArcelorMittal is pressing ahead with the commissioning of its hydrogen furnaces in Kraków, despite pausing any financial decisions on green hydrogen-ready direct reduced iron plants last November. The project represents an investment worth $12.6m. However, the world’s secondlargest steelmaker previously said it would delay final investment decisions on decarbonization projects after the European policy, energy and market landscape had not moved in a ‘favourable direction.’
Source: H2 View, 21 January 2025.
Building and engineering company Sir Robert McAlpine has been appointed as main works contractor for Tata Steel’s £1.25 billion green steel plant in Port Talbot. Sir Robert McAlpine will manage
up to 12Mt of direct reduced iron per year. The hub will serve local, regional and international markets and will contribute to the realization
Anglo American is partnering with the University of Birmingham and venturebuilder Cambridge Future Tech to launch PeroCycle, a new venture aimed at developing and commercializing carbon recycling technology for implementation in steelmaking. PeroCycle will build upon innovations made at the University of Birmingham’s School of Chemical Engineering by Professor Yulong Ding and Dr Harriet Kildahl, who pioneered an in-process carbon recycling method with the use of a double perovskite material. The use of the material enables the in-process splitting of carbon dioxide into carbon monoxide at considerably lower temperatures than current methods.
Source: Green Car Congress, 24 January 2025.
the main, civil, structural, and building works for a new electric arc furnace-based steel production facility. The facility will produce around 3Mt/yr. The project is set to be completed in three years and
of Saudi Arabia’s industrial ambitions in line with its Vision 2030 programme.
Source: GMK Center, 17 January 2025.
will involve the construction of a new electric arc furnace, ladle furnaces and associated works.
Source: Manufacturing Management, 29 January 2025.
Czech steelmaker Liberty Ostrava, a subsidiary of British Liberty Steel, which is up for sale, has resumed production at its hot strip mill after more than a year’s break. “This is another important milestone for us. The production of flat products such as coils, sheets, and steel strips, i.e. traditional steel mill products, which we are now reintroducing to the market, is returning to Liberty’s portfolio,” said Tomáš Mishinger, director of machinery trade.
Source: GMK Center, 29 January 2025.
‘DANIELI’ 600,000 TPY LIGHT & MEDIUM FLEXIBLE SECTION ROLLING MILL (2011)
120TPH Furnace, Breakdown Mill, Ultra-Flexible Reversing Mill, Continuous Finishing Mill, Automatic Stacker for Beams at Stahl-Gerlafingen, Switzerland
‘NKK-SMS’ COMPACT STRIP PRODUCTION PLANT (1995/2011)
150T Heat Size Electric Meltshop, Thin Slab Cast & Rolling, Thick Slab Caster & (2014) Skin Pass Mills at Hyundai Steel Dangjin, South Korea.
‘HAEUSLER’ 60,000 TPY LSAW WELDED PIPE PRODUCTION LINE (1991/2014)
Plate Edge Milling, 3-Roll Bending, Inside & Outside Welding, Copander, Testing Equipment and more at Hyundai RB Ulsan, South Korea.
‘CONCAST-DAVY-UNITED-FATA’ SLAB CASTER, HOT STRIP MILL & COLD ROLLING COMPLEX (1990s)
Slab Caster, Hot Strip Mill, Tandem Cold Mill, Annealing & Coating Lines at CAP Acero Huachipato, Chile.
Strategic Resources, a mineral development company, has announced a collaboration agreement with Tacora Resources to explore using Tacora’s iron ore concentrate for Strategic’s planned highpurity iron pelletizer project at Port Saguenay. The agreement allows Strategic to potentially purchase up to 25% of Tacora’s expanded throughput capacity, which could provide 25% to 40% of the required iron ore concentrate feed for the project. This collaboration aims to enhance the development of clean, green steel inputs in Canada and support the transition to a low-carbon economy.
Source: Tip Ranks, 29 January 2025.
ArcelorMittal and LanzaTech Global have announced that ethanol from the former’s Steelanol facility in Ghent reached a production milestone with the first barge load having been shipped. Ethanol produced can be sold straight into fuel markets or purified and transformed for use in a variety of consumer products such as garments, personal care, and packaging.
ArcelorMittal South Africa has shut down its steelmaking operations in Newcastle and Vereeniging, despite earlier
Chinese-owned steel manufacturer Disco Zimbabwe has announced the production of its first reinforced steel bars (rebars), marking a ‘significant milestone’ in Zimbabwe’s industrial sector. The rebars, essential for construction projects, are
JFE Steel and JSW Steel have completed the acquisition of Thyssenkrupp Electrical Steel India through their joint venture JSW JFE Electrical Steel. The purchase price is estimated at $457 million. The company, which was owned by Thyssenkrupp, will focus on the production of granular electrical steel (GOES). This
This achievement advances LanzaTech and ArcelorMittal's collaborative plan to create a robust European supply chain for regionally produced sustainable ethanol. Ethanol production began in 2023, and the facility is the first of its kind in the European steel sector.
Source: MSN, 30 January 2025.
reports that the government was considering a rescue package for the company.
In a 10-page letter to trade unions, including the National Union of Metalworkers of South Africa and Solidarity, ArcelorMittal said it had no plans to reverse its shutdown decision, which resulted in 3,500 direct and indirect job losses. Source: Daily Maverick, 1 February 2025.
being manufactured locally as part of the company’s efforts to support Zimbabwe’s infrastructure development and reduce reliance on imports.
Source: The Zimbabwe Mail, 3 February 2025.
will allow the joint venture to enter the Indian market for these products ahead of schedule. JFE Steel and JSW Steel aim to meet India’s growing medium- and long-term demand for GOES while creating an integrated production and sales system.
Source: GMK Center, 5 February 2025.
London’s High Court has ruled that a company in Sanjeev Gupta’s metals empire owes $53 million to a joint venture between ArcelorMittal and Nippon Steel. This judgment came after Gupta, who is battling many legal claims from creditors and a criminal prosecution for failing to file accounts on time, told the court his company was unable to participate in a trial held last November due to an inability to continue to pay legal fees.
Source: The Financial Times, 30 January 2025.
British industry minister
Sarah Jones has hosted a roundtable to finalise the UK government’s upcoming Steel Strategy at Sheffield Forgemasters. Following on from the inaugural meeting of the UK’s new Steel Council in January, the roundtable of more than 30 CEOs and industry leaders focused on steel demand over the next five- and 10-year terms, and is one in a sequence of followup events.
Source: The Manufacturer, 31 January 2025.
voestalpine Tubulars in Austria has successfully commissioned the profile rolling mill engineered by Friedrich KOCKS GmbH & CO KG. Following the completion of all work and successful commissioning, the new equipment is now fully integrated into the hot tube rolling mill at the Kindberg site in Styria.
The award of the contract was preceded by an intensive technological dialogue on the
design and construction of a solution for rolling seamless hollow sections. The centerpiece of the profile rolling mill, called PWW 530/4, consists of four consecutive 530 mm stands, each equipped with two horizontal and vertical rolls facing each other. These four rolls in the stands are individually driven. Based on KOCKS’ 3-roll stand design, they feature caliber adjustment and aim to allow for fast roll changes, providing flexibility
in production planning. The scope of supply also includes the corresponding equipment for the roll shop.
voestalpine Tubulars is now able to produce hot-rolled square and rectangular hollow sections up to large formats and wall thicknesses.
For further information, log on to www.kocks.de
AMETEK Land, a manufacturer of monitors and analysers for industrial non-contact temperature measurement, has released a new video demonstrating its technology live within a UK steel plant.
Filmed at Marcegaglia UK’s Sheffield steelworks, it provides insights for customers wanting to see how LAND’s products solve challenges in industrial environments.
The video is narrated by Marcegaglia UK’s Phil Smales, who guides viewers through the stainless-steel melt shop, explaining how the mill operates, and showing where the LAND solutions are used in the process.
James Cross, AMETEK Land’s head of industry management, commented: “I feel like we’re part of the digital ecosystem that helps with the operation and efficiency of the Marcegaglia UK plant, and we’re proud to be a part of that. We’ve developed a great partnership with the team, and I’m delighted that, with this new video, customers can see for themselves the difference that we’re making. It’s the first video of this kind that we have produced, and one we believe will help industrial plant operators understand how we can forge a similar collaboration with them, meeting their own unique steel production challenges.”
Phil Smales added: “The main challenges are keeping tight control on temperatures and the materials. The LAND team look at our processes and comes up with ideas or equipment that could enhance our processes in our plant for future operations.”
Part of Marcegaglia, a global industrial group, Marcegaglia UK is the largest precision tube manufacturer in Great Britain, delivering carbon steel product solutions worldwide.
For further information, log on to ametek-land.com/industries/steel
Fives has partnered with a European tube manufacturer to develop a new OTO flying plasma cut-off system. The advanced technology can cut a wide range of tubes with different thicknesses.
The OTO plasma cut-off is a solution that uses a high-temperature jet of ionized gas to melt and remove material along the cutting path, aiming to create a smooth and precise surface. Compared to conventional saw or blade cutting, the plasma solution enables cuts on a wide range of tubes (CHS, RHS, SHS, SPECIAL). It significantly reduces mechanical stress and equipment wear and eliminates the need for blade replacement, says Fives.
Fives is currently testing both a traditional and an automated system for its OTO tube mills. The automation technology works with robots, making it particularly suitable for high-capacity production and complex cuts. The robots can perform curved, angled, and 45-degree cuts, even on irregular tubes. This allows maximum flexibility to adapt to different production formats, which minimizes operator intervention.
The solution will soon be available on the market to meet customer needs.
For further information, log on to www.fives.com
Experience the future of plant operation in the metals industry with integrated solutions featuring cutting-edge sensor and control technology, AI-fueled processes, and predictive maintenance. Manage entire plant complexes from centralized operator stations, on site, or even off site, while benefitting from minimal integration efforts. Concentrate fully on your core operations and run your plants at a previously unimaginable level
A Chinese steel producer located in the northeastern part of China has placed an order with Primetals Technologies for a new 6-strand bloom casting machine. The equipment will be implemented later this year.
Primetals Technologies will supply the complete mechanical equipment along with full Level 1 and Level 2 automation systems.
The caster will enable the production of high-quality blooms for export markets, focusing on premium rails and wire rod, including steel
cord used for tire reinforcement. It will feature the largest curved copper tube mold ever implemented by Primetals Technologies. The DynaFlex hydraulic oscillator, which the company claims allows for flexible adjustment of the mold-oscillation parameters, is another key mechanical feature.
The fully automated roll-gap control system DynaGap Soft Reduction aims to minimize centerline segregation, enhancing internal strand quality. The bloom caster will also be equipped
with Dynacs 3D, a secondary-cooling model that calculates the full 3D temperature profile along the entire strand, facilitating optimal adjustments of secondary-cooling setpoints. Mold level stability will be controlled by the automatic LevCon control system, which includes autostart casting functions and auto-adaptive dynamic bulging compensation.
For further information, log on to www.primetals.com
As part of a strategic partnership with TVARIT GmbH, a specialist in AI solutions, PSI is integrating TVARIT’s metals-specific hybrid AI models into the PSImetals Service Platform. This will enable AI-driven solutions to be provided more quickly in the future, improving and accelerating customers’ digitalization processes, says PSI.
TVARIT provides Hybrid AI Models specifically for the metals industry. PSI developed the digital twin technology, which knows and tracks production data in real time across the entire production management chain. These models will be integrated into the PSImetals Service Platform to further use the production data to enhance the decision-making process in specific production management processes. This, claims PSI, will optimize metals production processes including production, quality management and decarbonization services.
“With PSI as a strategic partner, TVARIT takes a significant step forward in driving AI-powered Industry 4.0 transformation within the metals industry. By bridging data silos, this collaboration enables seamless end-to-end process optimization. Together, we aim to unlock hidden profitability, achieve exceptional quality standards, and empower metals producers to enhance sustainability and competitiveness,” stated Vikas Goel, vice president of operations at TVARIT GmbH.
For further information, log on to www.psi.de
Swedish company GreenIron details the mechanisms that underpin its ambitious goal: decarbonizing iron and steel production.
THE iron and steel industry is undergoing a significant transformation. As global industries push towards net-zero emissions by 2050, the urgency to innovate has never been greater.
Traditional steelmaking, while foundational to economic development, remains one of the largest contributors to global carbon emissions, responsible for nearly 8%* of annual CO2 emissions [claims of the global steel industry’s annual CO2 emissions tend to vary from 7% to 11%]. This reality demands a shift not only in energy use but in the very processes at the heart of steel production.
At GreenIron, we are redefining the way iron is processed, providing a pathway towards a more sustainable and circular economy.
The challenge: decarbonizing iron and steel production
Direct Reduced Iron (DRI) presents a promising decarbonization pathway, offering a low-emission alternative to traditional blast furnaces. Yet, many existing DRI facilities still rely on natural gas, limiting their climate benefits. The transition
to hydrogen-based DRI, though still early, is rapidly gaining traction as the key to carbon-neutral steelmaking – and GreenIron is at the forefront with a flexible, hydrogen-ready solution that scales sustainably.
The GreenIron process: a game-changer for CO2-free iron production
GreenIron’s innovative process tackles the challenges of decarbonising iron production by enabling fossil-free iron reduction using 100% renewable energy and hydrogen. Unlike traditional DRI methods that rely on large-scale infrastructure and continuous gas supply, GreenIron’s solution is both flexible and scalable – designed to adapt to real-world demands while supporting sustainable production.
Key features of the GreenIron process:
� Feedstock flexibility: the process can handle various materials, including iron ore fines, pellets, and metal by-products such as mill scale and slags. This adaptability bridges the gap between virgin iron production and recycling, making it a
versatile solution across different industries.
� Modular and scalable design: our modular system design allows production to be tailored to local conditions and individual customer needs, avoiding high costs and hence risk associated with large-scale, capital-intensive infrastructure.
Modular expansion for scalable growth
GreenIron’s modular design allows a prosite
and provides a cost-effective, demand-driven pro-
GreenIron’s modular design allows a production site to start with a single GreenIron furnace and gradually increase capacity by adding more furnaces as demand grows. This decreases the need for large initial investments and provides a cost-effective, demand-driven production set-up, reducing financial risk.
Flexible batch process for maximum adaptability
With GreenIron’s batch process, the type of materials being processed can change from
hour to hour, providing flexibility in operations. In addition, the furnaces can be easily started and stopped in response to energy availability, enabling optimal energy use and mitigating risks during periods of fluctuating power supply.
The outcome: a CO2-free reduction process that supports circular value chains and empowers steelmakers to secure sustainable iron inputs for their operations – without compromising flexibility or growth potential.
The GreenIron process is a catalyst for change across the value chain. Below are some of the key applications and advantages of this technology:
� Production of fossil-free iron for green steel: GreenIron’s process supplies the iron units essential for carbon-neutral steel production, making it ideal for both greenfield projects and retrofits.
� Utilisation of by-products: by enabling the reduction of industrial waste and by-products like mill scales, filter dusts, and slags, we contribute to closing material loops and enhancing
resource efficiency.
atives:
Support for circular economy initiby reducing reliance on virgin raw materials and making use of residues, the GreenIron process aligns perfectly with the circular econo-
my’s objectives.
The role of partnerships in scaling GreenIron’s solu-
tion
partnerships are central
energy suppliers, recycling
A technology’s true impact lies in its adoption, which is why partnerships are central to GreenIron’s growth strategy. By collaborating with iron ore producers, energy suppliers, recycling companies, foundries and steelmakers, we create ecosystems that enable sustainable operations.
Our recent Memorandum of Understanding (MOU) with Vale, one of the world’s largest mining companies, exemplifies this approach. Together, we’re working to establish a direct reduction facility in Brazil and expand operations in Sweden, where Vale supplies iron ore agglomerates to our commercial facility in Sandviken.
this approach. Together, we’re
This partnership highlights how strategic collaboration can accelerate decarbonization across the metals supply chain, ensuring access to critical inputs such as hydrogen and renewable power – essential for supporting largescale green steel initiatives.
This partnership highlights how decarbonization across the metals scale green steel initiatives.
A Reducing & Sizing Block for long products keeping its promises. Achieve your goals with KOCKS RSB®
finishing size in round or hexagonal dimensions up to 160mm
increase in production up to 20%
up to 10% energy savings in the mill line
The broader industry transformation: where are we headed?
The GreenIron process is part of a larger wave of innovation transforming the steel industry. The transition from coal-based to hydrogen-based ironmaking represents one of the most significant shifts in the sector’s history.
Key trends driving this change include:
� Green hydrogen investments: Global regions are rapidly scaling up hydrogen infrastructure.
� Carbon Border Adjustment Mechanisms (CBAMs): EU regulations penalise imports with high carbon footprints.
� Focus on Scope 3 emissions: Producers must address emissions throughout their supply chains.
Challenges ahead and the path forward
Despite these advances, the road to zero-carbon iron and steel is not without its challenges. The cost and availability of green hydrogen, regulatory uncertainty, and infrastructure requirements remain barriers to rapid adoption.
To support the development of green industrial value chains, policies must address external dependencies that impact companies’ transfor-
mation efforts. Key focus areas include:
� Permitting processes: streamline and make them more predictable through clear guidelines and stronger collaboration between stakeholders.
� Climate-focused procurement: implement standardised climate criteria to drive demand for low-carbon materials.
� Risk sharing for local investments: national mechanisms should support municipalities making industrial-related investments.
� Aligned targets: all actors must work towards shared goals that consider environmental and socio-economic impacts.
� EU climate policy: uphold commitments under Fit for 55 and the EU Emissions Trading System (ETS).
� Local value sharing: ensure communities benefit from renewable energy projects to foster acceptance.
The transformation of the iron and steel industry will require collaboration across all sectors, from governments, communities to private enterprises.
The vision will become reality With the right policies, partnerships, and techno-
logical advancements, the vision of a sustainable and circular steel industry can become a reality. By addressing these key areas, we can create a policy environment that accelerates the green transition while ensuring socio-economic sustainability.
Companies that prioritise innovation, like GreenIron, are proving that it’s possible to overcome hurdles – particularly by offering modular and flexible solutions that lower risk.
At GreenIron, we believe the time for change is now. By offering a modular, flexible, and CO2-free solution for iron reduction, we’re helping lead the way toward a sustainable future. Through innovation and collaboration, we’ll remain at the forefront of this industry-wide transformation.
In a world where decarbonizing the steel industry is top-of-mind for all steelmakers, the journey to global fossil-free steelmaking is both ambitious and necessary; and with a clear vision, strong partnerships, and the right tools, a climate-neutral metals and mining industry by 2050 is well within reach. �
Performances, operational reliability and quick startups are the result of 20 years of continuous research and development activities, carried out at the Danieli research center and onsite together with partnering customers. Depending on plant configuration, MIDA QLP can make use of more than 30 Danieli patents covering technological layouts, production equipment and Danieli Automation solutions, such as power, instrumentation and intelligent digital controls.
— The most efficient, digitally controlled electric steelmaking with no impact on the power grid.
— 10 m/min casting speed, allowing up to 1.5-Mtpy productivity on one casting strand, 23.5 hours out of 24 of continuous endless-casting operation.
— No gas-reheating furnace, and no induction heating during casting.
— Danieli Automation robotics and artificial intelligence for zero-men on the floor.
— Least power-consuming process with the lowest carbon footprint.
— The most competitive plant in terms of CapEx and OpEx.
With the order placed by CMC Steel for its fourth new, MIDA QLP hybrid-ready minimill, the Danieli scorecard hits 26 plants for long-product endless casting-rolling, out of 115 total minimills.
PLANTS
The outrage over former President Joe Biden’s announcement on 3 January to block the proposed US Steel/ Nippon merger has escalated with Nippon Steel saying it was taking legal action against the US government.
By Manik Mehta*
BIDEN explained his decision to block the controversial $14.3 billion acquisition of US Steel was to protect national security interests and supply chains.
The merger deal, announced more than a year ago, was controversial from the start, with both political parties expressing their opposition to the control levers of a company, once considered an icon of the US industry, falling into the hands of a foreign company.
The United Steelworkers (USW), the steelworkers’ union, which had also opposed the merger deal, applauded Biden’s decision as the ‘right move for our members and our national security’.
David Burritt, the president/CEO of
US Steel, who had campaigned for the merger with Nippon Steel, criticized Biden’s decision as ‘shameful and corrupt’. The Committee for Foreign Investment in the United States (CFIUS), which reviewed the merger deal and assessed its risks to national security, failed to reach a consensus on whether the deal would pose a national security risk and left it to the President to decide.
Both the merger partners had argued, while the deal was being reviewed by the CFIUS, that the merger was necessary to provide the investment needed for US Steel’s domestic steel operations, with the latter warning that it could be forced to shut down the mills represented by USW,
*US Correspondent, Steel Times International
if it did not get the $2.7 billion investment planned by Nippon Steel as part of its proposed purchase. US Steel also warned that the deal’s rejection could frighten away foreign investment in other US industries.
The two companies had said in a joint statement that they were confident that their merger would revitalize communities that rely on American steel, including in Pennsylvania and Indiana, provide job security for the American steelworkers, enhance the American steel supply chain, help America’s domestic steel industry compete more effectively with China and bolster national security.
In their 6 January lawsuit, both claimed that Biden’s executive order to
filed a separate lawsuit against Lourenco Goncalves, the CEO of rival steelmaker Cleveland-Cliffs, and Dave McCall, the president of the United Steelworkers (USW) union, for their actions to try to block the deal which, the lawsuit alleged, are ‘anticompetitive and racketeering activities illegally designed to prevent any part other than Cliffs from acquiring US Steel as part of an illegal campaign to monopolize critical domestic steel markets’. The lawsuit, which is aimed to stop Cliffs and USW from acting together, also seeks
could discourage the much-needed foreign investment in other US industries, and that the ultimate result will be a setback to US Steel’s future competitiveness. One expert, familiar with the internal dissonance within the Biden administration over blocking the merger deal, described the decision as ‘bad’, saying that it would not actually protect union jobs and could do great harm to the company.
US Steel, which needs an infusion of fresh investment to upgrade its facilities, had pinned its hope on the acquisition
block the merger was issued for ‘purely political reasons’. In a statement, the two companies described their legal actions as a continuation of their ‘commitment to completing the transaction – despite political interference’. The lawsuit comes as no surprise, with both companies calling Biden’s order ‘a clear violation of due process’ and seeking appropriate action to protect ‘our legal rights’. Biden, they said, had ‘ignored the rule of law to gain favour with [the United Steelworkers union] and support his political agenda’. However, the deal was also opposed by President Donald Trump and Vice President J.D. Vance had also opposed the deal, with Trump saying that he would block it if it was not dead by the time he assumed office. There is bi-partisan belief that US Steel, once a prized jewel of the American steel industry, should not fall into foreign hands.
Besides the lawsuit initiated against the US government, the two companies
‘substantial monetary damages for their conduct’.
But USW President McCall again praised Biden’s action and denounced the lawsuit.
To recall, US Steel had stated in August 2023 that it had received several offers for purchasing the company, including one $7.3 billion offer from ClevelandCliffs which had overtaken US Steel to become the second biggest American steelmaker behind Nucor; however, talks with Cleveland-Cliffs had broken down. Cleveland-Cliffs’ offer, by comparison, was about half of Nippon Steel’s offer of $14.7 billion.
The USW, on its part, opposed the deal because it felt that Nippon had not given it sufficient guarantees protecting unionized jobs at some of the company’s older mills staffed by union members, and urged US Steel to continue to ‘support good jobs, healthy communities and robust national and economic security well into the future,’ the union said in a statement.
Some experts fear that Biden’s action
by Nippon Steel; the former is also aware that even if another rival like Nucor Corp. or Cleveland-Cliffs expressed interest in acquiring part or all of US Steel, antitrust concerns could prevent such deals which could give a strong monopolistic position to that buyer.
Meanwhile, Cleveland-Cliffs, which had unsuccessfully tried to buy out US Steel, issued a statement with Lourenco Goncalves, chairman/president/CEO, saying that Nippon Steel-US Steel continued to play the ‘blame game’ in a desperate attempt to distract from their own failures. He called the lawsuits a ‘shameless effort to scapegoat [sic] others for US Steel’s and Nippon Steel’s self-inflicted disaster’.
“Cleveland-Cliffs and the USW were not the only ones who recognized the adverse national security implications of this acquisition. This deal drew instant bi-partisan opposition, including from President Trump, who vowed multiple times that he would block the deal,” the Cleveland-Cliffs statement said. �
Benefits:
Utilizes cutting-edge optics and laser scan technology providing an exceptionally high sampling rate
Practically unrivalled measuring accuracy
Reliable measurements in even the harshest of environments (oil, dust, steam etc.)
Easy to handle and is almost maintenance free
Big installation flexibility of 1, 2 and 3 axis gauges for products as small as ø 0.012 mm – 520 mm
Having led Argentina for just over a year, Javier Gerardo Milei has set in place a number of controversial economic policies which have had a sizeable impact on the domestic steel industry.
By Germano Mendes de Paula*
JAVIER Gerardo Milei is an economist, politician and professor, having served as Argentina’s 52nd president since 10 December 2023. As the leader of the Libertarian Party and the coalition La Libertad Avanza, he was elected with 55.65% of the votes in the second round, making him the president with the highest proportion of votes in the country’s history. Milei has been characterised in various ways, including right libertarian, farright, ultra-conservative, ultra-liberal, and right-wing populist. He identifies himself as an anarcho-capitalist, which essentially translates to advocating for minimal government. A year into his presidency, it is pertinent to examine the macroeconomic landscape and its impacts on the steel industry.
Milei’s electoral campaign highlighted the dollarisation of the economy and the closure of the Central Bank as pivotal
points. However, these measures have not been implemented. The country’s low dollar reserves and international scepticism complicate bond sales in US currency. Early in his administration, Milei enacted a 50% devaluation of the Argentinian peso as part of an inflation-reduction strategy. Following this initial devaluation, the government has adjusted the rate by 2% each month. When Milei promised to close the Central Bank, he communicated an intention to stop the currency issuance that contributed to the inflationary cycle.
Milei has executed significant fiscal adjustments. By November 2024, government spending decreased by 27% compared to 2023, when adjusted for inflation. The average amount of pensions and social security benefits dropped by 17%, with civil servant payrolls falling by 19%. Investment in public works plummeted by over 77%, and the public
workforce shrank by 36,000 positions from January to November 2024.
Argentina’s poverty rate rose to nearly 53% in H1 2024, according to official statistics. This marked a sharp increase from 41.7% in H2 2023 and more than double the rate of 26% seven years prior, illustrating the severe social consequences of Milei’s economic policies.
Upon Milei’s election in 2023, Argentina had one of the highest annual inflation rates in the world at 211%. Monthly prices were escalating by 13% and surged to 25.8% in December 2023, following a significant currency devaluation enacted by his administration. However, as of November 2024, monthly inflation has decreased to 2.4%, the lowest value since July 2020, even though it still accumulated to 166% y-o-y and 112% for 2024.
In 2024, the Argentinian stock market experienced a 172.5% increase in pesos,
* Professor in Economics, Federal University of Uberlândia, Brazil. E-mail: germano@ufu.br
leading gains among 20 global exchanges. When measured in dollars, the Merval index rose by 113.8% based on the exchange rate at the year’s end. This discrepancy is due to the dollar appreciating (in nominal terms) by 27% against the peso. Nevertheless, the gap between the official and parallel exchange rates has narrowed from 200% to less than 20%, a significant improvement attributed to Milei’s policies, which included greater flexibility for exporters to convert their revenues in the parallel market.
According to the OECD, Argentina’s GDP was projected to diminish by 3.8% in 2024, followed by growth of 3.6% in 2025 and 3.8% in 2026 (see Table 1 for additional macroeconomic data). Given the extensive macroeconomic shock, a GDP reduction of less than 4% in 2024 can be viewed as a relatively favourable outcome.
The steel industry performance
As reported by the Argentinian Chamber of Steel, cement shipments in the construction sector fell by 25% y-o-y from January to November 2024. The automotive industry also faced an 18% decline in production in the same period. Conversely, the agricultural machinery sector maintains
positive prospects for the wheat harvest, with preliminary results indicating higher yields than the previous cycle. Similarly, shipment levels to regions engaged in energy activities remain stable, with positive levels for 2025.
Graph 1 compares the production of steel inputs and products in Argentina from December 2023 to November 2024 with the same previous period. Notably, pig iron production decreased by 15.4%, while DRI output fell by 25.9%. Crude steel production dropped by 21.8%, with hot-rolled longs (including seamless tubes) contracting by 32.9%, hot-rolled flats by 22.9%, and cold-rolled flats by 23.5%.
In 2024, ArcelorMittal Acindar, the leader of long steel products, which was the most impacted market segment, temporarily halted operations at its Villa Constitución mill three times. The first interruption occurred from 18 March to 15 April due to a sales drop of approximately 35%-40%, reflecting the challenging conditions in the domestic economy. In June, the company announced a shutdown of its DRI unit, melt shop, and rolling mills, expecting all units to resume operations starting midJuly. Moreover, in December, it suspended 700 workers at the Villa Constitución mill, indicating a serious downturn in production, which would amount to only 600kt being produced in 2024, against 1.1Mt in 2023 and 1.2Mt in 2022.
By late October 2024, the Argentinian Chamber of Steel projected that the country’s steel shipments would decline by 26% y-o-y for 2024, followed by a 25% recovery in the subsequent year (Table 2). For apparent steel consumption, the anticipated figures are a 32% drop and a succeeding increase of 28%. However, even with strong growth, steel use in 2025 would be 13% lower than the level registered in 2023. Imports are expected to decrease by 42% in 2024, followed by an increase of 32% in 2025, while exports are forecasted to grow by 8% and then 13%. All data in this paragraph refers to rolled steel.
In conclusion, the impact of Milei’s macroeconomic shock has severely affected the steel industry. This, to a large extent, can be explained by a projected decline of 23.9% in gross fixed capital formation for 2024, according to OECD (Table 1). It is noteworthy that four of the five largest steel companies operating in Argentina –Ternium, Tenaris, ArcelorMittal Acindar, and Gerdau – also work internationally, while Acerbrag is part of the diversified Brazilian group Votorantim. Therefore, these enterprises do not rely solely on Argentina for their revenues and profits. While the situation in 2024 was challenging, there appears to be a sense of optimism that the worst may be over, with expectations that 2025 will bring significant improvements. �
India’s finished steel demand outpaced the growth rate in major steel-consuming countries in the calendar year 2024, driven by an increased governmental focus towards steel-intensive construction in the housing and infrastructure sectors, along with robust demand from engineering, packaging, and other segments. By Dilip
Kumar Jha*
CRISIL, an S&P Group credit rating agency, estimated India’s finished steel demand to have risen by 11% in 2024, marking the second consecutive year of double-digit growth after a 13.5% expansion recorded in the previous year.
This upward trajectory has been supported by strong finished steel production, which grew by 12.7% year-onyear to reach 139Mt in the financial year 2023-24. This positive trend is attributed to supportive government policies that have stimulated robust demand growth, as well as significant investments in expanding steel production capacity. India’s Steel Policy aims to achieve 300Mt of production capacity and 265Mt of demand by 2030.
Global steel demand is estimated to have registered a de-growth of approximately 1% in 2024. Demand in China, the largest steel producer and consumer, is projected to have fallen by about 3.5%, driven by reduced demand from the real estate sector, despite favourable policy changes and the release of support packages. Steel demand
Source: Crisil, a S&P Group credit rating agency
Source: Joint Plant Committee, India’s Union Ministry of Steel
in Europe, Japan, and the United States also showed a decline of 2–3%. However, demand growth in developing economies such as India and Brazil helped prevent a steeper global decline. Demand is estimated to have increased by 11% in India, 5.6% in Brazil, and 2.7% in other steel-consuming emerging economies.
Sehul Bhatt, director (research) at Crisil Market Intelligence and Analytics, stated, “In 2024, supply growth from India’s mills was moderate at 5.2%, due to extended periods of planned and maintenance shutdowns. Aggregate crude steel production by the top seven players increased by 0.05%, while finished steel
production rose by 0.5%. However, crude and finished steel production from medium and small players increased by 14% and 11.3%, respectively, underscoring the consistent demand growth from long steel end-users.”
Crude steel production grew from 109.14Mt in FY 2019-20 to 144.29 Mt in FY 2023-24, registering a robust growth of 13.4% over the previous year (127.197Mt in FY2022-23). The domestic steel industry’s capacity expanded from 142.29 in FY 2019-20 to 179.515Mt in FY 2023-24, supporting the production growth. Capacity utilization increased to 81% during the same period.
But India’s steel story isn’t merely about expansion in capacity, it is equally about rising domestic consumption. Total finished steel consumption grew from 100.17Mt in FY 2019-20 to 136.29Mt in FY 2023-24, indicating a strong domestic demand with a growth rate of 13.7% over the previous year. Industry projections, including a conservative 6% CAGR in steel demand
through FY 2026-27, suggest that India’s steel sector is poised to meet, and perhaps exceed, demand.
To boost steel production, a key initiative is the Production Linked Incentive (PLI) Scheme, aimed at attracting capital investments and reducing imports, with an anticipated INR295 billion investment and an additional capacity creation of 25Mt for specialty steel.
Growth trend for 2025
Domestic steel demand has been growing
at the fastest pace since FY 2021–22 in the post-global financial crisis era, largely driven by the central government’s capex initiatives. However, this growth hit a roadblock in the current fiscal year, as government capex declined by 12.3% yearon-year during April to November 2024. With private capex activity expected to remain subdued due to subpar economic growth, the outlook for steel demand growth hinges on a significant recovery in government spending. Unless government capex sees a meaningful rebound in FY 2025–26 (April-March), domestic steel demand growth may decelerate.
Steel demand in India is projected to continue outpacing other major steelconsuming economies with an estimated growth of 8–9% in the calendar year 2025. This growth will be driven by a shift toward steel-intensive construction in the housing and infrastructure sectors, along with stronger demand from engineering, packaging, and other segments.
Crisil estimates global steel demand in 2025 to increase by 0.5–1.5%, supported by easing financing conditions and pent-up demand in key steel-consuming economies, which will bolster manufacturing activities. Anticipated recoveries in residential construction in regions such as the European Union, the United States, and Korea, driven by improved financing conditions, will also contribute to this growth. India will remain a leader in demand growth among major economies.
The imposition of a safeguard duty, as proposed by the domestic steel industry, could have a positive bearing on steel prices in 2025. If implemented by the end of February, steel prices in 2025 are expected to be significantly higher than in 2024, with the impact being more pronounced in the first half of the year.
Domestic steel prices, however, have been under pressure due to the decline in global steel prices. Steel prices have a potential upside of 4% to 6%, contingent on the implementation of the safeguard duty. As mills ramp up production volumes from newly commissioned capacities, the resulting increase in supply may keep flat steel prices under pressure, although they are still expected to remain higher than the 2024 average. Intense competition among mills to capture market share could, however, limit price increases. �
Steel Times International is a leading publication serving the global steel industry and offering its readers a strong focus on all aspects of the production process embracing both basic oxygen and electric steelmaking.
Choose your subscription package...
8 print copies and a printed copy of the Steel Times International Directory
Digital copy of the magazine delivered to your inbox every month
Four special digital only issues per annum
Access to our digital archive of past issues and webinars
Weekly Steel Times International newsletter
Printed A1 annual wallplanner
PLUS access to all digital issues of Furnaces International
With a digital subscription you will receive all the benefits of the Print + Digital option, excluding print copies!
This article assesses the potential impact of a global green iron trade in terms of shifting energy demand between regions and in terms of cost savings by comparing three scenarios for a global near-zero GHG steel industry. By Süheyb Bilici, Georg Holtz, Ole Zelt, Alexander Jülich, Annika Tonjes1, Robin König, Stefan Kronshage, Andreas Meurer2, Zhenxi Li3, Hilton Trollip, Bryce McCall4, Saritha Sudharmma Vishwanathan5, and Stefan Lechtenböhmer6
IN 2021, direct emissions from the industry sector made up about 21% of global emissions. The steel industry alone is responsible for 7% of the global energyrelated CO2 emissions, including industrial process emissions. The primary production of crude steel is a high-temperature process that includes the reduction of iron ore to iron and the further processing of iron to crude steel. The current state of the art in primary crude steel production relies on the use of fossil fuels, both as a reducing agent and for direct energy use. However, several CO2 emission reduction options for the steel sector are emerging, including increased recycling and material efficiency, carbon capture and storage (CCS) and hydrogen-based direct reduction of iron ore. The latter process has been the subject of numerous project announcements, including first investment decisions by major steel companies in Europe. This technology requires (green) hydrogen to reduce iron ore to Direct Reduced Iron (DRI), with few to no associated CO2 emissions. It is anticipated that this process will play a pivotal role in the global steel transformation.
The necessity to produce green hydrogen at scale and affordably for the direct reduction of iron ore raises the question of where in the world DRI production with green hydrogen will be concentrated in the future. The currently dominant technology for iron reduction, the blast
furnace, produces hot liquid metal which is subsequently processed into steel on-site. In contrast to liquid hot metal
from the blast furnace, DRI produced in a shaft furnace exists in a solid state and can be transported at reasonable costs
1. Wuppertal Institute for Climate, Environment & Energy, Wuppertal, Germany
2. German Aerospace Center (DLR), Institute of Networked Energy Systems, Stuttgart, Germany
3. Environmental and Energy Systems Studies at Faculty of Engineering, Lund University, Lund, Sweden
4. Energy Systems Research Group. Chemical Engineering Department, University of Cape Town, Cape Town, South Africa
5. National Institute for Environmental Studies, Tsukuba, Ibaraki, Japan, and the Indian Institute of Management-Ahmedabad, Ahmedabad, Vastrapur, Gujarat, India
6 University of Kassel, Kassel, Germany
as Hot Briquetted Iron (HBI) over long distances. The transportability of HBI as a bulk commodity allows the spatial decoupling of the iron reduction step from the steelmaking step. This may contribute to the global steel transformation by introducing an additional spatial dimension. Rather than transitioning from coal-based blast furnaces to hydrogen-based shaft furnaces for DRI production at the same production site, steel companies may decide to invest in shaft furnaces in global ‘sweet spots’, which are rich in renewable energy and, in the best case, also possess significant iron ore resources. Subsequently, the DRI could be transported in the form of
potential of relocation of energy-intensive industrial processes due to differences in the costs of renewable electricity production and analyse the concept of green iron export and its economic feasibility for Australia and South Africa. Lopez et al examine various steel supply chain configurations for European countries and address green iron import options from Australia, South Africa and Mauritania. The international green iron trade is inspected by Agora Industry and the Wuppertal Institute and its implications for importers and exporters are addressed in terms of employment, production costs and value creation. There are also several
HBI from global sweet spots to steelmaking facilities around the globe. This approach could help to reduce the associated costs of hydrogen-based steel production for steel companies. Eventually, a global green iron trade could emerge in the future, resulting in a near-zero global steel industry that differs significantly from the current state of affairs in which no such relocations are considered.
Several studies have examined the
scenario studies and roadmaps that explore how different mitigation options could be applied in global pathways towards a net-zero steel industry, but without any consideration of a global green iron trade. Agora Industry and the Wuppertal Institute consider global green iron trade in their Global Green Iron scenario and discuss its implications for the global decarbonization pathway, but do not break this down to regional decarbonization pathways. To the
author’s knowledge, no published global scenario exists to date that considers the global green iron trade as an element of a regionally differentiated global steel decarbonization pathway. Hence, the potential magnitude of a global green iron trade, its potential impacts with regards to relocation of energy demand between regions as well as with regards to global costs of the steel transformation towards near-zero emissions have not yet been analysed. This study addresses the research gap. By comparing three scenarios – Domestic, Intermediate Trade and Max Trade – it assesses the potential of a global green iron trade and its impact on the global steel industry transformation to nearzero emissions by 2050.
Scenarios can serve various purposes, from prediction through exploration of potential futures to normative description of desirable futures. Long-term scenarios do not aim to predict, but rather serve to depict alternative futures – often constructed as back casting scenarios based on specific future targets – to assess their respective impacts and to foster debate. Three scenarios that contrast alternative pathways for the global steel industry are presented in this article and their respective impacts are assessed. All three scenarios are based on regional near-zero technology pathways from the literature which is combined to form a global picture with the ambition of describing transformation pathways for the global steel sector, which achieve near-zero emissions by 2050.
The technology pathways for the main steel producing regions are used as the key basis for the scenarios. They are collected from various sources, such as studies, articles, government reports and policies detailing steel decarbonization pathways. Roadmaps from stakeholders such as steel associations and governments have been preferred over scientific studies in the selection of regional technology pathways in order to address the regional differences and the strategic considerations of actors to the largest extent possible. From the pathways described in these sources, those which best match the ambition level of achieving near-zero emissions by 2050 were selected. Where no sufficiently ambitious pathway
could be found in the literature for a region, a technology pathway was generated based on available policy documents or press releases of steel companies. Technology pathways have been finalized by adjusting them based on current policy and our own expertise.
A core strategy to achieve near-zero emissions by 2050 would result in a more efficient use of steel compared to
today. To reflect this, regional studies that include material efficiency strategies in their pathways towards a near-zero steel industry in 2050 are selected, if available. Chen et al., Green Energy Strategy Institute et al., Material Economics and the United States Department of Energy provide regional near-zero technology roadmaps that match the above-mentioned selection criteria for
China, South Korea, the EU 27 and the United States, respectively. Technology pathways from these studies have been adopted without major adjustments. The selected regional decarbonization pathways follow different technology paths due to differences in fossil energy endowments, economic development levels and steel demand trends. The EU, the US and China are regions with stabilizing or even
decreasing (China) crude steel demand, which can increasingly be met by scrap recycling. Therefore, secondary steelmaking plays an increasing role in these regions. In contrast, India faces a significant increase in crude steel demand and features the most cost-effective crude steel (CS) production with DRI using domestic thermal coal. Due to the resulting slow increase in scrap availability in India, demand growth is satisfied by primary production, mainly from conventional processes until 2030 and from fossil-DRI routes between 2030 and 2040. After 2040 the latter will be transitioned to green hydrogen. With scrap availability being expected to remain at current levels, decarbonization of primary production is expected to be driven by H2-DRI in Japan and South Korea. Existing conventional production technologies are either retrofitted with CCS or shut down in these regions, according to the selected technology pathways. Fig 1
Deduction of technology pathways for the Intermediate Trade and Max Trade scenarios
The technology pathways of the Intermediate Trade and Max Trade scenarios are derived from the Domestic scenario and additional assumptions on the substitution of domestic H2-DRI production with green iron imports, while total steel production and the role of other routes remain unchanged. In the Max Trade scenario, economic efficiency of steel production is the key factor for the future development of green iron markets.
It is assumed that all HBI-importing regions – i.e. China, EU 27, India, Japan, South Korea, the United States and the rest of the world (RoW) – take advantage of cost-efficient DRI production in global sweet spots as soon as possible. Consequently, they will begin importing green iron in the late 2020s, creating an increasing demand in the emerging global green iron market. It is assumed that the global sweet spots (Australia, Brazil, South Africa) will only be able to meet half of the demand for green iron by 2030 due to the limited pace at which the renewable energy and hydrogen production capacity required for green iron production can be built. With imports meeting half of their green iron demand by 2030, the other half is produced domestically in HBI-importing regions. After 2030, it is assumed that the global sweet spots will be able to increase
supply of green iron to an extent that all additional green iron demand of HBIimporting regions can be met. Since there is no differentiation of green iron import strategies between regions, the emerging global green iron trade is assumed to equally expand to all HBI-importing regions with the same pace.
In the Intermediate Trade scenario, it is assumed that a global green trade will initially (until 2030) include only those HBI-importing regions that have high domestic hydrogen costs, limited renewable energy potential in the proximity of existing steel plants and thus great benefits from importing green iron – i.e. the EU 27, Japan and South Korea. These regions are thus assumed to follow the same import strategy as in the Max Trade scenario. But, the Intermediate Trade scenario differs from the former scenario with regards to the green iron import strategies of HBI-importing regions like China, India, the United States and the rest of the world. They are assumed to focus on expanding their domestic capacity for green iron production for reasons of self-sufficiency and strategic independence, in particular before 2030.
With the EU 27, Japan and South Korea being the only green iron importing regions with growing but limited green iron demand until 2030, it is assumed that green iron trade will initially develop through bilateral agreements between importing and exporting regions. After 2030, it is assumed that these bilateral agreements will give rise to a global green iron market, whose increasing liquidity will facilitate price reductions. Despite continuous ambitions for self-sufficiency in China, India, the United States and the rest of th world, it is assumed that the emerging global green iron market will attract half of the additional demand for green iron in these regions, while the other half will be met by domestic production through further capacity expansion and use of domestic renewable energy. The global import volume of green iron is calculated from regional technology pathways in the WISEE EDM Global Steel Model (GSM) – visit https://www. i2am-paris.eu/detailed_model_doc/wiseeedm for more information – and this is assumed to define the amount of green iron, which is globally traded. In the next
step, the GSM distributes the global green iron trade volume to the HBI-exporting regions – Australia, Brazil and South Africa – according to their market shares in global iron ore trade in 2020.
There are several studies that analyse the decarbonization pathways of the iron and steel industry in a global context, such as Agora Industry and Wuppertal Institute Bataille et al., IEA Net Zero Steel Initiative, Yu et al. In terms of global CS production volumes, our scenarios, together with IEA and Yu et al., show the lowest value for 2050 (1987Mt CS) among the compared scenarios, rooted in optimistic assumptions concerning material efficiency. In contrast, global CS production increases to 254Mt/yr, 2200Mt/yr and 2150Mt/yr in Net Zero Steel Initiative, Bataille et al. and Agora Industry and Wuppertal Institute, respectively. A higher level of CS production makes a deeper structural shift in the technology mix necessary, in order to achieve near-zero emissions by 2050. As a consequence, the level of DRI production would be higher when global CS production is assumed to be higher. This implies that the potential scale of global green iron trade may be greater than addressed in this study.
Another notable difference is the reduction in annual CO2 emissions by 2030 between the scenarios. Emission reduction shares vary from 17% to 47% between the scenarios examined, with the Tech Moratorium scenario in the Net Zero Steel Initiative and our scenario study. Figs 2, 3,4 and 5
A potential challenge implied by the trade scenarios is that the renewable electricity required to produce H2-DRI for a large share of the global steel industry must be generated in a few exporting countries only – Australia, Brazil and South Africa, according to the scenarios. This implies the need for a very rapid scale-up of renewable electricity production in these countries. Producing 165-290Mt of green iron for export in Australia in 2050 would require 575-1025 TWh of green electricity only for this purpose, which is 2.1-3.8 times today’s electricity consumption in the country. Similarly, producing 65-115Mt of green iron in Brazil and 12-22Mt in South Africa would require 230-410 TWh green
electricity in Brazil and 45-80 TWh in South Africa, which amounts to 40-72% and 2035% of today’s electricity consumption in these countries, respectively.
These three countries possess a significant potential for renewable electricity production. In Australia, only 3-19% of the on- and offshore area suitable for green electricity production would be required to produce sufficient amounts of green electricity for DRI production according to the scenario (own elaboration based on COAG and Feitz et al). In the case of Brazil, the estimated potential for offshore wind alone exceeds the total electricity demand by more than 150% with currently feasible technologies. But the main challenge is to tap this potential in a comparably short time frame, considering that electricity demand for green iron exports comes on top of the renewable electricity required to replace fossils in the power sectors. In particular countries such as Brazil and South Africa, whose domestic electricity demands are expected to grow significantly until 2050 (200% - 480% in Brazil, 120% in South Africa), face the challenge to ramp up renewables fast enough to cover additional demand and phase out fossil fuelled power generation at the same time. In this context and from a systemic
perspective, green iron exports and their additional electricity demand only provide GHG mitigation benefits if it is ensured that this does not (directly or indirectly) extend the use of fossil fuels in power generation. Scaling up renewables at a rapid pace while avoiding unintended effects on fossil-based power generation brings up challenges for these countries.
Australia does not have a continentwide electricity grid and hence a subnational perspective is appropriate to avoid escalating infrastructure costs. As a consequence, the scale-up of renewables for green iron export would be mostly decoupled from dynamics in the domestic electricity system – except capital and engineering capacities required. Iron ore mines are concentrated in the northwestern part of Australia and hence a focused and massive ramp-up of renewable electricity and electrolyser capacity would be required in that area. In Brazil, the region Minas Gerais possesses 87% of steel production and 54% of iron ore production. In addition, protected areas of the Amazon, the Pantanal and the indigenous population are not located in this region. The region is well connected to the national electricity grid – hence the scale-up of renewables for green iron export needs to be
integrated into an overall electricity system transformation in order to avoid increased fossil-based electricity production.
The planned renewable electricity production in Brazil for 2050, about 2500 TWh according to EPE, would be sufficient to cover the expected domestic demand, 810-2100 TWh according to EPE, but could be exceeded if the additional electricity demand for green iron exports comes on top. Hence, the scenario might imply the need to ramp up renewables faster than currently planned.
Considering the investments in renewable electricity generation, transmission lines, hydrogen storage and DR plants, green iron production is a capital cost-intensive investment. Although the global ‘sweet spots’ have favourable conditions for renewable electricity and iron ore reserves, high financial risks could discourage investments for H2-DRI production in some of these regions. Cost calculations for South Africa as an example shows that a weighted average cost of capital (WACC) higher than 25% in South Africa would make the investments for hydrogen production unviable from a purely economic perspective, as the levelized cost of hydrogen (LCOH) in South Africa would then be higher than the estimated
hydrogen import costs in potential HBIimporting regions in 2030, as illustrated in Fig. 6. This signifies that very high financial risks in global ‘sweet spots’ could economically favour domestic production of DRI in today’s major steel producing regions based on iron ore and hydrogen imports.
Concerning major potential HBIimporting regions such as China, India and the EU, dependencies on single or few HBI-exporting countries for a strategically important industry such as the steel industry might be of importance, besides cost considerations. China has a high ambition on guaranteeing energy security and increasing self-sufficiency. Although, in 2020, China imported 82% of its iron ore consumption, it also produced 270Mt of raw iron ore domestically. With steel production set to decrease, China’s iron ore demand will decrease to 170-280Mt in the trade scenarios. This opens up possibilities to increase the share of domestic iron ore in steel production through maintaining the absolute level of domestic iron ore production while reducing imports. However, China needs to import only 40115Mt of DRI in the trade scenarios – a level that might be acceptable from a selfsufficiency perspective.
India’s steel consumption will increase significantly up to 2050, according to our scenarios. Today, India is a net exporter of iron ore and it has abundant iron ore reserves to cover its future demand domestically, if mining activities can be scaled up at a sufficient pace. India also features low-cost solar potential and thus, in principle, could even become a green iron exporter in the long term. Like other growing countries such as Brazil and South Africa, the main challenge is to scale up green electricity capacities sufficiently fast to decarbonize a growing electricity demand while producing green hydrogen for the steel industry simultaneously. Green iron import, as envisaged in the trade scenarios, could provide some relief to this challenge, but would come with cutbacks to self-sufficiency, which would counter current strategies targeting strong domestic production costs.
The magnitude of green iron imports varies across scenarios, as shown in Fig. 6 The volume of green iron imports into the EU 27, Japan and South Korea reaches 95 Mt/yr by 2050 and is assumed to take place equally in both trade scenarios. The global
green iron trade volume is contingent upon the assumptions made regarding the import volumes of other HBI-importing regions. In the Intermediate Trade and Max Trade scenarios, the global green iron trade volume reaches 240Mt/yr and 430Mt/yr, respectively, by 2050. In relation to global green iron production, which is 590Mt/yr in 2050 in both scenarios, 39% and 73% of green iron production is traded in 2050 in the Intermediate Trade and Max Trade scenarios, respectively. The export volume of iron ore of HBI-exporting regions decreases in both trade scenarios. In 2020, Australia, Brazil and South Africa exported 873Mt/yr, 343Mt/yr, and 66Mt/ yr of iron ore, respectively. Assuming a constant rate of iron ore production until 2050, 26% and 47% of iron ore production is used to produce and export green iron in the Intermediate Trade and Max Trade scenarios, respectively (Fig. 6). These results demonstrate that the quantity of iron ore processed to HBI in the HBI-exporting regions in the trade scenarios is significantly below their current iron ore export volume. The current iron ore mining capacities hence do not constrain the potential of global green iron trade to reach a trade volume of 240-430Mt/yr in 2050, as presented in this study.
In summary, the global green iron trade starts in the late 2020s and grows
significantly until 2050, resulting in a global trade volume of 10-25Mt/yr in 2030, 125-200Mt/yr in 2040 and 240-430Mt/ yr in 2050 in the trade scenarios. In the Max Trade scenario, China, India and RoW are the regions with the largest annual HBI imports in 2050, accounting for more than 100Mt/yr in each region, while South Korea, Japan and the EU 27 together import 95Mt/yr in 2050. In the Intermediate Trade scenario, the annual green iron import volumes demonstrate a more uniform distribution between the regions. The green iron import volumes of China, India and RoW in the scenario account for 42Mt/yr, 61Mt/yr and 41Mt/yr in 2050, respectively, while the import volumes of the EU 27, South Korea and Japan remain equal between the trade scenarios at 25Mt/ yr, 39Mt/yr and 31Mt/yr, respectively.
Australia, Brazil and South Africa are the HBI-exporting regions, with Australia being the largest exporter with an annual export volume of 165-290Mt/yr in 2050, followed by Brazil with 65-115Mt/yr and South Africa with 12-22Mt/yr. Fig 7
Further research is required to better understand the practical potential of green iron exports or imports on the regional level. Since our study only considers domestically produced H2-DRI being
replaced by green iron imports, another important topic for future research is the potential of HBI imports to replace other domestic ironmaking routes – in particular blast furnaces. Finally, cost factors of green iron trade that go beyond hydrogen costs could be added to the analysis in future. While our analysis suggests that green iron trade could lower the cost of the steel transformation, globally, its practical implementation will hinge on issues ranging from challenges to scale-up renewable electricity sufficiently fast in HBI-exporting countries over certification issues, questions of self-sufficiency and strategic global partnerships between potential green iron importers. �
This research was funded by the European Union Horizon 2020 research and innovation programme under grant agreement no 101003866 (NDC ASPECTS). Thanks also to Fei Teng and Clemens Schneider, Lukas Hermwille and Tomas Wyns.
This article was previously published in Energy and Climate Change 5 (2024) 100161. For a full version, Google the following: ‘Global trade of green iron as a game changer for a near-zero global steel industry? – A scenario-based assessment of regionalized impacts’
The Energy Engineering Group and Process Metallurgy Group at Luleå University of Technology (LTU) details the current landscape of biocarbon production and utilization, alongside insights gained through its research initiatives. By Lena Sundqvist-Öqvist* and Kentaro Umeki**
ACCORDING to the World Steel Association (WSA) data for 2023, 71.1% of steel production was ore-based through the BFBOF route (blast furnace and basic oxygen furnace) and 28.6% was scrap-based through the electric arc furnace (EAF), resulting in a 7-9% contribution from the steel industry to global CO2 emissions. A major contribution comes from coke and coal use in the blast furnace (BF), a minor
part is related to the use of coal at the EAF.
disturbances or affecting the steel quality, i.e. alkalis and phosphorus, are low enough. Research shows that biocarbon can replace fossil coals in BF ironmaking i.e. replace injection coal at the BF, coke breeze at the sinter plant, being part of a self-reducing residue of briquettes, and replacing fossil coal in the coke. The optimum ratio for efficient self-reduction and requirements on coke strength *Professor, process metallurgy, LTU **Professor, energy engineering, LTU, figure prepared by Gangadharan Seenivasan, PhD student, LTU (To find out more about the FINAST project, follow this link: https://www.ltu.se/en/research/research-subjects/machine-elements/finast)
Carbon is essential in metallurgical processes, and the question is how fossil coals presently used can be replaced by biogenic renewable carbon (so-called biocarbon). Reported research implies that biomass converted to charcoal (biocarbon) with properties resembling those of fossil coal can replace fossil coals as long as the content of elements causing process
limits the maximum addition. It may be theoretically possible to replace around half of the fossil coals depending on the prerequisites at the specific steel plant. Together with carbon capture technologies (CCS/CCU), negative CO2 emissions might be possible. However, a huge amount of biocarbon demand is a hinder to the implementation, and fossil coals for coke making will still be required. Presently, several steel producers in Europe and globally aim for fossil-free
steelmaking through hydrogen (H2) based direct reduction to produce carbon free DRI (H2-DRI). H2-DRI are melted in and EAF, submerged arc furnace (SAF) or other type of furnace. To make the process route fossil-free, renewable sources of carbon must be used to reduce in H2-DRI remaining iron oxides, foam the slag and dissolve carbon in the steel during the smelting step. The required biocarbon quantities are reasonable as a major part of the energy input at the EAF is renewable electricity.
The conversion to fossil-free steelmaking makes understanding the functionality of biocarbon in the EAF process even more important, especially the effect of biocarbon properties on its contribution to reduction, slag foaming, and carburization, as well as methods to produce biocarbon from biomass of various quality.
FINAST project at LTU Energy Engineering Group and Process Metallurgy Group at Luleå University of Technology (LTU) are driving several collaborative research initiatives related to biocarbon production and utilization, one of those is part of FINAST. The FINAST project (Research and Innovation in Norrbotten for Advanced Green Steel Production and Manufacturing) is an interdisciplinary research effort in collaboration between LTU, Swerim and SSAB, and funded by the EU Just Transition Fund and The Swedish Agency for Economic and Regional Growth. The overall goal is to contribute with knowledge, tools and competence provision to enable a transition to climate-neutral production and use of steel. One of the focuses is to develop knowledge about how biogenic sources and green hydrogen can best be used in future steel production. The activities include biomass conversion technologies, biocarbon usage, and system analyses as well as industrial symbiosis.
One task of the FINAST project is the optimization of biocarbon production processes for steelmaking. The task aims at optimizing biomass pyrolysis processes and biocarbon post-treatment processes, such as leaching, to find a pathway to produce high-quality biocarbon suitable for fossil-free steelmaking. The task also investigates the use of waste streams from forest industries, such as bark and logging residues. The feedstock from waste streams often contains high levels of critical ash-forming elements, such as potassium (K) and phosphorus (P). It requires a dedicated knowledge about how these elements transform at high temperatures in pyrolysis reactors in order to build and adopt a mitigation strategy for these critical elements based on the origin of biomass feedstock.
Another task of the FINAST project is investigating the functionality of biocarbon in the EAF, taking aspects of future H2-based steelmaking via DRI-EAF route into consideration. The availability
of biocarbon is crucial and, therefore, this study investigates biochar of varying quality relating to its origin and processing route. The impact from slag composition, with future smelting of DRI and scrap in different proportions is also considered. DRI will introduce iron oxides and gangue, which requires reduction work, a higher slag
rate, a higher need of flux addition, and a different slag composition.
Biocarbon production and biomass resource
Biocarbon is carbon-rich (up to 80-95%) solid materials produced from biomass resources. Of the three major technologies
for biocarbon production, which include torrefaction, hydrothermal carbonization, and pyrolysis, only the latter, at relatively high treatment temperatures (600-800°C), can satisfy basic requirements for biocarbon properties (for example, elemental carbon and fixed carbon content). In the pyrolysis reactor, biomass particles are heated in
an oxygen-free environment to release volatile matter and leave around 15-30% of biocarbon.
Although biomass pyrolysis has been used by humans since early civilization, there are many challenges to make biocarbon that is suitable for steelmaking in an economically and environmentally sustainable manner. Some major issues related to biomass resource, biocarbon production and properties are summarized in Fig 1. In particular, biocarbon yields are low when quality is prioritized. Another notable issue is the complexity of storage and transport because biocarbon can experience self-heating and sometimes selfignition when stored in a large volume.
With this context, one of the focuses of biocarbon production in Sweden is utilization of waste streams from the forest industry, such as bark and logging residues, to avoid unnecessarily large demands from being added to forest value chains. However, such biomass assortments contain high contents of potassium, calcium, phosphorus, magnesium, and sometimes silicon. This calls for an effort to develop technologies for ash removal from biocarbon.
Technical challenges using charcoal, besides the risk of phosphorus impairing
and biocarbon is investigated through optical dilatometry and thermogravimetric analyses, which are shown in Fig 2. The gas evolution due to the reduction of FeO, which contributes to slag foaming, can be monitored as function of temperature. To support future fossil-free steel production via H2-DRI and EAF, the experiments include slags corresponding with different combinations of feed material and biocarbon of various quality.
Clean biocarbon without quality-disturbing mineral can replace fossil coals like anthracite in the EAF or in other smelting furnaces. However, to cover the quantities
Types and amount of biomass resources available to each country vary significantly and biocarbon production processes need to be flexible enough to be able to accept this variance. Biomass can generally be categorized into forestry, agricultural, and aquatic biomasses as well as biogenic wastes. Unless effective methods to remove critical ash-forming elements from biocarbon are developed, biomass contains excessive contents of potassium and phosphorus, with the exception of stemwood from forest biomass.
Because of the large forest industry (sawmills, pulp and paper mills), forest management and utilization systems in Nordic countries are well established.
steel quality, include the possibility of mineral components dissolving in the slag, which may increase its interaction with the ceramic lining and increase wear. Further, the ability to create slag foaming through the interaction between biocarbon and iron oxides contained in the slag may vary depending on the structure of biocarbon and contained minerals as well as slag composition.
The dissolution of carbon in the molten steel is also impacted by the biocarbon properties.
Present research conducted in the process metallurgy group at LTU involves laboratory experiments, supported by thermodynamic calculations. The interaction between slag
needed in steelmaking, it is essential to use biocarbon from low-quality biomass, also containing disturbing minerals. Therefore, future research needs to develop new methodologies in the production of biocarbon and also specific technologies that can modify the biocarbon to a quality suitable for use in steelmaking. Further, the research related to biocarbon in steelmaking looks into opportunities to utilize biocarbon with non-optimal quality, and how to handle this during smelting. These developments are foreseen to enlarge the available biomass base for biocarbon production, which is necessary to avoid excessively high costs due to the lack of raw biomass. �
If we are to halt runaway climate pollution while meeting global demand, the way we make steel needs to change. Currently, steel contributes between 7-11% of global CO2 emissions, and demand for the product is expected to increase by onethird between now and 2050.
Clean steel will only be possible with a rapid shift to low-emission production technologies – such as green hydrogenbased methods. For alignment with the International Energy Agency’s (IEA) 1.5°C scenario, an estimated 35% of ore-based steelmaking must be produced through hydrogen methods (specifically hydrogenbased direct reduction or H2-DRI) in 2050.
RMI and the Green Hydrogen Catapult (GHC), a coalition of ambitious green hydrogen market leaders, have embraced the challenge of decarbonizing the steel value chain and are actively
working towards this goal.
Introducing green iron corridors
To understand how we get there, we have to understand where we’re coming from. Traditionally, steelmaking all happened under one roof, where large integrated facilities supported both iron and steelmaking and where coal was used as the primary fuel and reductant. Because of this, cheap coal resources drove the geographic patterns of iron and steelmaking in the last century, with an advantage accruing over time to those who moved fastest to integrate into highly optimized facilities with economies of scale.
But with the advent of greener methods whose cost competitiveness is driven by renewable energy availability and
scalability, ironmaking will be drawn to new geographies rich in iron ore and renewable resources (demonstrated by new H2-DRI projects underway in Sweden where there is a combination of high-quality ore and costcompetitive wind and hydropower energy). It could prove cost-effective, and win-win, to split these processes up: with ironmaking taking place in one location with abundant ore and renewable energy potential and steelmaking happening somewhere else with steelmaking capabilities – and demand – already in place. This process splitting could open new markets for regions with abundant ore, but little steelmaking capability, and accelerate the global transition to low emissions iron and steel production, satisfying climate-conscious buyers who are serious about green steel. We call these potential export-import routes green iron corridors.
To decarbonize steel effectively, we need to look at all the processes that go into its production. Ironmaking – the most carbon-intensive step in the steelmaking process – is critical to this goal.
By Rachel Wilmoth1, Chathurika Gamage2, Lachlan Wright3, Sascha Flesch4
The business case for green iron corridors becomes clearer when strategically choosing export and import locations based on resources and needs. A sizeable portion of the cost to produce green steel is driven by the cost of renewable hydrogen, approximately 15–40% of the cost of steel. So, locations with cost-competitive hydrogen production and iron ore will have a competitive advantage. Depending on route-specific trade-offs between quality of renewable resources, cost and distance of seaborne transport, and cost and efficiency of shipping a more finished product of iron rather than iron ore, the iron reduction could either occur at the mining location or at a secondary location where renewable energy – and thus reduction – is particularly competitive before final shipment to steelmaking centres. Current subsidies will unlock cost reductions for hydrogen-based iron production in developed countries today, but ultimately the supply chain will
be optimized around competitive renewable energy resources.
Another key determining factor on the export side will be the iron ore resource — not all ore is created equal. Typically, DRI with Electric Arc Furnace (EAF) operations prefer iron ore pellets with an iron content of at least 67% and lower concentrations of key impurities such as silica, phosphorous, and alumina. To meet this requirement, ores are often beneficiated at the mine to separate the iron oxides from the impurities, producing a concentrate that is then pelletized. This process is already routinely done globally, favouring locations with high-grade ore such as Canada and Brazil, but also with low-grade ore, such as the 20–35% iron ore mined and upgraded in the United States.
Alternatively, for the ores that are difficult to upgrade to DRI-EAF grade quality due to specific composition, there is work underway to utilize additional downstream processing technology (e.g., Electric Smelting Furnace, ESF) which is already proven in other industries (e.g., ferroalloy production) to remove the impurities. This downstream processing could provide a second life and alternative pathway for Basic Oxygen Furnace use alongside DRIs. Our cost analysis indicates either option as economically feasible, with the preference driven by existing infrastructure and ore specifics such as starting grade, iron-bearing mineral type (magnetite versus hematite), and specific impurity composition. Among major iron ore miners, investments are being made for both options, with Vale, Rio Tinto, and Fortescue increasing DR-grade pellet production via upstream beneficiation and Rio Tinto and BHP are investing in downstream ESF pilot facilities.
Given these various drivers, an understanding of today’s most competitive iron ore reduction locations requires a combined view of subsidies, iron ore quality and management, shipping distances, and renewable resources. RMI, with support from technical advisors and GHC members, has developed technoeconomic modeling to identify these cost-competitive regions and evaluate corridor trade-offs between these factors. The modeling confirms lowest-cost export options with highgrade iron ore co-located with favourable renewable energy resources and enabling policies that can produce iron at $390 per ton – comparable to recent global prices. Shown in Figs 1 and 2, these export
locations include the United States (due to IRA subsidies and tax credits), South Africa, Canada (with tax credits), Mauritania, Australia, Brazil, and Chile. Pairing these export regions to importers with strong steelmaking capacity, reliance on iron ore imports, and demand for green hydrogen to meet energy security and decarbonization targets enables a clear business case for top importer regions of Europe, Japan, and South Korea to develop a blueprint for green iron corridors.
Green iron corridors can offer efficiency, cost, and growth opportunities across the steel value chain. As countries are establishing hydrogen strategies, regions such as Northern Africa and Australia are emerging as promising green hydrogen exporters while others will rely on imports to supplement their domestic production capabilities, such as the EU’s Hydrogen Strategy target of importing 10 million tons of green hydrogen by 2030. When these export and import regions overlap with existing iron ore trade flows, transporting finished green iron sees both cost and energy savings compared to transporting hydrogen and ore separately. Few infrastructure changes will be needed on the shipping side for this transition, as briquetted green iron, also known as Hot Briquetted Iron (HBI), can be handled and shipped via similar processes as existing iron ore and act as a vector for hydrogen trade.
The main benefit of a green iron corridors approach for importers is to lower costs and enable a faster transition for their steel sector. Crucially, it can help to avoid some of the domestic H2-DRI production infrastructure spending that is required for 1.5°C alignment, while still seeing cost and efficiency savings. At least$5.5 billion has been allocated to 10 commercialscale hydrogen-ready DRI facilities by government funds in Europe, but even with these generous subsidies, companies are struggling to reach final investment decisions, citing high costs for domestic hydrogen as a financial barrier. Instead, companies are already considering green iron imports into Europe from regions with lower hydrogen costs as a way to lower their emissions in a cost-efficient manner while still keeping steel production in Europe (to put the $5.5 billion government investments in perspective, it is estimated that it would require $105 billion in capital investments for new steel facilities
Fig 1. By choosing export and import locations based on resources and needs, Green Iron Corridors can offer efficiency, cost,and growth opportunities across the steel value chain
Fig 2. Green iron production is cheapest in regions with a combination of optimal renewable resources, high grade iron ore, and enabling policies. Levelized cost of Hot Briquetted Iron (HBI) locations chosen based on existing mines in key iron ore exporting regions
•
•
•
•
•
3. Green iron corridors split the iron and steel making processes with hydrogen and iron production in one region and steelmaking in another, offering cost and efficiency savings
and at least $330 billion for the associated hydrogen and electricity production capacity totaling $435 billion to transition the entire European integrated steel industry to hydrogen-based steelmaking).
Building out the infrastructure to provide roughly 5Mt of renewable hydrogen production to transition the integration, steel production would require 250 –350 TWh per year of electricity – a 10% increase from current generation in Europe – supplied by 150–350 GW of new renewables and using 4.5-10 million acres of a land-constrained region. Instead, some of this infrastructure spending and build-out can be avoided while also saving between 5–40% on the cost of clean steel by importing green iron rather than producing domestically (shown in Fig 3 with Germany as an example importer). These cost savings can be achieved while still maintaining domestic steelmaking activities, which make up approximately 75% of the iron and steel direct jobs.
In Europe, the combination of committed buyers demanding green steel who are
willing to pay 20-30% premiums for green products and implementation of carbon taxes makes a strong business case for establishing these trade corridors sooner rather than later. As EU Emissions Trading Scheme (ETS) free allowances phase out and the Carbon Border Adjustment Mechanism (CBAM) phases in by 2034, cost savings compared to fossil-based steel either produced in (55% of consumption) or imported into Europe (34% of consumption) can also be realized. For example, steel made in Germany from the lowest cost green iron imports compared to domestic fossil-based steel with projected ETS carbon taxes will be a roughly similar cost in 2028 and significantly cheaper by 2030 (up to 20% cost savings). Although cost is important, it is not everything: other factors such as an available skilled workforce, geopolitical risk, water and land availability, government support, energy security and equity, hydrogen readiness and enabling policy, and stakeholder engagement will also play a role in selecting key export and import locations.
The idea of green iron trade is gaining momentum, with interest from steel incumbents and on-ground development from new companies. Yet, advancement from concepts and MOUs to construction and production remains to be seen. To accelerate green iron supply chains, RMI and the Green Hydrogen Catapult will unite extensive sector experience, analytical capabilities, and system-level assessments to promote the advantage of corridor networks. Combining our research with supply chain convenings, we aim to kick-start the launch of first-ofa-kind green iron corridors with private and public partnerships. Action is needed across the value chain to make this a reality: governments must show intent, steelmakers in importing regions must show appetite, and iron producers and iron ore miners in exporting regions must begin laying the investment foundations. We can decarbonize steel production efficiently and cost-effectively with green iron corridors: now is the time to accelerate the transition. �
[
The Multi-Servo Array EDT delivers unrivalled roll texture control and consistency. The informed choice for surface critical strip. First orders secured. Please enquire below for further information.
[ Rollscan Roll Inspection ]
Surface to core roll inspection for back up and working rolls in hot and cold mill applications. A choice of units to meet your needs, backed by local engineering, service and support.
[ Strand Condition Monitors for Continuous Casting ]
Sarclad is your proven partner for a bespoke solution for your caster. In-Chain and off-line SCM solutions available. Please enquire below.
sales@sarclad.com
sales@sarcladna.com
sales.china@sarclad.com
sarclad.india@sarclad.com
With the demand for alternative iron products by North American steelmakers growing, it is widely believed that there is a need for continued investment in additional domestic direct reduced iron (DRI) and hot briquetted iron (HBI) – and possibly even pig iron – production capacity. By Myra Pinkham*
“I believe that the long-term future for alternative metallics is very bright as both demand and production is only poised to increase in the years ahead,” said Ronald Ashburn, executive director of the Association for Iron & Steel Technology (AIST), with demand growing not just in North America, but globally.
In fact, he said that driven by societal and market demands for greener steel, worldwide DRI output has grown by approximately 25.6% over the last five years with billions in capital being invested in greenfield facilities throughout Europe and North America. This, he said, is even the case in a world where steelmaking is dominated by the classic blast furnace (BF)/ basic oxygen furnace (BOF) route.
But while in most regions of the world about 75% of their steel is produced using blast furnaces, Thais Terzian, CRU’s principal steel metallics analyst, noted that it is a
different situation in the US where electric arc furnace (EAF) steelmakers currently have a 72% market share and where that share is expected to increase to about 75% of domestic steelmaking by 2029.
Philip Gibbs, a senior equity research analyst for KeyBanc Capital Markets, said that it is that proliferation of EAF steelmaking, particularly of EAF sheet and coil steelmaking, that has resulted in the use of alternative iron by US steelmakers to grow materially over the past decade or so, maintaining that it was the growth in the EAF steelmaking share that had, and continues, to result in that calculus to change.
That said, while US demand for alternative irons has been growing, it hasn’t been doing so excessively, Francesco Memoli, Tenova Inc’s president and chief executive officer, said, explaining, “One reason is that supply remains limited, both
*North America correspondent, Steel Times International
due to geopolitical reasons and other factors.”
Memoli said that he sees the Covid pandemic as the turning point, as before that expectations for US steelmakers to use more alternative iron weren’t achieved – at least not in volumes that were previously predicted. “However, over the past few years we have started to see a new beginning,” he said.
“And I believe that is a trend that is here to stay,” Timothy van Audenaerde, managing director and global metals lead for Accenture, said, especially if, as is expected, the share of US steel produced by blast furnaces continues to be reduced.
Audenaerde observed that currently scrap accounts for about 64% of US steelmaking raw materials with the DRI/HBI share being about 10% and the rest being pig iron. He said he expects that by 2050 the US DRI/HBI share will increase to 23% at the same time
as the scrap share increases to about 72% resulting in an even lower need for primary metal.
Clearly in 2024 there had been mixed performance in relation to pig iron, DRI and HBI, CRU’s Terzian said, although she pointed out that that was more related to supply than demand, explaining that due to the war in Ukraine, getting pig iron from Russia is no longer an option for the US and imports from Ukraine are uncertain.
But despite that, Alexander Kershaw, a senior Fastmarkets analyst, said that, supported by Brazilian material, US pig iron imports were actually up 10.6% yearto-date through November, and could continue to grow as new EAF steelmaking capacity comes online.
According to Philip Bell, president of the Steel Manufacturers Association (SMA), about 17 million short tons of new US EAF capacity is expected to come online by 2027. And at the same time as this is occurring, Audenaerde noted that more
EAF steelmaking capacity is coming online elsewhere in the world.
KeyBanc’s Gibbs noted that one big concern with all of this new EAF steelmaking capacity coming online has been where will the steelmakers get the raw materials they need to feed those plants – not just pig iron but also scrap, DRI and HBI. In fact, Terzian said that DRI consumption has been growing at a greater rate partly due to limited pig iron availability.
But at the same time there are questions about the availability of other steelmaking raw materials.
One frequent concern is whether there will be enough availability of prime scrap, which, Gibbs said, has been one of the motivating factors in the investment into certain scrap sorting technologies, including those converting shredded scrap into a more usable, higher quality grade by separating out non-ferrous metallics such as copper from the scrap.
Actually, it isn’t the scarcity, or a potential scarcity, of scrap that has been driving steelmakers to use more alternative iron products, Tenova’s Memoli said, stating that it has been quality-driven, including by such high-quality end-use markets as the automotive sector. “The higher quality that the steel grade is, the higher percentage of alternative iron or virgin iron units that steelmakers will use as opposed to scrap given the impurities that are contained in scrap,” he explained.
Memoli said that another driver is the push for greater decarbonization or sustainability. While at this time there isn’t any legislation mandating companies to produce steel with low emissions, he said that is something that is likely to materialize eventually. “Once it does, we could see increased production of green, low carbon emissions virgin iron units that don’t exist today, such as DRI, HBI and pig iron produced using direct reduction and hydrogen.”
Automakers and other steel consumers have increasingly been asking for more sustainable steel, Audenaerde pointed out, and as long as that continues, companies should be motivated to invest in more DRI and HBI production. He, however, admitted that this could be influenced by future environmental regulations and governmental incentives, which are things that are hard to predict.
“But it is more of a necessity than a desire for steelmakers to use more alternative iron,” KeyBanc’s Gibbs, said, explaining, “There is a need to find new raw material sources if they want to keep their supply chain flexible given that the only place they can get pig iron in a reasonable amount of time is from Brazil, and even that lead time is much longer than those for scrap.”
Gibbs noted that with the growing US EAF market share, some domestic blast furnace capacity pivot to also producing pig iron. Memoli added that pig iron is not only a marketable product, but it also allows mills to be less dependent upon imported pig iron. “That supply, however, would have an expiration date given that US blast furnaces are old and will eventually come to the end of their life.” Also, he said that pig iron production tends to have high carbon
emissions.
But AIST’s Ashburn noted that there have been a number of forecasts predicting that DRI demand could potentially outstrip supply with DR-grade iron pellets becoming the ‘chokepoint’ of steel industry decarbonization. However, he pointed out that one roadblock to greater longterm DRI production has been access to either cleaner burning natural gas or green hydrogen at a time when a viable green hydrogen market is materializing much slower than had been hoped.
However, Ashburn noted that during AIST’s recent European Steel Forum there were also considerable discussions about the potential decoupling of ironmaking from steelmaking. Ashburn said that this is based on the premise that in the future steelmaking would occur close to end-use markets while ironmaking will take place in regions with efficient access to iron ore and clean energy.
Therefore, he said that such a decoupling would bode well for North America, which has quality iron ore reserves and clean energy with regional access to nuclear, wind, solar and hydroelectric resources in addition to abundant reserves of natural gas.
Memoli pointed out that the share of how much alternative iron a steelmaker uses – and what kind of alternative iron they use – depends upon the product quality they are trying to achieve. For example, automotive steel grades –particularly those for exposed automotive applications – tend require a very clean raw material mix.
As for the various alternative iron products, Memoli said that HBI is often seen as being subpar when compared with pig iron, which tends to be considered the gold standard because of its quality and reliability. He said in between the two are low carbon cold DRI (CDRI), high carbon CDRI and high carbon hot DRI (HDRI) with the preference being for a high carbon product.
Memoli said he believes that there is potentially more interest in producing pig iron using new, less carbon-intensive technologies, such as ENERGIRON, a DRI technology jointly developed by Tenova and Danieli, which he said is well-suited to produce high quality alternative iron at a low cost, pointing out that Nucor uses this technology at its Louisiana DRI facility. Despite the slower than expected ramp up of that facility, he noted that Nucor is
currently producing a high carbon CDRI there at a record rate of 330.3 tons per hour.
With the US projected to see strong steel production growth later this year – about 13% year-on-year for steel produced via EAFs and about 4% year-on-year via blast furnaces as new EAF production capacity comes online – CRU’s Terzian said there is definitely a need for more domestic alternative iron production capacity to come online, hopefully at least an additional 3Mt/ yr DRI or HBI production plant.
Gibbs noted that despite this, very little US alternative iron capacity has come online over the past 12-18 months – other than US Steel’s 500kt Gary Works pig iron project. However, he added that given that there are several projects that are potentially in the hopper, it is possible that there could be an incremental increase of up to 4-5Mt of US alternative iron production capacity within the next five years.
Most of those projects, however, are in early stages and won’t come online for a while, CRU’s Terzian said, with the first likely to be Mesabi Metallics’ direct reduction (DR) iron ore pelletization project in Minnesota, which is slated to come online in 2026.
Also, in connection with the new EAF being installed in its AM/NS joint venture Calvert, AL, mill, Arcelor Mittal and Nippon Steel are reportedly considering doubling HBI production capacity at the Corpus Christi, TX, plant, but Terzian said that is very preliminary, describing it as more of a plan at this time than an actual project.
In addition, there are several projects that have been proposed that could be at least partially paid for through the US Department of Energy’s (DOE’s) $6 billion Industrial Demonstrations Programme, which is funded by the Infrastructure Investment & Jobs Act (IIJA) bipartisan infrastructure bill and the Inflation Reduction Act (IRA).
For example, Cleveland-Cliffs is in negotiations with the DOE for a $500 million grant to replace its Middletown, OH, blast furnace with a 2.5Mt/yr hydrogenready DRI plant and two 120-megaWatt electric melting furnaces (EMF) to feed molten iron to the existing infrastructure already on-site, including the BOF, caster, hot strip mill, and various finishing facilities. With this, the mill will continue to have approximately three million net tons per year of raw steel production capacity but
will no longer use coke for iron production.
Accenture’s Audenaerde, however, noted that questions remain over the availability of green hydrogen for these projects given the need to have affordable renewable energy to effectively produce hydrogen.
That said, Memoli pointed out that there has already been a successful hydrogenbased DRI pilot plant in Sweden and that Tenova has built two plants in China that are producing more than 1Mt of DRI using over 70% hydrogen. “So, hydrogen-based DRI is no longer just a new developing technology,” he said. “It is now a reality.”
He said that the next new technology to be developed is one for producing pig iron
using direct reduction. That, he explained, would mean that it doesn’t have to be produced using a blast furnace and would be cheaper, as well as resulting in very low carbon emissions.
While it might not be in a straight line, Audenaerde said there will continue to be growth in alternative iron use and investments in production capacity.
Gibbs agreed, noting that there are already a lot of projects on the drawing board and that more are likely to be added as more US EAF production capacity comes online. “But only time will tell how many new plants will come online, over what time period.” �
Future Steel Forum 2025, our seventh live event, unites decarbonization and digitalization in modern steelmaking. With presentations from leading steelmakers including home-grown Spanish company CELSA Group as well as newcomers to the world of green steelmaking like Hydnum Steel, and not forgetting other leading European players and larger Far Eastern businesses, such as JFE Steel, it looks as if the Forum will score big with delegates once again. Secure your spot today!
SPONSOR OR EXHIBIT: For any exhibition or sponsorship opportunities, contact Paul Rossage paulrossage@quartzltd.com +44 (0)1737 855116
WHAT LAST YEAR’S ATTENDEES HAD TO SAY…
“Very well organized event with a good line of speakers focused on digitalization and decarbonization.”
Scan the QR code to preview the conference programme, or visit the website to find out more
“Great immersive atmosphere and excellent platform to learn and network.”
“Unique blend of upcoming technologies, challenges and the latest innovation in digital transformation for the iron and steel industries.”
“A good mixture of high-level presentations, panels and networking opportunities at an excellent location.”
Carbon Clean, a carbon capture technology company, is aiming to accelerate the drive toward sustainable steel production through its columnless, modular designs. By Aniruddha Sharma*
1. How are things going at Carbon Clean? Is the steel industry keeping you busy?
Innovation drives us – we’re constantly pushing the boundaries of what is technologically possible so that hard-toabate sectors such as steel can reap the business benefits of decarbonization. We’re transforming carbon capture so the technology can be easily replicated and rolled out at scale, just like EV batteries and solar panels. This can only be achieved through designs that are fully columnless and modular, which is why we launched the CycloneCC C1 series. By eliminating the columns used in conventional carbon capture plants we’ve created a scalable, repeatable design that can be massproduced.
2. What is your view on the current state of the global steel industry?
Steel is one of society’s most vital industries, and crucial to human progress. We recognise the challenges the sector faces, from tariffs to technology. Regulation, such as the EU’s Carbon Border Adjustment Mechanism (CBAM) will have a significant impact. In India, for example, steel exporters are exposed due to comparatively high current emissions. Around 23% of all Indian iron and steel exports go to the EU, all of which will face the incoming CBAM. Carbon capture, utilisation and storage (CCUS) offers a viable route to net zero for the steel industry. Our focus is on helping steel producers cut their emissions in a costeffective manner.
3. In which sector of the steel industry does CARBON CLEAN mostly conduct its business?
As carbon capture experts, we work across the entirety of the steel industry, capturing emissions at source using our modular technology. Our solutions are particularly beneficial for blast furnaces, delivering high
performance and high-capacity capture at different concentrations of CO2. The steel sector accounts for around 7% of global greenhouse gas (GHG) emissions, so the pressure is on to generate significant reductions.
4. Where in the world are you busiest at present?
The Global Carbon Capture and Storage Institute recorded a 60% year-on-year increase in the global CCS project pipeline, with 2024 another year of exponential growth in CCS project development. This capacity growth has been concentrated in North America, Europe and the Middle East. We are experiencing this international momentum across these key geographies and have seen good progress in the AsiaPacific region.
5. Can you discuss any major steel contracts you are currently working on?
We recently signed an agreement with JSW Steel and BHP, exploring the feasibility of our modular CycloneCC technology to capture up to 100kt of CO2 per year –
*Chair and CEO, Carbon Clean
the largest scale CycloneCC deployment to date in steelmaking. Utilisation – the ‘U’ in CCUS – is a key component of the project, with the intention being to liquefy captured CO2 so that it can be sold locally. Our semi-modular technology is also being successfully deployed by Tata Steel in India, capturing carbon from a blast furnace to make it available for on-site reuse.
6. Where does Carbon Clean stand on the aluminium versus steel argument?
For us, what’s important is bringing down the cost of carbon capture so that it is commercially viable. For too long, cost and space have been an obstacle constraining widespread adoption of carbon capture. With CycloneCC, we are cutting the total installed cost and the physical space required by up to 50% compared to conventional solutions.
7. What are your views on Industry 4.0 and steelmaking?
Digitalisation and artificial intelligence (AI) hold great promise for the optimisation of many industrial processes. As carbon capture becomes increasingly embedded in the steelmaking industry, AI will help to improve the overall reliability and efficiency of production. A step change will be to move from providing insight and analysis to ‘closed-loop’ systems where AI makes independent decisions and acts upon them.
8. In your dealings with steel producers, are you finding that they are looking to companies like Carbon Clean to offer them solutions in terms of energy efficiency and sustainability? If so, what can you offer them? We’ve been driving the decarbonization of hard-to-abate industries for more than 15 years and have 49 technology references globally. We offer the steel sector two carbon capture routes, both
using our proprietary APBS-CDRMax solvent, which out-performs the industry standard. CaptureX is our semi-modular, cost-effective alternative to open-plant construction, delivering exceptionally high capture rates at high capacity. Our most recent innovation is the fully columnless, modular CycloneCC C1 series, which captures up to 100kt of CO2 a year. CycloneCC’s ‘plug and play’ design makes it straightforward and cost-effective to install, and units can be easily added as capture capacity increases.
9. How do you view Carbon Clean’s development over the short-tomedium term in relation to the global steel industry?
We’re an established CCUS partner to the steel sector, and this is set to continue. Carbon capture is essential for protecting jobs and enabling the steel sector to make the clean energy transition. Electric arc furnaces require major investment, which is why carbon capture is such an important technology for blast furnaces. We’re mindful of the steel industry’s focus on profit, so it’s crucial to convey the business case for carbon capture beyond its decarbonization value.
10. How optimistic are you for the global steel industry going forward and what challenges face global producers in the short-tomedium term?
Increased global demand for steel will bring challenges as net zero targets loom, as the sector is one of the hardest to abate. CCUS is widely recognised as an essential decarbonization lever.
Policy incentives that crowd-in funding are catalysts for increasing deployment and encouraging first-of-a-kind projects that accelerate technological progress. Striking the right balance in the ‘carrot and stick’ approach is key – and some geographies offer more incentives than others.
11. What exhibitions and conferences will Carbon Clean be attending over the next six months?
We’re at the penultimate stage in the commercialisation of CycloneCC and firstmover customers have the opportunity to influence the development of the fully commercialised product, so our main focus is on demonstrating this technology around the world. We’ll be in Houston during CERAWeek, of course, and we will be exhibiting and speaking at ADIPEC.
12. Carbon Clean is headquartered in the UK – what’s happening steel-wise in the area?
Decarbonization is high on the agenda of the new UK government. We look forward to the imminent Industrial
Strategy for more detail, in particular the role of CCUS for hard-to-abate industries. The government is also laser-focused on economic growth and making the country a clean energy superpower. We see industrial decarbonization as central to this mission.
13. Apart from strong coffee, what keeps you awake at night?
We’re witnessing a critical moment in history. The technologies to mitigate the impact of climate change are available, yet deploying them at the necessary scale requires stable, consistent policy over the long term. Policy certainty can never be taken for granted.
14. If you possessed a superpower, how would you use it to improve the global steel industry?
The ability to turn back time would be the ultimate superpower in mitigating the impact of climate change. The more realistic option is to radically reduce emissions produced by the industries society most relies on. This is why carbon capture is so important for the steel industry. �
Tebulo Robotics specializes in the design and supply of innovative robotic solutions that operates under extreme conditions.
With our many years of experience, knowledge in technology and driving forward advancements in engineering and robotics, we are able to offer a wide range of superior solutions that accelerate industrial processes.
Tebulo Robotics creates high quality robot applications that are the solution for making industrial processes faster, better, safer, cleaner and more productive.
The specialist task of casting guns, a role taken up by the appointed ‘Gunfounder’, serves as a salient representation of the advancements in metallurgical knowledge and skill between the 16th and 18th centuries. By Tim Smith*
IN the April 2020 edition of Steel Times International I wrote an article on John Browne, ‘The King’s Gunfounder’, who was an ironmaster with blast furnaces on the Weald of Southern England.
John was born in 1591 during the reign of James I (who was also James VI of Scotland) and died in 1651 having seen three monarchs during his lifetime and an 11-year period when Britain was a Republic (1649-1660), following nine years of the Civil War (1642-1651). In the war, Royalists, supporting King Charles I (James I’s son), and Parliamentary forces (Roundheads –named after the shape of their helmets), engaged in bitter battles across England, Scotland and Ireland. The Civil War broke
out when Parliament objected to Charles’ demands for them to raise taxes and, for this refusal, he had dissolved Parliament. Later to become the leader of the rebellion, Oliver Cromwell joined the parliamentary army at the start of the war and rose through the ranks, achieving the defeat of Charles’ son in Scotland in 1650 and a victory at Worcester in 1651, which ended the Civil War.
Charles I was captured a year later by the Scottish army and handed over to the Parliamentarians who tried him and found him guilty of treason – ‘a tyrant, traitor, murderer and Public Enemy’, carrying out the death penalty on 30 January 1649 by beheading. His son, also named Charles,
had fled to France following his defeat in Scotland.
John Browne had been appointed ‘Gunfounder’ to the ill-fated Charles I, following in his father’s footsteps when he retired from this role. John was thrown into prison by the Parliamentary forces in 1645 following the defeat of the Royalist army at the battle of Naseby, since John had been supplying guns and shot to the King’s forces. However, at this time, Browne was the sole competent founder of ordnance in the whole of Britain, so when the Parliamentary forces required guns to arm three new frigates, they reluctantly released him after just six-months. He accepted an undertaking to supply only Parliament with
guns and negotiated a lucrative contract to cast 86 guns of various sizes for these ships. Cromwell was appointed Lord Protector in 1653, two years after the defeat of the Royalists, but Parliament became increasingly dissatisfied with Britain as a Republic under Cromwell’s rule. Cromwell died in 1658 and Parliament voted to invite Charles I’s son to return to England to take up the throne as Charles II, which he did in 1660, accepting a limit to his powers mandated by Parliament.
Upon the restoration of the King, John Browne once again became ‘The King’s Gunfounder’, a role he retained until his death in 1651.
Browne cast both bronze and cast iron guns. The earlier cast guns were bronze (referred to as ‘brass’ in documents) at a time when iron guns were made from staves of wrought iron held together by hoops, sometimes with a cast breech. Attempts to cast a cheaper gun in iron failed at first due to their measurements being the same as those for the lighter section bronze guns demanded by the Navy to reduce the weight of ordnance on ships. Bronze gun measurements increased in 1538 and the casting of cast iron guns became possible. The first cast iron gun to be made in Britain was cast at Buxted, East Sussex, by Ralf Hogge (Huggett).
The weight of cast iron guns was a little less than bronze guns of comparable size – the density of grey cast iron – in which carbon is present as free graphite – ranges from 5.54 to 7.81g/cc as the quantity of graphite decreases. The density of bronze is in the upper portion of this, ranging from 7.8-8.9g/cc, depending on the copperto-tin ratio. More importantly, the cost of casting in bronze was some 270% higher than casting in iron. Browne even found a way of making cast iron guns lighter –known as Drakes – in which the bore at the breech end was tapered to provide thicker metal where the greatest forces were experienced on igniting the charge. Browne also offered guns in which the surface had been machined on a lathe to impart superior strength and appearance, but the additional cost of this process made the Navy reluctant to buy such guns.
The Weald produced in excess of 35,442 guns ranging in sizes from 42lb (19kg) shot to 0.5lb (0.22kg) between 1588 and 1770 – a time within which official records have survived. This number does not include guns that failed on initial inspection or test firing at the furnace site, or those sold clandestinely without license. Also, there are no numbers for the first 50 years from the casting of the first gun at Buxted in 1538 to 1588. During this period, some guns were cast for the Dutch – who sold some for a profit to the Spanish to recoup the cost.
As casting guns was a specialist task, only 38 furnaces of the 119 operating in the Weald were capable of undertaking this work. Guns were cast vertically in a pit in front of the furnace, a core being inserted in the mould to cast the barrel hollow. A bulbous gun head was included on the muzzle to supply molten metal to feed shrinkage cavities formed during solidification and also to act as a reservoir for slag and impurities to rise out of the gun into this head. The head was sawn off prior to reaming the gun to provide a smooth bore, and sold to the forges at a reduced price as, being grey iron and containing slag, they were more difficult to refine than the iron sows cast for forging which normally would be white iron. They were possibly not recycled through the blast furnace due to the effort needed to cut them into smaller pieces to safely do so but could be melted whole in a reverberatory air furnace, a number of which were established beyond the Weald as they required coal as fuel (a commodity not then
local to Southern England).
The casting of guns petered-out after 1760 when guns from other locations began to be accepted by the Board of Ordnance. Wealden guns diminished further in 1770, when the Carron company of Scotland started supplying the Board of Ordnance with guns, despite their early attempts producing inferior guns to those cast on the Weald. However, from 1775 the Board of Ordnance insisted that only
solidly cast and bored out guns would be accepted – a technology patented by ‘Iron Mad’ Wilkinson of Cumbria. This produced a sounder barrel without porosity – a weakness of hollow cast guns arising from moisture if present in the central core. This ended the Wealden gun trade, although reaming of surplus hollow cast guns continued into the 1780s to supply merchant ships and other markets.
The amount of iron needed for the largest guns would be around three tonnes. The largest gun type, the ‘Cannon’ had a 7” (178mm) bore and weighed up to 2.5 tonnes. The amount of metal to be cast to also supply the gun-head totalled 3 metric tonnes. The smallest gun cast on the Weald weighed around 400lb (180kg) requiring around 480lb (217kg) of metal. Evidence of a gun head still in place is a rare find, but one example has been found in Maryland, USA. These heads seem unusually large since, when casting grey iron as required for a gun, the graphite formed counteracts much of the shrinkage porosity, but no doubt trial and error determined this as the correct size. Since Wealden furnaces typically produced little more than 1.0 to 1.5 tonnes of iron a day, it would be necessary to allow an accumulation of metal in the hearth over a period of three
or four days to cast the larger guns, with the inherent dangers of a break-out of the metal or cooling causing a ‘freeze-up’ in the furnace. Indeed, a later gunfounder, John Fuller, in letters written between 1738 and 1751, refused to cast Cannon, writing: ‘will not make 42 pounders [19kg] shot at £20 a ton – a gamble at £80 a throw, also when making 4 tons of metal 1 ton is burned away……’
In 1664, George Browne (son of John) makes a similar comment regarding the time needed to accumulate sufficient metal: ‘…..being so long as four nights if not more in the hearth, which will be in great danger of cooling in the hearth when it should run which, when it happens (as in casting great pieces…is frequently to be
expected) is the loss of the piece’. Indeed, there are references to air furnaces being used in which metal could be melted and kept molten, certainly when casting bronze guns. The demand for guns varied greatly from year to year as illustrated in a plot for the 31-year period between 1668-1699, during which time 13,495 Wealden guns of all types were proofed in London.
During this period England was involved in no less than four wars and a rebellion, with peaks in the graph corresponding to the third Anglo-Dutch war (1672-74), the Franco-Dutch war (1672-1678) and the largest resulting from the Nine Years war (1688-1697).
This trend of variable gun production is also reflected for other periods. �