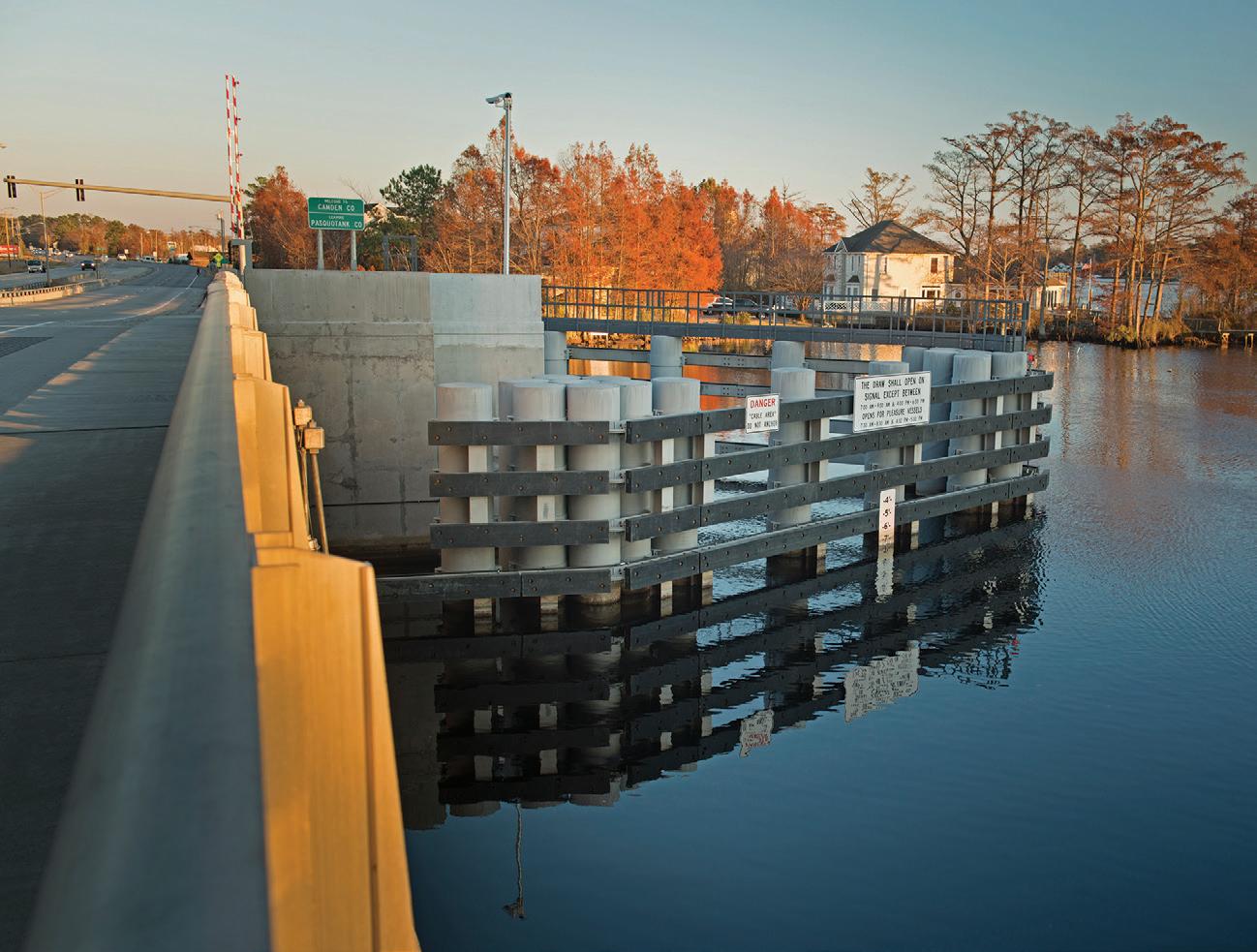
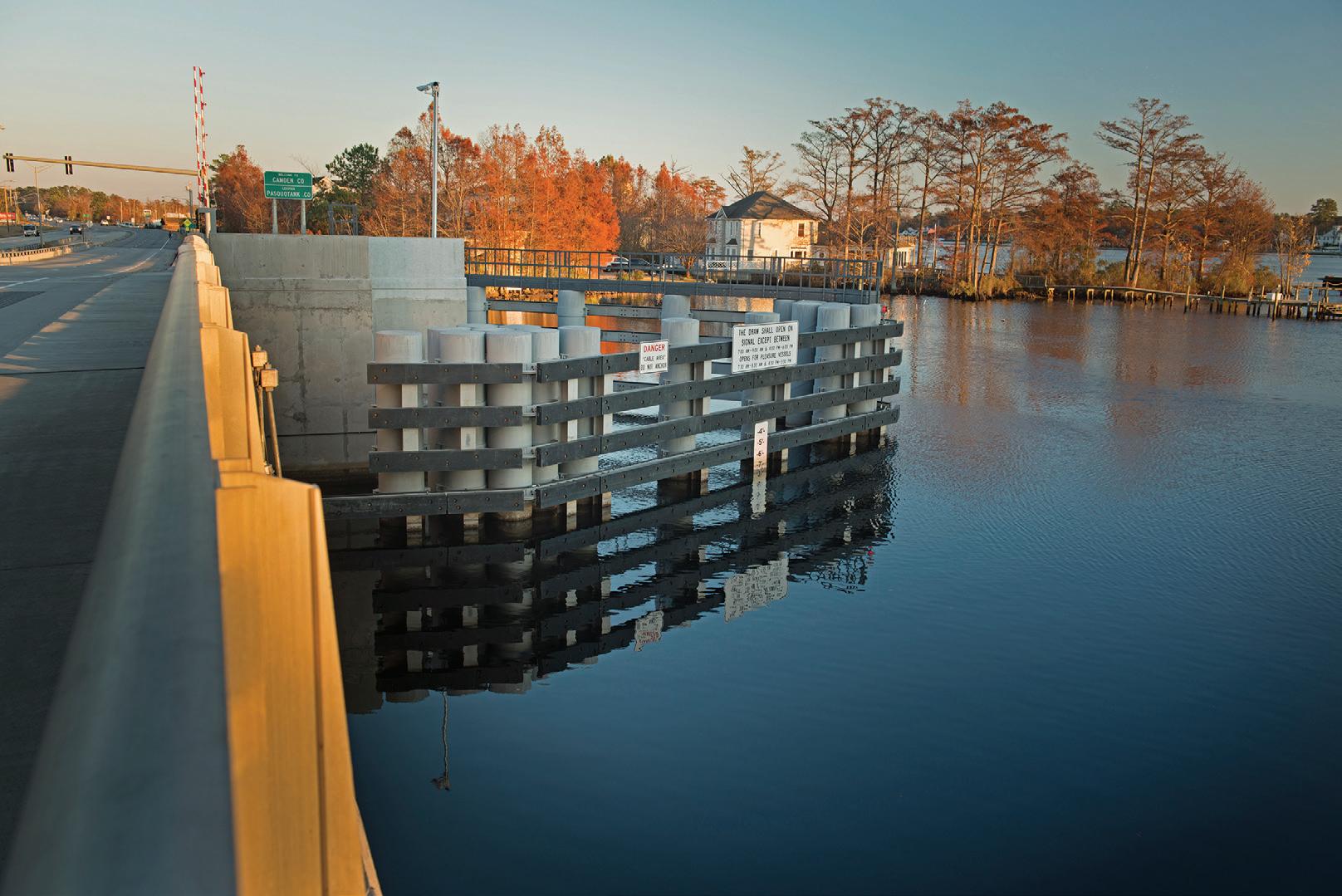
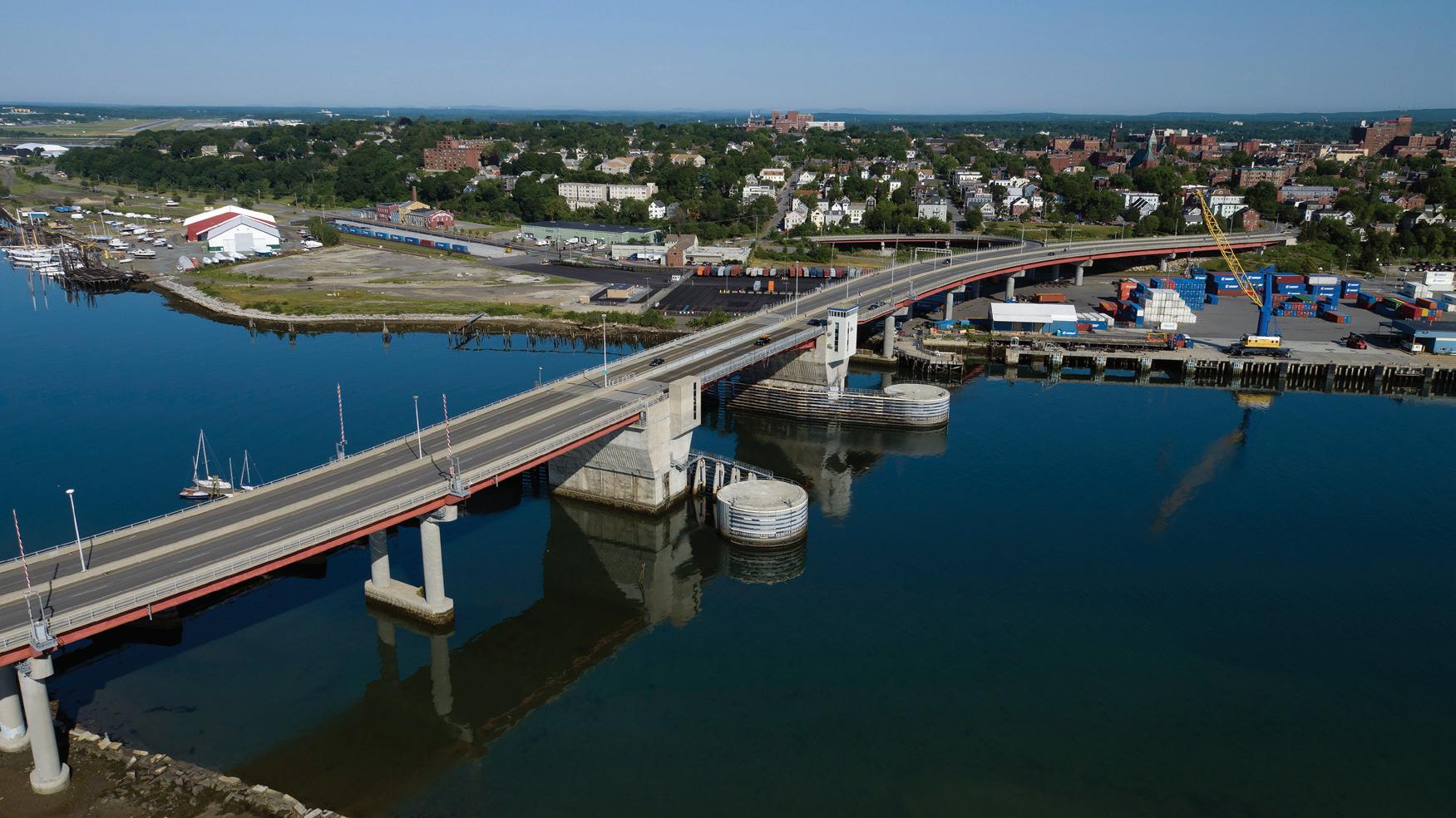
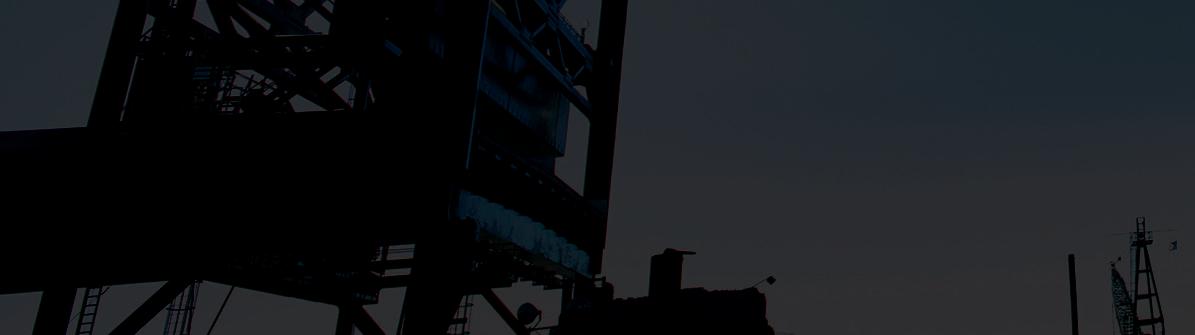

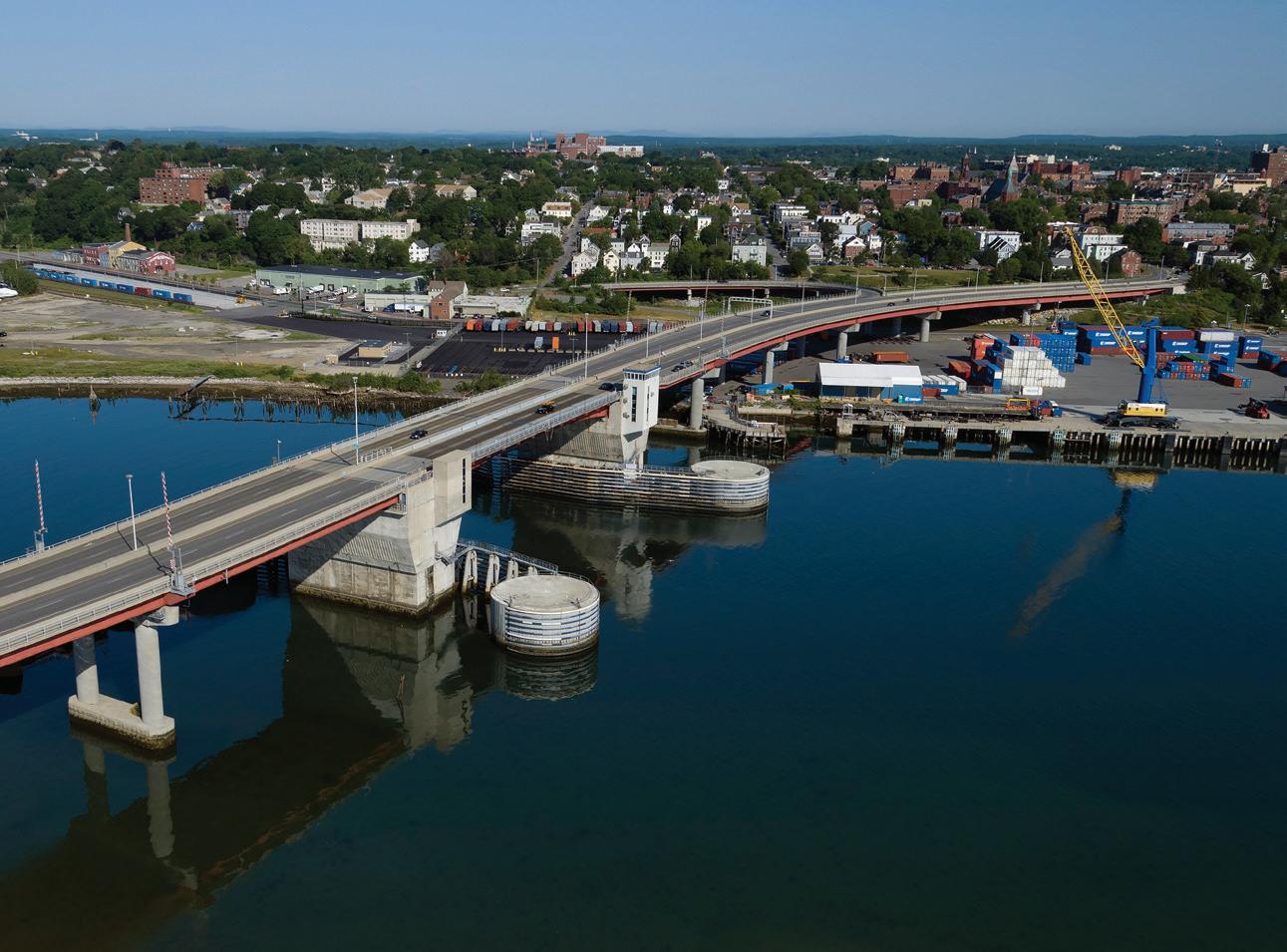
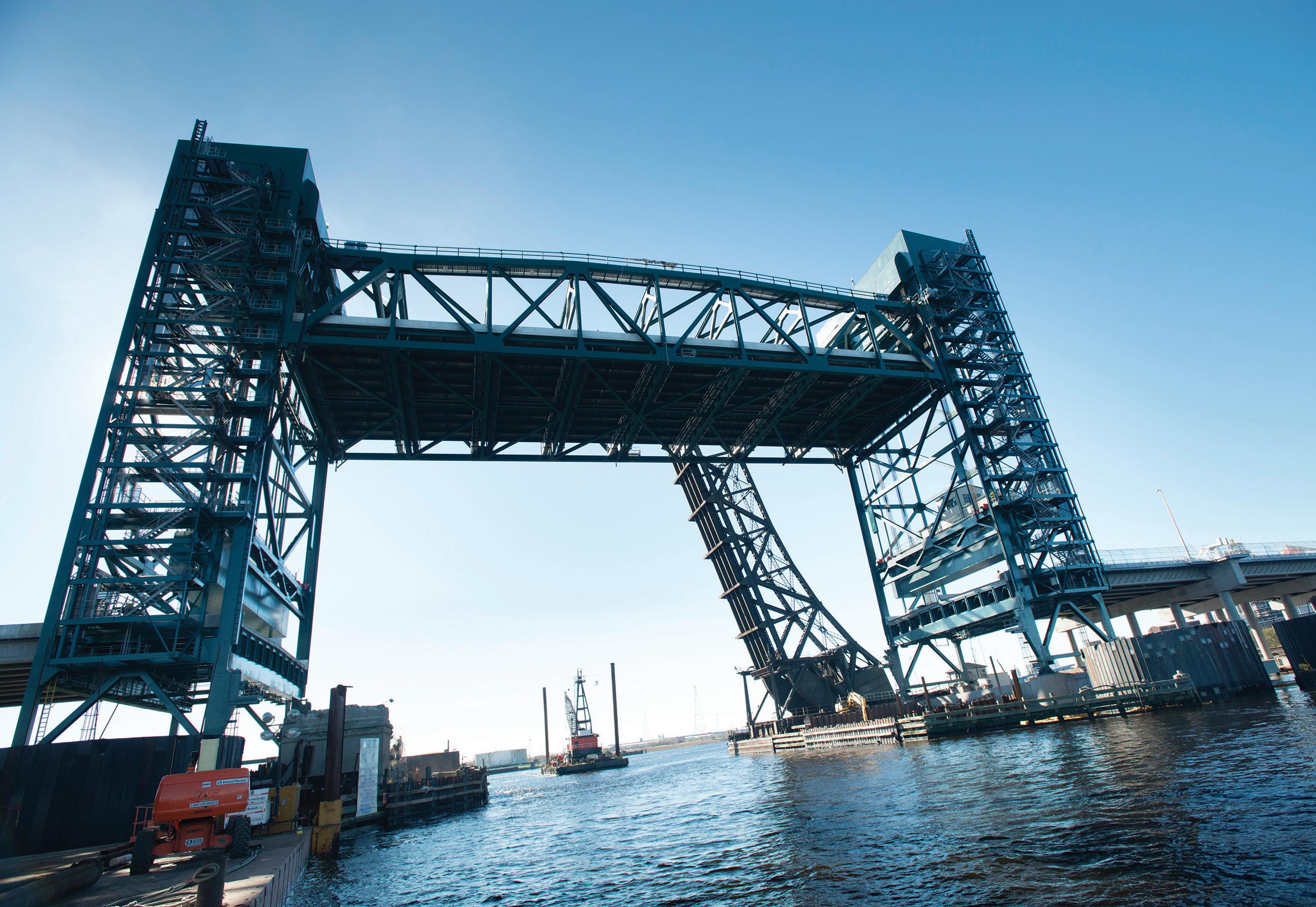
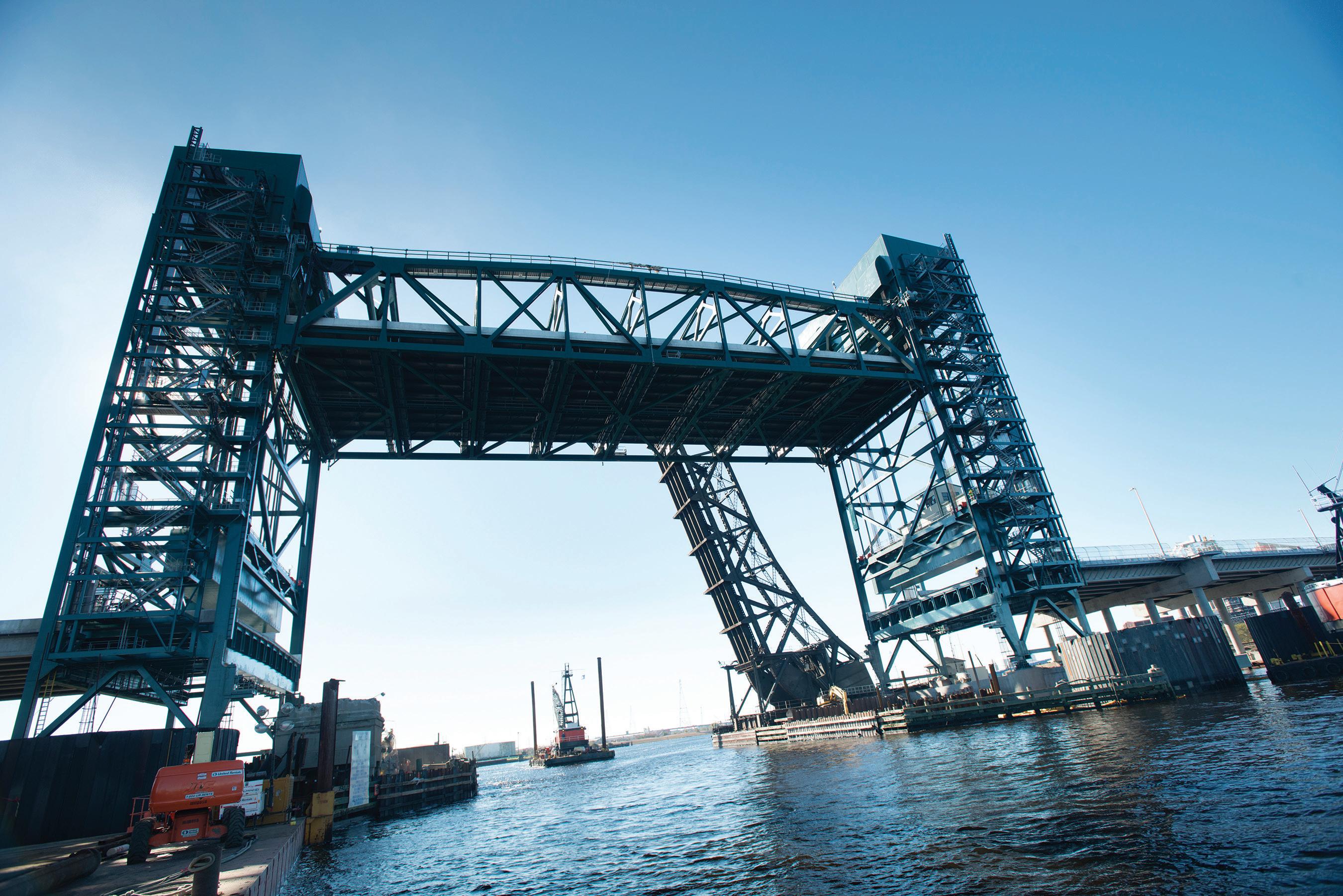
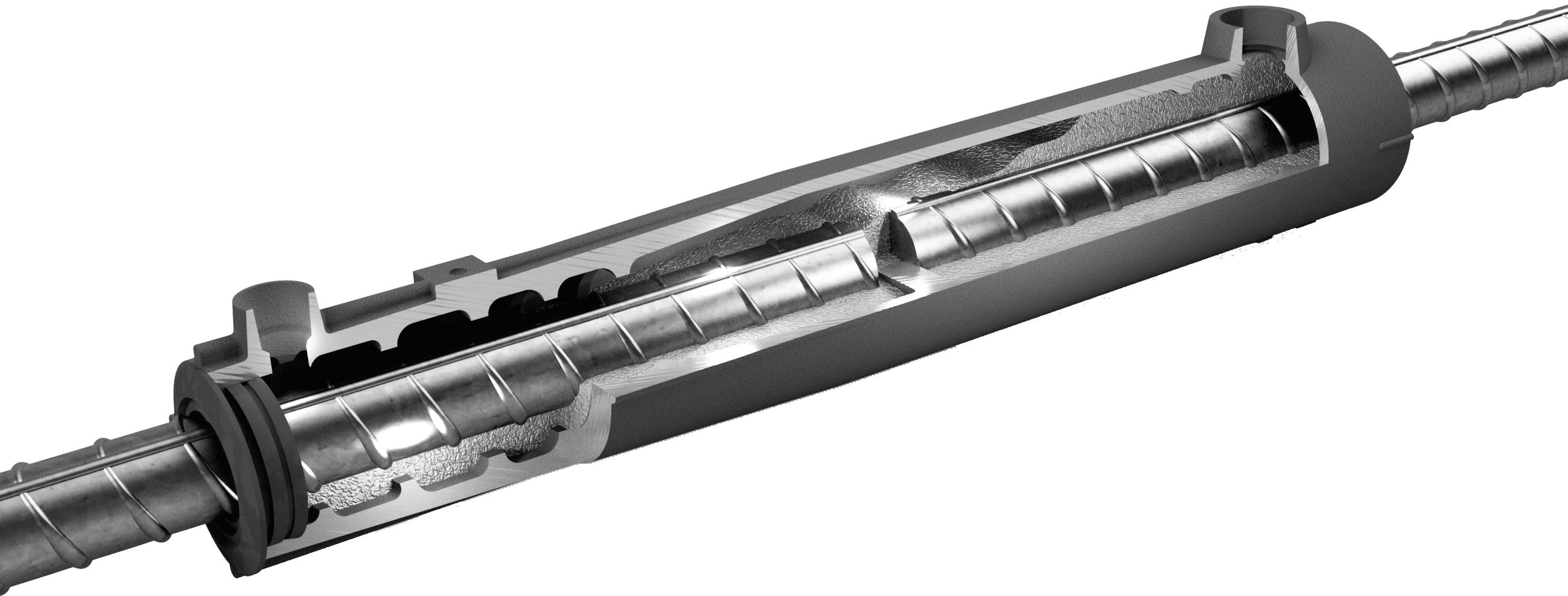
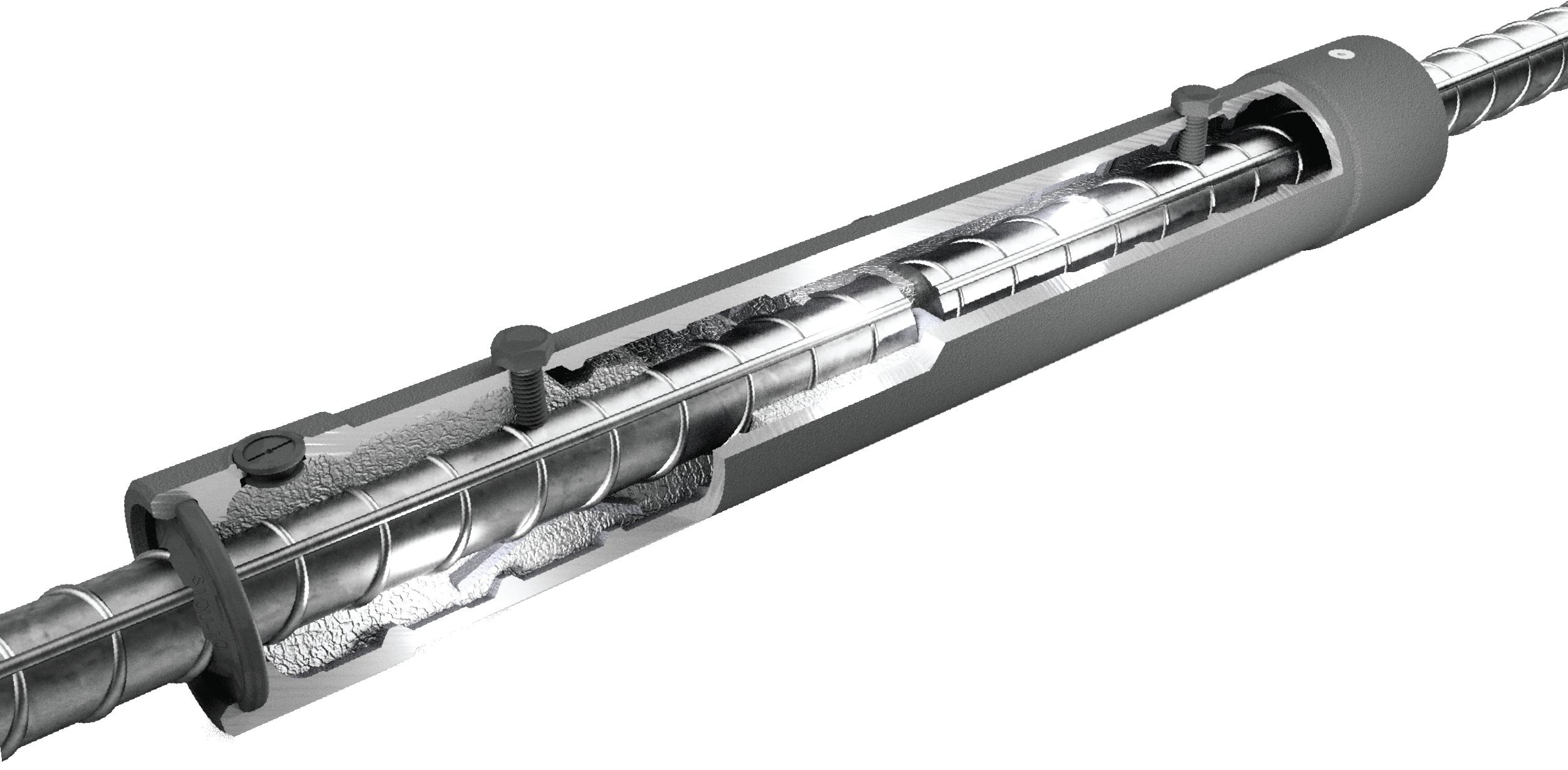

Anthony Canfor 27 ASC Steel Deck 82 Atlas Tube 87 Canadian Wood Council 37, 89 Chicago Clamp 3, 84
Computers & Structures, Inc. 71, Back Cover Gatefold CTS Cement 72 DEWALT 21 DuraFuse 76
Enercalc 8, 79
Hohmann & Barnard 25, 75
IDEA Statica 80
JLG 17
Simpson Strong-Tie 6, 73, 77
Splice Sleeve Inside Front Cover
Strongwell 24, 90
Williams Form 29
Support your rooftop loads in uplift and download conditions. The distributed load capacity of 4,000 lbs per complete system makes the Joist Grip Framing Clamp System the ideal support for rooftop unit installations.
Hanger Clamps, rated for 1,000 lbs each allow hanging of equipment below the roof deck while utilizing the ability of the Framing Clamps to distribute the load to panel points on the top chord of the bar joist.
Utilize the Panel Point Bridge to safely suspend HVAC equipment, conveyors or other ceiling fixtures without welding or drilling using the Suspension Clamp System.
Using corrugations in the standard roof deck, the Upper Deck Fall Protection Anchorage System easily clamps to the top chord of the bar joist or wide flange support.
subscriptions@structuremag.org
Chair John A. Dal Pino, SE Claremont Engineers Inc., Oakland, CA chair@STRUCTUREmag.org
Marshall Carman, PE, SE Schaefer, Cincinnati, Ohio
Erin Conaway, PE AISC, Littleton, CO
Sarah Evans, PE Walter P Moore, Houston, TX
Linda M. Kaplan, PE Pennoni, Pittsburgh, PA
Nicholas Lang, PE Vice President Engineering & Advocacy, Masonry Concrete Masonry and Hardscapes Association (CMHA)
Jessica Mandrick, PE, SE, LEED AP Gilsanz Murray Steficek, LLP, New York, NY
Brian W. Miller
Cast Connex Corporation, Davis, CA
Evans Mountzouris, PE Retired, Milford, CT
Kenneth Ogorzalek, PE, SE KPFF Consulting Engineers, San Francisco, CA (WI)
John “Buddy” Showalter, PE
International Code Council, Washington, DC
Eytan Solomon, PE, LEED AP Silman, New York, NY
Jeannette M. Torrents, PE, SE, LEED AP JVA, Inc., Boulder, CO
Executive Editor Alfred Spada aspada@ncsea.com
Managing Editor Shannon Wetzel swetzel@structuremag.org
Production production@structuremag.org
Director for Sales, Marketing & Business Development
Monica Shripka Tel: 773-974-6561 monica.shripka@STRUCTUREmag.org
• Concrete Repair Mortars
• Corrosion Protection
• Construction Grouts
• Waterproofing
• Sealants and Joint Fillers
• Coatings and Sealers
• Epoxy Adhesives
• Cementitious Flooring Systems
• Cure and Seals
• Densifiers
• Structural Strengthening Products
• Precast
• Epoxy Adhesives
Visit www.mapei.com/us for details on all MAPEI products. Your single-source provider for construction
MAPEI offers a full range of products for concrete restoration, waterproofing and structural strengthening. Globally, MAPEI’s system solutions have been utilized for such structures as bridges, dams, tunnels, highways, parking garages, stadiums and high-rises.
By W. Lee Shoemaker, Ph.D., PE
With a metal building anchoring its warehouse, Costco has designed its ½-million-square-foot roof for public recreational use in Mexico City’s Parque La Mexicana.
By Carlo Citto, PE, SE
The service life of a 135-year-old stone arch bridge was extended with a structural repair project that entailed a new reinforced concrete deck, cantilevered sidewalk, and stone masonry repair.
By Nathan C. Roy, PE
A history of the structural evolution of Boston’s Quincy Market.
and Ann L. Collins, Ph.D.
A plug-and-play structural bridge replacement meets the needs of fast-paced
John Dal Pino,
Ehsan Noroozinejad Farsangi, T.Y. Yang, Pejman Sharafi, Mohammad Noori, and Iman Hajirasouliha
By Gwenyth Searer,
PE, SE, Terrence Paret, Hayley Proctor, PE, and Prateek Shah, Ph.D.
Angelina V. Stasulis, PE,
Chris Cerino
Peter Atherton,
By Dr. Frank Griggs, Dist. M. ASCE
Goupil, PE
By John Dal Pino, PE, STRUCTURE Editorial Board Chair
Ithought it would be interesting to our readers if I explained what goes on behind the scenes at STRUCTURE and how a very dedicated group produces a high-quality magazine every month.
STRUCTURE was formed in 1994, and like any well-run organization, the magazine has a Mission, Vision, and Objectives. A summary is that we aim to be the premier resource for practicing structural engineers by publishing interesting, informative, and inspirational content that introduces new, innovative, and evolving technical concepts, presents lessons learned by examining past projects, and promotes structural engineering as a true and distinct profession.
The content in STRUCTURE is reviewed and driven by the active Editorial Board, all of whom are volunteers. Without them there would be no STRUCTURE magazine in its present form. I want to thank our current board for their service, dedication, and friendship. They are:
• Evans Mountzouris (20 years, DiSalvo Ericson Group (retired), Milford, Connecticut)
• Eytan Solomon (5 years, Silman, New York City)
• Jessica Mandrick (9 years, Gilsanz Murray Steficek, New York City)
• John “Buddy” Showalter (19 years, International Code Council, Washington, DC)
• Nick Lang (3 years, National Concrete Masonry Association, Ashburn, Virginia)
• Linda Kaplan (8 years, Pennoni, Pittsburgh, Pennsylvania)
• Marshall Carman (2 years, Shaeffer, Cincinnati, Ohio)
• Kenneth Ogorzalek (2 years, KPFF Consulting Engineers, La Crosse, Wisconsin)
• Sarah Evans (1 year, Walter P Moore, Houston, Texas)
• Jeannette Torrents (5 years, JVA, Denver, Colorado)
• Erin Conaway (7 years, AISC, Littleton, Colorado)
• Brian Miller (17 years, Cast Connex Corp., Davis, California)
I would venture that they, along with all our past Editorial Board members, probably didn’t remotely know what they were getting into when they first volunteered. I certainly didn’t.
As it turned out, the job is what I imagine it is like working at a newspaper: re-occurring hard deadlines, high standards for ethics, fairness, comprehensiveness, readability and accuracy, and lots of stress at times. I have been at it for 11 years. Many have said that engineers are not good writers (or editors) but I have found that not to be the case by a long shot.
We aim to be the premier resource for practicing structural engineers by publishing interesting, informative, and inspirational content.
I also want to thank our authors, for without them, there would be no content to publish. For most, writing is hard or harder than editing, and producing a worthy article takes much effort. Our authors make commitments and work toward schedules and deliverables set by the Editorial Board and endure and respond to the comments and edits we suggest. Without drawing a Venn diagram or presenting an org chart, let me explain in a few bullet points how STRUCTURE functions behind the curtain:
• Three overlapping groups work together: the Editorial Board responsible for content, the Content Editor responsible for overall content editing, layout and graphics, and Marketing responsible for business development and advertisements.
• Our main traditional article types are: Technical, Project, Insights, Structural Forum (structural engineering opinion pieces), InFocus (Editorial Board opinion pieces), Business Practices and Historical Structures (many thanks to Frank Griggs for his bridge articles).
• Each article is populated and tracked in a detailed database that looks ahead one year.
• Every article is assigned to a Board member who reviews it at the Draft and Final stages and approves it before publication. If necessary, we perform a second, independent internal review or engage the services of the NCSEA Code Advisory Committee.
• STRUCTURE is not a peer reviewed publication, but as you can see, we strive to be the next best thing.
• Authors work on a volunteer basis.
We have been working hard in 2024 to make the magazine more consistent in terms of organization, content, and appearance. We have also updated and freshened our website. Please have a look if you haven’t recently. All the credit goes to Shannon Wetzel, our Managing Editor and Director of Content, and Monica Shripka, our Director of Sales, Marketing and Business Development.
In the last year or so, we have developed two new article types we hope you like—Structural Influencers and Iconic Structures. To promote structural engineering, the Structural Influencer articles focus on the achievements and impacts current members of the profession are having. The articles are in an interview format (new to STRUCTURE). The Editorial Board and the individual Structural Influencers have had a lot of fun with these. The Iconic Structures series focuses on the impressive structures that are all around us (but we sometimes overlook) and the structural engineers who designed them.
Finally, thank you, readers, for your years of support: by contributing articles, reading articles, supporting our advertisers and providing effective commentary and feedback to us. I hope you now know a little more about STRUCTURE and all involved. If you have an opinion or gripe, send me an email. If volunteering for the Editorial Board sounds fun to you, contact me. Hope to see you all at the NCSEA Summit this November in Las Vegas. ■
John A. Dal Pino is a Principal with Claremont Engineers, Inc. in Oakland, California. He serves as the Chair of the STRUCTURE Editorial Board (jdalpino@ claremontengineers.com).
Bridge engineering is embracing risk management following the rare, large-scale Key Bridge incident.
By Jason Miles, PE
The recent collapse of the Francis Scott Key Bridge underscores the critical need to safeguard bridges from vessel collisions and highlights the impacts a major bridge failure can have.
In addition to the tragic loss of life, a major bridge collapse can have far-reaching adverse effects on the local transportation infrastructure and economy. Bridge failures not only snarl roadway traffic and slow down critical freight, but they can also block shipping channels and major ports for weeks or even months. While vessel collisions are more common than the public may realize, most incidents occur on inland waterways when barges impact bridge piers or their protection systems. Most of these collisions do not significantly damage the bridge or result in closures.
A large-scale collapse such as the Key Bridge is an exceedingly rare event—yet, it has raised awareness of the engineering challenges involved in bridge safety and prompted global concerns about similar vulnerabilities.
The Key Bridge collapse shares several similarities with the major collapse of the Sunshine Skyway Bridge in Tampa Bay, Florida, that occurred in 1980. The Sunshine Skyway Bridge was also a long-span steel truss, and the collapse was caused by an ocean-going vessel striking a primary pier. The incident resulted in 35 fatalities, drawing global attention and becoming a turning point for bridge design in the United States. It also spurred significant research which evolved into the bridge design codes for vessel collision assessment and protection that are still in use today. After that collapse, Modjeski and Masters developed the “Criteria for Design of Bridge Piers Against Ship Collision in Louisiana Waterways,” which was used by several states while the “AASHTO Guide Specifications for Vessel Collision Design” was developed, based in part on Modjeski and Masters’ work. Since then, Modjeski and Masters has continued to perform site-specific vessel surveys, risk assessments, and design of protective measures against vessel collisions.
Bridges that were built before the 1980s—prior to the development of unified vessel collision design guidelines—may be particularly vulnerable to damage or collapse. Many factors can increase vulnerability, including bridge geometry, superstructure type, pier type and locations, frequency and size of marine vessels, and waterway characteristics like current speeds and turns in the channel. It is important that existing bridges, especially older ones, are assessed for vulnerability by a method that considers all these factors. It is also important that these assessments are updated over time as conditions change, such as increases in marine vessel size or changes
in the waterway. If a bridge is found to be vulnerable, appropriate countermeasures specific to the bridge site can be employed to reduce the level of risk.
Container ships in particular have dramatically increased in size over the last 40 years. As open ocean port capacities increased with larger cranes and deeper dredging, shipping companies pushed designs of container carrying vessels larger to increase efficiency. In 2016, the expansion of the Panama Canal was completed, giving rise to new classes of “Panamax” vessels, further increasing the number of large container ships in use.
In vessel collision, ship size is typically expressed in terms of “dead weight tonnage,” or DWT. DWT is the total weight in metric tons that a ship can carry when it is fully loaded, including cargo, fuel, ballast water, and provisions. In the 1980s, large container ships generally ranged up to 50,000 to 60,000 DWT. The largest container ships being produced today exceed 200,000 DWT, representing a 400% increase in mass.
Designing piers and pier protection systems to handle collision loads from large ocean-going vessels can be challenging, and the construction costs associated with these systems can be quite high. Current AASHTO LRFD Bridge Design Specifications express
collision load in terms of DWT and vessel impact speed. As the excerpt from the code shows, lateral design loads for collisions from ocean-going vessels can be extremely high.
As we gain a better understanding of the risks our structures could face—and our capabilities of mitigating those risks increase—there is a growing expectation that our infrastructure should be designed and managed to provide resiliency.
Resiliency refers to the ability of a bridge or other section of infrastructure to recover from adverse events in a timely manner. It is important to consider the bridge in the context of the infrastructure system it is a part of. The Key Bridge is not only an important section of the highway network around Baltimore and the east coast, but it also spans a critical waterway and allows access to one of the busiest ports on the east coast.
Engineers have many tools at their disposal for mitigating vessel collisions. For a new bridge, the location and geometry of the crossing can be optimized to reduce the probability of a collision. For example, increasing the span length and locating the crossing away from sharp curves in the channel can reduce the chances that a vessel will strike the piers. Once a pier location is set, design options are available to mitigate the effects of an impact, many of which can be retrofitted to existing bridge sites. The most practical and cost-effective method in the long run is usually to design the bridge pier to resist the loads from an impact directly. Piers that are appropriately sized for vessel collision minimize future maintenance issues, as no external mitigation systems that require inspection and periodic repair or replacement are needed. Additionally, stand-alone pier protection systems can make up 25% or more of the total cost of a new bridge.
For existing structures, engineers frequently employ the use of dolphins, which are large structural elements placed around the pier intended to deflect vessels and absorb the energy of a collision. Fender systems, intended to guide vessels away from piers while also absorbing energy, are also commonly used. Other options include dikes and artificial islands around piers that a vessel would ground on prior to striking a pier. Dikes and artificial islands can be very effective, even for the largest vessels, but they require significant clearances between the pier and navigational channel. This makes them not a feasible option in many cases.
Designing structures for extreme events, such as vessel collisions, hurricanes, earthquakes, and tsunamis, presents a significant challenge for engineers. As time goes on, issues like climate change and increasing vessel size only add to this challenge.
In the past, damage from extreme events was generally accepted, including the loss of services that went along with that damage. Now, expectations have shifted, and we expect our infrastructure to be able to resist extreme events, or be able to return to service quickly after damage occurs. Engineers have the knowledge and tools to meet these challenges, but this requires a commitment to long-term planning and appropriate funding levels.
In the past 40 years, one of the most significant advances in bridge engineering has been the shift from deterministic approaches to probabilistic methods.
A deterministic approach is one-size-fits-all; a design load is determined, and the structure must be designed to withstand that load. A probabilistic approach looks at overall risk and can consider many factors and their chances of occurring. This allows engineers to adjust the target performance level of a structure during design to best meet expectations of the owner. Bridges considered to be critical can be designed to higher performance levels. This approach acknowledges the economic choices owners are often faced with, allowing limited resources to be spent where they are needed most.
As infrastructure ages and environmental conditions evolve, it is imperative that we continue to prioritize rigorous assessments, innovative engineering solutions, and strategic investments to safeguard these critical structures. By doing so, we can enhance resilience, protect lives, and ensure the function of our vital transportation networks for generations to come.
of special projects, including scour analysis and soil-structure interaction, structural health monitoring, steel truss rating and rehabilitation, and post-collision damage assessment and repairs.
The role of bond patterns and their implications for masonry design are explored.
By Kirby Beegles, PE, SE
Masonry construction is prevalent in buildings as a material that is straightforward to assemble and can be sourced locally to each region. Beyond its widespread use across various building types, masonry's true marvel lies in its ability to meet diverse design requirements through a singular, composite system:
• Its structural integrity is a key benefit, offering resistance to both gravity and lateral forces.
• It acts as a reliable fire barrier, safeguarding different occupancies, stairways, or building exteriors.
• Masonry can incorporate insulation to contribute to an effective thermal barrier.
• Its resistance to moisture-induced decay ensures long-term durability;
• Masonry offers the flexibility to be crafted for a high-quality and distinctive aesthetic.
One technique used to achieve a unique appearance with masonry is varying the bond pattern. Most masonry walls use rectangular blocks, and these blocks are often built with each course offset one-half the width of the course below in a pattern called “running bond.” Refer to Figure 1 for an example of a running bond pattern as well as other types of patterns. The conventional running bond, with its staggered courses for a balance of strength and efficiency, contrasts with “stack bond” where vertical joints align or nearly align (Fig. 2).
This article delves into the implications of the bond pattern on the structural design of masonry. While a wide range of bond patterns can be used, for practical purposes the most commonly used are the running bond and horizontal stack bond patterns. Other patterns, such as diagonal basket bond, diagonal bond, and basket weave are beyond the scope of this article.
The term “stack bond” is not explicitly defined in the Building Code Requirements and Specification for Masonry Structures (TMS 402/60222). Instead, it is referenced in the commentary as one type of bond pattern that is “not laid in running bond.” Running bond is defined to exist when the overlap of units is at least one-fourth of the unit length. In typical running bond construction, the units overlap by one-half of a unit length; however there may be situations where it is desired to use less of an overlap for aesthetic purposes. The code recognizes that stack bond, where the vertical joints of the blocks are in alignment, is one type of pattern that does not achieve the required one-quarter unit overlap; however, any bond pattern where the vertical joints overlap by less than one-quarter are subject to special provisions in the code for masonry “not laid in running bond” (Fig. 3).
The building code requirements for masonry were primarily based on structural testing and research performed on wall panels laid with
running bond construction. The default assumption in the code is that masonry is laid in a running bond pattern, therefore, when other patterns are used there may be some effect on the strength of the wall.
The Portland Cement Association (PCA) performed structural tests in accordance with ASTM E72 (Standard Test Methods of Conducting Strength Tests of Panels for Building Construction) to determine the impact of varying bond patterns on the strength of masonry walls constructed with hollow units. The tests considered vertical loading with an eccentricity equal to one-sixth of the wall thickness to determine compressive strength, and a uniformly applied lateral load to determine bending strength (Portland Cement Association, 1961).
There was no decrease in the compressive strength for different bond patterns when blocks are laid in the horizontal running or stack bond position. However, walls tested with blocks laid in the diagonal or vertical position exhibited some loss of compressive strength due to the reduction in net area associated with those patterns (the webs of the vertical units do not align with respect to the direction of force, therefore do not contribute to the strength). The result of this outcome is that the equations for compression in the code do not require reductions in strength based on the pattern used.
In many patterns, including horizontal stack bond, the flexural strength of a wall spanning vertically was surprisingly determined to be higher when compared to running bond. Only three patterns (Vertical Stack, Basket Weave B, and Coursed Ashlar, refer to Figure 1) resulted in a reduction in vertical flexural strength. Horizontal Stack Bond, a commonly specified alternate to running bond, was one of the patterns found to have a higher flexural capacity when spanning vertically between supports. For walls spanning horizontally between supports, stack bond construction was shown to be considerably weaker in flexure. One example of this condition is a CMU shaft at the perimeter of a building (Fig. 3). In this case, one or more walls are exposed to exterior wind pressure and do not connect to a diaphragm to allow the wall to span vertically. The exposed wall must span horizontally between the perpendicular walls at the corners of the shaft. Testing of this condition showed that the flexural strength of the stack bond masonry was approximately 30% of running bond construction. It is important to note that the testing was performed on hollowblock panels that did not contain any reinforcement. The effect of reinforcement on the capacity of masonry walls is discussed in the following sections.
The most significant impact of the bond pattern is on the flexural strength for horizontally-spanning elements. Even though tests showed masonry exhibits some flexural strength with bond patterns other than running bond, the code sets a flexural tension stress value for unreinforced masonry to 0 psi when using these patterns in horizontallyspanning walls (refer to tables 8.2.4.2 or 9.1.9.1 (TMS 402-22)). To compensate for this reduction in flexural strength compared to running bond, masonry designed to span horizontally with patterns other than running bond must include horizontal reinforcement. When using patterns other than running bond, TMS 402 requires a minimum horizontal reinforcement ratio of 0.00028 over the gross vertical cross-sectional area of the wall. The maximum spacing of this reinforcement is 48 inches. This requirement applies to all nonrunning-bond walls, regardless of whether they span horizontally or vertically, and is needed to provide continuity across the head joints. Designers may use horizontal bond beams or joint reinforcement to meet this requirement. Bond beam reinforcement should be centered in the beam or constructed with bars symmetrically placed about the wall centerline because of the potential for load reversals. The choice of using bond beams or joint reinforcement to meet this minimum requirement will depend on several factors, which are discussed in the following paragraphs.
Although the primary impact to the strength is when walls span horizontally, vertically-spanning walls are subject to a few unique requirements as well. The ability for head joints in "not laid in running bond"
patterns to transfer flexural compressive stresses under bending forces in vertically-spanning walls is limited. If horizontal bond beams are provided at 48 inches, the effective width of the compression area is the same as running bond construction (Fig. 5a), however when masonry is not laid in running bond and does not have bond beams spaced at 48 inches or less, the effective width of the compressive area is limited to the lesser of either the length of the masonry unit or the spacing between vertical bars. This requirement is especially relevant for masonry that uses joint reinforcement instead of bond beams: while all “not laid in running bond” masonry must have horizontal reinforcement, if joint reinforcement is used to meet this requirement instead of bond beams, the flexural compression zone width will be limited as indicated in Figure 5b.
Concentrated loads do not distribute across head joints in walls “not laid in running bond.” The distribution of concentrated loads through masonry walls is limited by the head joint placement, however bond beams located at the point of application can be used to distribute the load over a greater width (Fig. 6). This provision also applies to bearing calculations: the increase in bearing area for a larger supporting element is limited to an area that must be truncated by the head joints when masonry is not laid in running bond.
The in-plane shear strength of unreinforced masonry is heavily influenced by how the wall is constructed, specifically which bond pattern is used and whether the masonry units are open-ended. Open-ended units allow grout to fill the void space between cells along the length of the wall, interlocking them and limiting the influence of the weak head joints that occur between units (Fig. 7). For walls not laid in running bond, those constructed using open-ended units can realize a shear strength of at least two times the strength of walls that do not have open-ended units. Running bond patterns provide an additional increase in shear capacity, nearly four times the strength of masonry that is not laid in running bond and not constructed with open-ended units. In the 2016 edition of the code, the in-plane shear provisions did not include allowances for partially grouted walls not laid in running bond. The 2022 edition of the code provides an allowance for in-plane shear in these walls, but limits the allowable strength to 23 psi (using strength design provisions), a result of the integrity that the required prescriptive horizontal reinforcement provides. This lack of shear strength can be overcome by constructing fully-grouted walls with open-ended units, or by reinforcing the wall to invoke reinforced design provisions. The design equations for reinforced masonry do not differentiate capacities for in-plane shear based on running bond versus “not laid in running bond” patterns.
If the masonry wall is a Special Reinforced Shear wall, increased reinforcement must be provided for walls constructed with “not laid in running bond” patterns (Fig. 8). Non-participating masonry elements have similar increased requirements.
Non-structural partitions and veneer are typically subject to an even wider variety of layout patterns than structural masonry, and the unique requirements applicable to “not laid in running bond” patterns for these elements may be encountered more often.
TMS 402 requires that veneer blocks laid in other than running bond patterns have horizontal joint reinforcement of at least one W1.7 wire
spaced at a maximum of 16 inches on-center. This is equivalent to the required cross-sectional area for Concrete Masonry Units, applied over a single-wythe 4-inch nominal wall thickness.
For horizontally-spanning masonry partition walls, the prescriptive requirements of TMS Chapter 15 are not applicable to walls with “not laid in running bond” patterns. This follows the test results and provisions in the TMS that pertain to the lack of allowable flexural tension stress parallel to bed joints. If designers wish to span nonrunning-bond partition walls horizontally between supports, they must be constructed with continuously grouted sections (using open-ended units and designed as unreinforced masonry), or reinforced and designed in accordance with relevant sections of Chapters 8 and 9 of the TMS.
Bond patterns in masonry can be used to achieve a unique aesthetic and are a simple way to articulate the visual context of a building. While the structural capacity of masonry walls is typically based on a running bond pattern, other patterns may be used with some reductions in strength and careful attention to detailing. A summary of the requirements for structural masonry constructed in other than running bond patterns is as follows:
• Horizontally spanning unreinforced masonry has zero flexural tension capacity, (instead of at continuous grout sections).
• Vertically spanning unreinforced masonry is unaffected by the bond pattern.
Notes:
1) See TMS 402 for permitted stresses at continuous grouted sections.
2) See TMS 402 for grouting requirements at shear walls.
• Vertically spanning reinforced masonry must account for a reduced effective compression zone width, unless horizontal bond beams are provided and spaced at 48 inches or less.
• Concentrated loads do not disperse across head joints, but bond beams may be provided to increase the distribution width.
• In Special Reinforced Shear Walls, the spacing of horizontal and vertical reinforcement is reduced to the minimum of the following: 24 inches, one-third the wall height, or one-third the wall length, and the minimum horizontal reinforcement ratio is increased to 0.0015Ag.
• The shear strength of unreinforced masonry is reduced to 23 psi (15 psi using allowable stress), with a slightly higher stress permitted when the wall is grouted with open-ended units.
• When “not laid in running bond” patterns are used, bond beams (rather than joint reinforcement) can help overcome some of these strength reductions.
Table 1 can be used by designers as a reference for which provisions of the TMS apply to walls “not laid in running bond.” While the content of this article was developed using the provisions from the 2022 edition of the code, the relevant sections of the 2016 edition are also provided.
This article was written by Kirby Beegles, PE, SE, and edited by Owen Zukowski, EI. Graphics are by Dan Eagen. Beegles, Zukowski, and Eagen are employees of Martin/Martin Consulting Engineers, a structural and civil design firm headquartered in Lakewood, Colorado.
3) Solid gray cells indicate no changes in requirements when compared to masonry laid in running bond.
4) Unless horizontal bond beams are provided at 48” max.
MMC can raise the quantity and quality of housing available globally by offering more affordable and environmentally friendly construction alternatives, resulting in decreased costs, expedited project timeframes, and improved building quality.
By Ehsan Noroozinejad Farsangi, T.Y. Yang, Pejman Sharafi, Mohammad Noori, and Iman Hajirasouliha
The housing crisis is a multifaceted global problem that impacts millions of people. The need for affordable housing has increased dramatically due to rapid urbanization, population expansion, and economic inequities greatly beyond the ability of conventional construction methods to provide suitable and timely solutions. Lower-income and marginalized groups are mostly impacted by this crisis, which is characterized by acute shortages of housing units, rising costs, and low standards of living in many areas of the world.
To overcome these obstacles, Modern Methods of Construction (MMC) have become a viable option. MMC includes a variety of cutting-edge methods, such as prefabrication, modular building systems, and off-site construction, that increase productivity, lower costs, and produce higher-quality structures. MMC potentially provides an efficient way to significantly speed up project delivery and reduce overall costs by moving major elements of the building process from conventional on-site methods to controlled factory settings.
MMC represents a transformative approach to building, shifting many traditional construction activities to a controlled factory
environment. This raises building quality and sustainability while increasing efficiency. MMC includes methods like 2D panelized systems, in which substantial wall pieces are prefabricated and sent to the site for assembly, and 3D volumetric modular construction, in which whole rooms or portions of structures are built off-site and then transported for erection on location.
Although the idea of prefabrication dates back to the 19th century, it is currently experiencing a renaissance due to technological advances and the increased need for affordable and environmentally friendly building solutions in response to both population growth and climate change impacts. MMC applications span from large-scale commercial and institutional structures to residential dwellings. For example, due mainly to government initiatives to alleviate housing shortages and promote sustainability, MMC currently makes up a sizable fraction of new building projects in the UK.
Because of its adaptability, MMC may be used in a variety of building sizes and forms. Modular high-rise buildings in China show MMC's capacity to complete massive, intricate structures in a fraction of the time needed for conventional construction. In a similar vein, other European nations, like Germany and Sweden, have included MMC widely into their building plans, taking use of its advantages to build reasonably priced and energy-efficient houses.
The housing crisis is a global issue that can intensify social inequality and lead to increased pressure on urban infrastructure and resources. About 3 billion people, or 40% of the world's population, would require access to suitable housing by 2030, according to UN-Habitat. This tremendous demand serves as a stark reminder of the shortcomings of traditional construction techniques, which are sometimes expensive, slow, and unable to keep pace with the rate of urbanization and population growth.
Cities in North America, such as Los Angeles and Vancouver, where homelessness is on an upward trajectory and property values are surging, are severely lacking in affordable housing. The affordability dilemma for middle-class and lower-class families has been made worse by the over 30% increase in the median housing price in Los Angeles alone over the last five years. Comparable problems are being faced by European nations like Germany and the UK.
Conventional construction methods are unable to meet these demands, becoming less and less viable due to dependence on on-site labor, lengthy schedules, and vulnerability to delays and cost overruns. Building a single-family home the traditional way can take six months to a year, and unexpected problems can drive up expenses by 10 to 15%.
Furthermore, traditional building methods frequently fall short of the sustainability standards necessary to tackle climate change. About 39% of the world's carbon emissions are attributable to the construction industry, mainly because of inefficient building methods and materials. MMC and other creative ideas provide a path ahead. By employing these techniques, the building sector may get past the drawbacks of conventional methods and satisfy the pressing need for reasonably priced, environmentally friendly housing on a worldwide basis.
MMC addresses the worldwide housing issue in several ways: Cost Efficiency: The saving on building expenses is one of MMC's most important advantages. Economies of scale are possible with off-site mass production in a factory, which helps reduce labor expenses and material waste. Research from the UK suggests that MMC is a feasible choice for affordable housing projects since it may reduce building costs by up to 20% compared to traditional techniques. Additionally, significant savings can be achieved by adopting optimization techniques for the elements and connections of the modular systems.
Speed and Time Savings: MMC shortens the building schedule considerably. Projects can move on with site preparation concurrently and cut down construction time by half using prefabricating components off-site. Using modular construction techniques, a construction group in China completed the construction of a 57-story skyscraper in just 19 days, demonstrating the possibility for rapid project delivery. This speed is especially important in areas where there is an acute housing scarcity since traditional approaches are unable to quickly fulfill the demand. Quality and Precision: Building component quality and precision are improved by the factory's regulated environment. Strict quality control lowers the possibility of flaws and guarantees uniform standards for all building components. Over time, fewer expensive repairs and maintenance will be required due to the increased structural integrity and improved performance of buildings.
Environmental Impact: By consuming less energy and producing less waste material, MMC helps to make buildings more sustainable. Because prefabrication techniques use more precisely cut and assembled components than on-site construction, they produce less waste. Carbon footprints of buildings are further reduced by the adoption of energyefficient technology and sustainable materials like timber in various MMC systems. According to a Swedish research report, modular buildings have the potential to save up to 30% on operating energy costs when compared to conventional structures.
Global Success: Successful MMC deployments throughout the world have demonstrated its advantages. For example, prefabricated modular housing has drastically cut building timeframes and costs in Hong Kong's public housing developments while upholding high standards of sustainability and quality. In a similar vein, modular construction is becoming more and more common in U.S. urban redevelopment projects, where addressing the housing scarcity demands speed and efficiency.
MMC does confront a number of obstacles and hurdles. It is crucial to comprehend and tackle these challenges in order to facilitate the broad adoption of MMC technology.
Regulatory Obstacles: The regulatory landscape is a major barrier to the implementation of MMC. Zoning and building rules, which are frequently based on conventional building techniques, might make it more difficult to approve and execute MMC projects. For instance, differences in the U.S. legislative frameworks between states and localities make it challenging to implement standardized MMC procedures. It will take concerted efforts to update building rules and expedite MMC
approval procedures to address these regulatory obstacles.
Market Reluctance: Many parties involved in the construction sector, such as customers, contractors, and developers, may be reluctant to embrace new technology since they are used to conventional building techniques. This reluctance frequently stems from worries about the durability and attractiveness of prefabricated structures. These prejudices may be dispelled, and acceptance increased, by informing the market about the demonstrated advantages and achievements of MMC.
Logistical and Technical Problems: The implementation of MMC may also be hampered by logistical problems like the assembly and transportation of large modular components. Transporting prefabricated modules to the building site involves logistics that need to be carefully planned and coordinated, especially in crowded metropolitan locations. Furthermore, it might be difficult and require specialized expertise to integrate MMC with the current infrastructure and ensure compliance with conventional building processes.
Skills and Workforce: Another major obstacle is the requirement for competent personnel to manage and execute MMC technology. Retraining and upskilling the workforce is required to operate with new materials, tools, and procedures in the shift from old to contemporary building methods. To address this, funds for education and training programs must be allocated in order to create a pool of qualified experts who can spearhead the implementation of MMC.
Examples & Case Studies: Certain regions, such as Malaysia and the UK, have faced difficulties in implementing MMC. Due to logistical challenges and legislative obstacles, the incorporation of MMC into public housing developments in Malaysia has been delayed. The market's sluggish response to MMC in the UK, despite government backing, emphasizes the necessity for continued education and policy support to promote wider adoption.
Through supporting regulations and legislation, governments can play a key role in helping MMC gain traction. Removing obstacles, fostering creativity, and advancing the general use of MMC technologies in resolving the housing problem are all possible with effective legislative changes.
Supportive Policies: Governments may put in place a variety of encouraging measures, including financial incentives, streamlined regulations, and financing for R&D, to encourage the use of MMC.
Global Examples: Policies that support MMC have been effectively adopted in a few countries. A substantial percentage of public housing developments in the UK must be constructed using MMC, in accordance with the government's "Modern Methods of Construction" requirement, setting an example for other countries to follow. In Singapore, prefabricated building technologies have been widely used in both the public and commercial sectors due to the establishment of explicit norms and incentives by regulatory bodies. The development of legislative frameworks and certification processes is underway in Canada and Australia with the aim of expediting the process of scaling up to address the housing crisis.
Recommendations for Policy Makers: In order to bolster the implementation of MMC, authorities had to concentrate on a few crucial areas:
• Revising Construction Codes: Building codes should be in line with MMC technologies' capabilities and specifications in order to expedite approval procedures and guarantee that quality and safety criteria are fulfilled.
• Offering Monetary Rewards: Make it financially feasible for developers and builders to use these cutting-edge techniques by providing grants, subsidies, or tax breaks to those who do so.
Many regions worldwide have successfully implemented MMC, showcasing how these techniques may safely and efficiently meet housing demands.
• North America: Modular construction in projects like "461 Dean Street" in Brooklyn, New York, drastically saves costs and development times. With an emphasis on economy and sustainability, this 32-story structure—the highest modular construction in the world—establishes a standard for other buildings in crowded metropolitan areas.
• Australia and Asia: MMC is being included in housing initiatives in Australia. The use of prefabricated building methods has decreased labor costs and allowed for quicker project completion. La Trobe Tower is a 44 level residential tower (including plant room) in central Melbourne by developers Longriver Investments with design by architects Rothelowman. At 133 meters high, it is Australia’s tallest prefabricated building. China and Malaysia are leading the way in the adoption of MMC in Asia. The tallest modular building in the world, Mini Sky City in the Hunan province of China is one of the most ambitious modular projects to date. Built by BSB (Broad Sustainable Building), the tower block was meant to prove the efficiency and rapid nature of the modular construction process with an aim of construction a 57-story building (at 682 feet!), in less than a month.
• Europe: The use of prefabricated timber buildings in Sweden has brought about a revolution in the housing sector by expediting the manufacturing of eco-friendly and energy-efficient dwellings. The
new P18 urban quarter in Stuttgart-Bad Cannstatt is a beacon project for resource-saving and energy-efficient construction. In a very short time, the Stuttgart-based company AH AktivHaus has succeeded here in realising an exemplary project based on modules designed by Werner Sobek, which can be groundbreaking for the urgently needed paradigm shift in the building industry.Thanks to substantial government incentives and legislation, modular building is extensively employed in Germany's residential and commercial sectors. In the UK, modular lightweight steel frame (LSF) buildings are becoming increasingly popular due to their unique advantages in terms of construction speed and associated costs compared to conventional systems. Victoria Hall in Wolverhampton was constructed in 2009 and was one of the first large scale modular buildings of its kind in the United Kingdom. Designed by O’Donnell East Architects, the tower was, for a time, the world’s tallest modular building with 24-stories and standing at 251 feet.
By contrasting different implementations, optimal practices and recurring patterns become apparent. Adoption of MMCs is often successful when there is a clear legislative framework, solid government assistance, and a focus on sustainability. Sweden and Germany, for example, have included MMC in their national housing regulations and are pushing these techniques as best practices for new construction. On the other hand, locations like North America and Asia demonstrate the scalability of MMC, as they efficiently handle housing demands in both urban and rural areas via the use of modular solutions.
• Encouraging Education and Training: Fund educational initiatives to create a workforce with the necessary skills to support the shift to MMC.
• Promoting Public-Private Partnerships: To promote innovation and exchange best practices in MMC, government agencies, private developers, and industry professionals should work together. Governments can handle the housing shortage more successfully and sustainably by putting these ideas into practice and fostering an atmosphere that encourages the adoption of MMC. Enabling MMC via policy is essential to beating current obstacles and realizing the whole possibilities of these modern building practices.
MMC can dramatically raise the quantity and quality of housing available globally by offering more affordable, more rapid and environmentally friendly construction alternatives, resulting in decreased construction costs, expedited project timeframes, improved building quality, and decreased environmental effects. However, adopting MMC is not without its difficulties. Obstacles such as technical and logistical difficulties, market reluctance, regulatory constraints, and the need for a competent labor force should be addressed. Governments are essential to this process since they create laws that encourage MMC use, update building rules, and fund educational and training initiatives.
Examples of successful integration of MMC into housing designs may be found in North America, Australia, Asia, and Europe. These case studies provide insightful information and crucial lessons that may direct MMC deployments in the future throughout the globe.
Dr. Ehsan Noroozinejad is the founding director of the Resilient Structures Research Group. (ehsan.noroozinejad@westernsydney.edu.au).
Dr Tony Yang is a fellow of the Canadian Academy of Engineering, a professor at The University of British Columbia (UBC), and the founding director of UBC Smart Structures.
Associate Professor Pejman Sharafi is the head of Modular Prefab Design Laboratory (MPD-Lab) and a principal research academic in the Centre for Infrastructure Engineering (CIE) and Urban Transformation Research Centre (UTRC).
Professor Mohammad Noori is an Emeritus Professor at California Polytechnic State University and a Visiting Professor at the University of Leeds.
Professor Iman Hajirasouliha is the leader of the Earthquake Engineering Group (EEG) at the University of Sheffield.
At Vulcraft and Verco, we believe in the transformative power of partnerships. When you collaborate with our experts, you’re gaining a strategic team dedicated to your project’s success at every phase. Our innovative steel joist, deck, and grating solutions are custom engineered to maximize the value of your roof and floor systems. We can turn your vision into reality, faster and more efficiently than ever before. Let’s build something extraordinary, together. Learn more about how we can help.
A measured response based on a full understanding of cracking and deflection can help engineers and owners avoid unnecessary panic and intervention.
By Gwenyth Searer, PE, SE, Terrence Paret, Hayley Proctor, PE, and Prateek Shah, Ph.D.
Two-way, conventionally reinforced concrete slabs (herein referred to as “two-way slabs”) behave in ways that many engineers and owners may not fully anticipate (see the September issue of STRUCTURE for a detailed description of system behavior). Twoway slabs have a predictable propensity to crack and deflect. Failure to provide a measured response to the cracking and deflection can result in misunderstandings regarding the objective physical evidence and can result in unnecessary structural intervention. This article is intended to help guide engineers in their investigations and to help avoid unnecessary panic on the part of the engineer, the owner, and the public.
It is critical to recognize that both radial cracking, where cracks radiate outward from the columns, and spiderweb cracking, where the cracking looks like a spiderweb on the surface of the slab with both radial cracks and cracks interconnecting the radial cracks, are extremely common on the top surface of two-way slabs. Figure 1 shows typical radial cracking in two-way slabs; Figure 2 shows typical spiderweb cracking in two-way slabs. Such cracking typically goes unnoticed, and the structures continue to function as intended.
As described in the September issue of STRUCTURE, radial and spiderweb crack patterns are characteristic of flexural behavior. However, it is not uncommon for engineers investigating existing two-way slabs to conflate the radial and spiderweb cracks with punching
shear failures—sometimes reported as imminent and even sometimes reported as having already occurred. Indeed, a recent article by Tepke et al. in Concrete International stated, “Cracks in elevated floor slabs that radiate outward from columns combined with cracks encircling the column are likely to be an indication of a condition that could result in punching shear failure…crack patterns that indicate the possibility of punching shear failure should be regarded as an eminent [sic] risk of catastrophic failure.” This dramatic claim is contradicted by research dating as far back as the 1950s by Elstner and Hognestad, which notes that the punching shear failure surface appeared to be “completely independent of the cracks formed beforehand.” Potential punching shear failures have long been recognized to be problematic precisely because they do not manifest visible precursors prior to failure. While spiderweb cracking might be construed to encircle columns, spiderweb cracking should not be confused with imminent punching shear failure. Both radial cracking and spiderweb cracking are extremely common in two-way slabs: if these crack patterns actually represented imminent or actual failures, two-way slabs ought to be experiencing catastrophic collapses on a regular basis. The fact that they are not is consistent with research indicating that punching shear failures are unrelated to either radial or spiderweb cracking.
Designers of two-way slabs have a choice of two different compliance methods related to deflection. In ACI 318-19, designers can comply with the minimum thickness requirements provided in Table 8.3.1.1, or
they can meet the requirements of Section 8.3.2, which limits maximum calculated deflections according to Table 24.2.2. Neither option is ideal from the perspective of understanding how the slab is likely to perform in real life. Complying with the minimum thickness requirements relieves the designer from having to calculate deflection and seems like an “easy out,” but this approach can be problematic because it fails to consider important variables that affect both initial deflection and longterm, creep-related deflection such as the concrete mix design; the age and strength of the concrete at the time of formwork removal; construction sequencing loads; and restraint against shrinkage, all of which have the potential to increase the out-oflevelness of a slab.
Designers who rely on the calculated deflections of their slabs and compare these deflections to the limits in Section 8.3.2 in lieu of relying on minimum thickness may be doing so to arrive at a more economical design. Slabs designed using the calculated deflection limits in Section 8.3.2 are likely to be thinner and may deflect even more than slabs designed using the minimum prescriptive thickness limits.
Although there are four rows of requirements, two of the deflection limits in Table 24.2.2 govern many designs:
1) Where floors do not support nonstructural elements that are likely to be damaged by large deflections, the immediate deflection due to live load shall not exceed ℓ/360.
2) Where floors support nonstructural elements that are not likely to be damaged by large deflections, the deflection that occurs after attachment of nonstructural elements plus immediate deflection due to live load shall not exceed ℓ/240.
First note that “ℓ” is defined as the “span length of beam or one-way slab,” which leaves room for interpretation when the limits are applied to two-way slabs. Although a detailed analysis of this subject
is beyond the scope of this article, it could be argued that ℓ should be the larger of the length and the width of the bay; that ℓ should be the diagonal distance across the bay; or that permissible deflection should be calculated for both the long span and the short span and then added together, similar to what might be done for a steel structure with decking, beams, and girders that frame a bay, where the permissible deflections of these elements are additive. The Commentary in ACI 318 is silent in this regard.
For utilitarian structures like parking structures, there tend to be few nonstructural elements that can be damaged by large deflections, so the immediate live load condition would typically be the governing deflection limit. For cases where the ℓ/240 limit is applicable, designers are permitted to ignore the immediate dead load deflection and all of the creep-related deflection that occurs after formwork is removed but before nonstructural elements are installed.
When investigating deflections and cracking of existing two-way slabs, keep the following recommendations in mind.
Engineers can fall into the trap of considering only the negative aspects of as-built construction. For example, in-situ reinforcing steel in two-way slabs near the columns is often positioned lower than called for in the construction documents. However, construction tolerances permit some variation in position, and the phi-factors for flexural and shear strength are in part intended to accommodate lower strengths that may occur due to variations in construction consistent with practical limitations. As more information is determined about the actual as-built structure, the need for phi-factors is reduced, and their values should trend towards 1.0. Consequently, considering the effects of out-of-position reinforcing but still using standard phi-factors, while ignoring code-permitted tolerances, overly penalizes the structure.
Moreover, while it may be tempting to analyze the slab using measured reduced flexural steel depths and assume that the rest of the construction is exactly as specified, it is important to also recognize factors that may increase strength. Reinforcing
steel that is specified as having a yield stress of 60 ksi can very easily have an actual yield stress of 68 to 72 ksi. Mill certificates from the project may contain valuable data regarding the actual yield stress of the bars. Similarly, concrete rarely falls below the minimum specified strength because concrete suppliers aim above the minimum, and because concrete tends to gain strength over time. Using the actual or likely strengths of the steel and concrete typically makes more sense than using the minimum strength, particularly if phi-factors are going to be used in the analysis.
The Maximum Permissible Calculated Deflections Table Is a Design Tool, NOT an Evaluation Tool
All too often, engineers measure the outof-levelness of a slab and then compare the out-of-levelness with Table 24.2.2 from ACI 318. However, doing so is incorrect. Pure and simple, this table, which is titled, “Maximum permissible calculated deflections” (emphasis added), is a design tool, not an evaluation tool; it cannot predict what the actual fieldmeasured deflections will be or should be.
efficiency
Experience the proven performance of the 2-SEAL™ Thermal Wing Nut Anchor. Designed to seal both the air barrier and insulation, it delivers thermal efficiency, corrosion resistance, and long-lasting durability through its stainless-steel construction. Trusted by professionals for reliable, high-performance facades.
www.h-b.com | 1-800-645-0616
Nothing in either the table or the Commentary indicates that the limits in the table are intended to be used as a measuring stick to evaluate or to predict the in-field performance of a slab. Researchers have shown that deflections related to shrinkage and creep can be double that indicated by ACI 318 Table 24.2.4.1.3, “Timedependent factor for sustained loads,” as summarized in ACI 435R-20. Since the engineer designing the slab is not even required to use this table if minimum thickness requirements are met, comparing actual out-of-levelness of a slab with an optional table that shows calculated limits on deflection does not make sense. Thus, Table 24.2.2 can only be used as a design tool to help proportion slabs, not as a tool to evaluate slabs.
Removing and testing concrete cores may seem like a good way to obtain the compressive strength of the in-situ concrete; however, the coring process actually damages the concrete in the core, as documented by Bartlett and MacGregor. The core may contain microcracks that occurred naturally during routine loading of the structure or due to restraint against shrinkage or due to the coring process itself; these microcracks may weaken the core in a way that would not occur either in a cast concrete cylinder or in situ where the concrete is confined, thus resulting in an apples-to-oranges comparison between results from cores and cylinders.
In addition, the core cuts through aggregate in a way that would not occur in a cast cylinder and that does not occur in the concrete slab. This process can result in lower-thanexpected concrete core strengths that may not actually be representative of the concrete in the structure, and adjustment of the data to account for some level of damage may not fully address the actual effects of the damage. Arioz et al. found that this phenomenon can be particularly problematic with well-rounded river gravel. Cores taken from concrete with such gravel often seem to give compressive strengths lower than those from cylinders.
Caution is also warranted when using statistical methods to derive an equivalent compressive strength. In a recent investigation, 14 cores were removed from the slabs in an existing seven-story structure. The compressive strength of every core was greater than the 4,000-psi minimum required compressive strength shown on the drawings, with an average strength of 4,300 psi and an impressively low standard deviation of only 110 psi. Yet when the investigator used ACI 562-16 to derive an equivalent compressive strength of the concrete, the procedure yielded an equivalent design strength of only 3,670 psi, a result that does not appear appropriate when looking at the data.
Surveying the elevation of the top surface of the slab and then comparing it to the elevation of the slab at the supporting columns and walls does not yield a measure of deflection. The elevation of the top of the slab has permissible construction tolerances; variations in flatness, levelness, slab thickness, and support elevations are also permitted, as are deflections of freshly placed plastic concrete that occurs while formwork is supported on suspended slabs. Quite simply, although deflection will always contribute to out-of-levelness of a slab bay, measured out-of-levelness represents only that, and not deflection. Finally, it is always wrong to include elevation data from distant bays in the computation of out-oflevelness of a slab within a given bay.
Camber is often omitted during
construction, even when it is specified in structural drawings. While camber can be used to offset some of the immediate deflection that occurs upon formwork removal, it requires the contractor to create difficult-to-fabricate vertically undulating formwork, complicates layout of reinforcing, and introduces labor-intensive complications during placement and finishing operations. As a result, it is quite common for camber to get value-engineered out of the construction, for the concrete subcontractor to decide to omit the camber on their own, or for the detail to be missed by the construction team during the chaos of construction. During an investigation of a slab that was specified to have camber, it is important to recognize that the camber may not have been installed, which may partially explain why measured out-of-levelness is larger than expected.
Even if camber is installed, it cannot solve all deflection issues. Engineers, architects, and owners often do not understand what camber can and cannot do. A slab that is exposed to water and that was constructed without an overall slope-to-drain will develop mid-bay puddles at the low points of the deflected shape, cambered or not. If excessive camber is specified and slab deflection does not overcome the camber, the slab will slope towards the column lines, and water will collect there. If only moderate or minimal camber is specified and slab deflection is greater than the camber, the slab will still tend to slope towards the centers of the bays, and water will collect there. Moreover, because deflection is time- and load-sensitive, slab drainage characteristics change over time. The only way to reliably avoid puddles in a twoway slab that is exposed to water is to provide sufficient overall slope in the slab that exceeds the local slope caused by deflection so that water will drain to one edge or point, or to install drains at the low point of every bay. Figure 3 shows an aerial photo of a parking structure; the presence of the puddles does not indicate that the slab is performing poorly; rather, the puddles indicate that drains should have been designed and installed in the middle of every bay but were not. Similarly, if designers do not want the water in those puddles draining through the slab, a traffic coating should be specified. The fact that water will travel through the inevitable cracks in a concrete slab should not be taken as convincing evidence that the slab is performing poorly.
One of the most important keys to investigating existing structures, including two-way slabs, is to follow the objective physical evidence. Just because someone noticed cracking recently does not mean that the cracking occurred recently. There is often evidence that the cracking has existed in its current state for a long time and thus may be less of a cause for concern than if it just occurred. The presence of paint,
soot, dirt, patching compound, prior repairs, and carpet glue within a crack all indicate that the crack was present at the time the material was applied over or accumulated in the crack. Figure 4 shows an example of paint within a crack that can be used to estimate the age of the crack.
Similarly, take care not to overestimate the width of cracks, particularly when the edges of the cracks are rounded or raveled. It is common for the edges of cracks to spall and become rounded over time when subjected to vehicular and even pedestrian traffic. Surface preparation like bead-blasting prior to installation of finishes, or to remove finishes, can also make a dramatic difference in the appearance of cracks (Fig. 5). Since the purpose of measuring crack widths is generally to understand their structural relevance, it is the crack width at-depth that is most relevant, not the crack width at the surface.
It is easy to get wildly off track with respect to investigation of two-way slabs. Nearly all two-way slabs have performed as designed, particularly given the many decades of documented performance as well as laboratory testing. Engineers should ensure that they do not overreact upon discovery of radial or spiderweb cracking or upon determining that the out-of-levelness of a slab is greater than ACI design limits for calculated deflection. Emergency shoring is rarely required, and expensive repairs and retrofits should generally be avoided unless there is a significant risk to life safety.
Engineering software offers expansive capabilities when used with thorough and grounded engineering practice.
By Angelina V. Stasulis, PE, SE
If you can't understand how the software designs this:
In Structural Engineering, structural analytical modeling software provides unparalleled efficiency. These software programs are celebrated for their ability to streamline calculations, craft intricate structural models, and expedite the design process. They empower engineers to tackle challenges with precision and creativity, embodying the transformative potential of technological advancement in the field.
Designed to be user-friendly, modeling programs often provide answers with ease, generating loads and auto-sizing complete structural skeletons in minutes. Their speed with calculations can be an allure to overburdened engineers facing tight deadlines. Their perceived power and accuracy can lull the most experienced into complacency. Without an impetus to question either the validity or suitability of the results, they often rely on default settings without reviewing documentation on what these settings are and how to change them. There’s a software term called “garbage in, garbage out.” If the inputs to the model are wrong, incomplete, or inaccurate, the output, no matter how favorable, is also useless. Thus, while software offers unprecedented capabilities, it is imperative for engineers to exercise caution and maintain a balance between technological convenience and thoughtful,
rigorous engineering practice.
With a little extra care and attention, engineers can develop structural modeling skills and managers can incorporate QA/QC practices that enable firms to successfully leverage the power of analytical modeling software.
The first, and likely largest, problem is that new software users often lack critical understanding of their objective. Often these users are new engineers, accustomed to solving well-defined textbook problem statements. While these simple scenarios are ideal for mastering individual concepts, they fail to develop big picture thinking required for our real-world tasks.
It is imperative that engineer make an explicit effort to maintain “responsible charge” of their software and treat it as something that requires specific instructions and oversight, rather than something that already knows it all/ knows better than you.
Structural Engineers are required not only to execute a collection of individual design actions, but also generate and apply loadings, identify limit states and code requirements, and consider how multiple conditions influence one another.
University Problem Statement Real World Problem Statement
"Check whether the section is adequate."
"Design the building."
Incorrect/incomplete loadings
Failure to verify applicability of program's default assumptions
Failure to understand global/local coordinate axes
Failure to provide complete lateral load path
Failure to consider diaphragm steps and/or discontinuities
Inconsistent application of load factors/combinations
Incorrect understanding of program output
Incorrect member boundary conditions
This big picture understanding of how to apply the tools from our college education to real-world problems is a giant gaping hole in our current education system.
Armed with software that enables you to design to a specific iteration of the building code, it can be tempting to input your geometry and press “go.” Over my career, I’ve observed a multitude of errors in analytical models, some of which are listed in Table 1. These errors are largely a result of three main things: not being aware of software limitations, not understanding processes, and not performing “sanity checks.”
Structural analytical models are good at “plugging and chugging,” performing repetitive calculations or multi-part problems that rely on the output from one part as the input to the next. Software is designed to execute pre-written processes based on provided input, much like a calculator can perform specific operations following order of operations rules, but doesn’t know when you meant to type a negative sign instead of a “minus.”
A few examples of such operations and sample processes for Structural Analytical Modeling Software are:
• Distribute vertical floor pressures to beams, when provided with direction on distribution (one-way, two-way, etc.).
• Transfer reactions from beams onto supporting members, when provided with appropriate degree of freedom conditions at the joint.
• Calculate lateral forces, when provided with parameters and geometry.
• Distribute lateral forces to lateral force resisting system elements, when provided with diaphragm behavior and element location, identification, and relative stiffness.
• Compute member capacities, when provided with appropriate bracing conditions, material strengths, etc.
• Compute member demands, when provided with limit states to consider.
• Apply load factors, when provided with direction on how to combine and factor different load cases.
• Compute drift and deflection values, when provided with load conditions and desired locations.
Software is not good at making decisions. While you might select a building code to design to in the software input screen, Too often,
Structural Engineers assume their analytical modeling software does all of this for them. After all, if you tell the program to design based on the IBC 2021, it’s an easy leap of faith to assume the program is going to identify and apply all provisions and referenced standards from the IBC 2021. What you’re telling the program is just which versions of equations to use in its limited pre-defined workflows. By blindly assuming the default settings are appropriate and that all required checks are being performed by the software, you are devaluing yourself, your skills, and abdicating the control and responsibility our profession is charged with. It is imperative that engineers make an explicit effort to maintain “responsible charge” of their software and treat it as something that requires specific instructions and oversight, rather than something that already knows it all/knows better than you.
Before asking software to do something for you, it's essential to grasp how the task would be performed manually. This step is often the key part of effective software use training, yet it's frequently overlooked. I’m not advocating for a return to the days of mastering moment distribution or performing finite element analysis by hand. Instead, the emphasis lies in understanding the pertinent code requirements for your design and identifying which limit states are likely to govern your approach. What decisions must you make, or what information must you possess to navigate through these code requirements? Consider sizing a steel column. You'd require both the demand and capacity for all relevant limit states. Begin by referencing the building code to determine the applicable limit states. To establish the demands, you'd need details such as the tributary area, applied loads, connections to other members, and load combinations. On the other side, determining the capacity requires factors like overall height and bracing conditions. These elements serve as your inputs. The code or specification guides you towards the desired outputs. Understanding this input-to-output transformation grants you insight into what should transpire within the "black box." This enables you to focus on providing the correct inputs, and understanding how any generalizations or assumptions in those inputs might affect your output.
Next, you need to understand how the software is approaching the problem. This is frequently excluded from Quick Start tutorials, which focus more on the clicks and menu options to go through in order to provide the program with input, not on how the program uses that
input. However, developers do frequently provide documentation on the assumptions, limitations, and processes inherent in their products through Help file documentation, online forums, and Wikis. Without knowing that effective length is important to column design, you might not think to ask how the program calculates a column’s effective length, or look into whether you can model a single column through multiple stories and expect the program “knows” it’s braced at each level or should consider its full node to node length.
If you don’t have an idea of what the “right” answer should be, you are putting too much trust in the computer. Too often, engineers fall into the trap of blindly running models until they yield a passing result, relinquishing their role to the software.
The computer is not smarter than you. Many times we rely on “experience” from PMs/PICs, but taking ownership for your own model can help develop that experience ahead of time. Human beings learn from experience. We train ourselves to expect certain results based on previous experience, so, for newer engineers, without experience, it can be easy to abdicate responsibility, saying there’s no way for them to know what to expect. That’s not accurate.
In lieu of experience, we can leverage critical thought about what controls what. It doesn’t take ten years to understand that you don’t need to compute snow drifts if there’s no way for them to govern over your roof live load, nor do you need to ask your 30-year veteran boss if an HSS4x4 column makes sense for your 10-story building when
• Handouts from Related Presentation, Black Box Magic: The Logic Behind Structural Modeling, by author at 2022 NCSEA Summit, available at www.structure.org.
• CASE Tool 5-3: Managing the Use of Computer Software in the Structural Engineering Office: www.acec. org/resource/case-tool-5-3-managing-the-use-ofcomputer-software-in-the-structural-engineering-office
• STRUCTURE Magazine Article "Simple Capacity Checks for Commonly Used Steel Sections" by Hee Yang Ng from April 2022: www.structuremag.org/article/ simple-capacity-checks-for-commonly-used-steelsections/
• How Structural Engineers Find Errors in Analysis and Design Results. Practice Periodical on Structural Design and Construction by James Hanson, Rose-Hulman Institute of Technology, 2021: scholar.rose-hulman.edu/ cgi/viewcontent.cgi?article=1179&context=civil_fac
you can perform your own load take down by hand for its 30x30 tributary area and check it against the AISC table. Thinking through how you could do some things by hand, and doing them, will help build that frame of reference for what a reasonable design might be, as can looking at past designs for similar projects. When in doubt, ask someone else how they would “back of the envelope” check something.
Regardless of the software used, engineers bear the ultimate responsibility for ensuring code compliance and accuracy in their designs. Dependence on others to complete tasks undermines this responsibility and can lead to errors. Taking charge of our work is not only professional but essential for ensuring the integrity and safety of structural designs.
Read the Building Code
It sounds so basic, but one of the best things we can do for our staff is to point them towards actual sections of the code and ensure they understand what the legal drivers for our work and the acceptance criteria are.
As an individual, the best way to determine your objectives is simply to read through the building code itself. Familiarize yourself with the different triggers and clauses that govern design and might add additional criteria. We bear the responsibility for their accurate execution. Defining the scope is paramount, particularly for newer engineers. Throughout my career, I've found that most errors come from lack of awareness. This underscores the importance of recognizing that no software program can cover every aspect of our work comprehensively. Acknowledging code requirements and project objectives enables us to understand where specific software capabilities can take up some of the load while maintaining control of the big picture ourselves.
Structural Engineers need to have an intuitive understanding of how structures behave and respond under various conditions. At its core, our primary role is to ensure the stability and load path of a structure. It's fascinating to note that even preschoolers grasp basic physics concepts intuitively, recognizing that a cereal box will tip when loaded at the top, but slide when loaded near the bottom. To foster this innate
understanding, structural engineers can utilize hands-on manipulatives like Play-Doh, paper, or spring models as tools in our training and practice. Everything we do in structural engineering is rooted in intuition, but complexity arises when we're juggling multiple factors for different load conditions simultaneously. Cultivating this intuitive sense of structural behavior, and leveraging it during design, is not only essential but also enriching.
In the realm of structural engineering, the importance of verifying the accuracy of models cannot be overstated. Attempting to verify a large and complex model without having first mastered a simple one is a recipe for potential errors and oversights. Engineers must adopt a methodical approach, especially when learning new software programs. In Software Engineering, there’s a concept known as “unit tests.” Unit tests are small tests designed to verify the correctness of individual units of code, such as functions or methods. They are written and executed by developers to ensure that each component of the software behaves as expected under various conditions and serve as a vital part of the QA/QC and provide documentation on how different parts of the software function.
Structural Engineers can mimic this technique by starting with a straightforward model that can be fully analyzed and verified by hand. Simple models offer the perfect opportunity to explore how the software distributes loads, generates wind and seismic loads, asserts fixity and boundary conditions, chooses unbraced lengths, applies load combinations to load cases, and presents results or reactions. By thoroughly understanding these fundamental aspects and being able to confidently and efficiently verify how the software is using input and generating output, engineers can confidently tackle more complex models, ensuring their accuracy and reliability in structural analysis and design.
Recommendation:
• Model a simple span beam to understand load combinations and releases.
• Model a four-post frame to explore lateral design.
The role of supervisors and managers in the development of their staff cannot be overstated. While acknowledging the challenges they
face—often ill-equipped to teach, with their focus squarely on deadlines and deliverables—it remains imperative for them to take an active role in cultivating the next generation of engineers. This responsibility falls on those who are “in responsible charge.” Without adequate mentoring, staff members are set up for potential failure. Supervisors must go beyond drawing reviews that merely check for reasonable sizes; they must hold their staff accountable for their methods and approaches. It's crucial to understand that getting the right answer through the wrong means is still incorrect. Engineers should feel a sense of ownership and responsibility for their designs, driven by the understanding that they are ultimately accountable for their work. Employers can support this by providing self-check tools, implementing robust QA/QC procedures and encouraging hand calculations. Most importantly, they should refrain from placing staff in situations where they feel compelled to rely on models before they're fully prepared. This proactive approach ensures not only the professional growth of staff but also the integrity and quality of the work produced.
Recommendations:
• Have staff explain their thinking and site code references before they start.
• Require staff to fill out some sort of hand-check form showing what they’re inputting, how, and what the answer is expected to be versus what it is.
Structural Modeling can be an incredibly useful tool in our toolbox, but requires an intentional approach from engineers as employees and employers. It’s critical for us to use these tools of responsibility rather than defer decisions and design to software, killing our value as a profession before any Artificial Intelligence program even has to fight us to do it. Fortunately, each of us has the ability to learn and fix this. As Spiderman's Uncle Ben (who actually quoted Voltaire) aptly noted, "With great power, comes great responsibility," a sentiment that underscores the immense capabilities and impact of modern structural engineering software that already exists, and all that is to come as we move into the age of Artificial Intelligence.
Angelina V. Stasulis, PE, SE started college as an Education major, intending to become a high school teacher. While she eventually changed majors and has been a consulting Structural Engineer since 2009, her love for teaching continues to drive everything she does. As a member of NCSEA's Board of Directors, Senior Structural Engineer for Shear Structural in Atlanta, GA, and parent, she constantly seeks out ways to leverage her passion for education.
The service life of a 135-year-old stone arch bridge was extended with a structural repair project that entailed a new reinforced concrete deck, cantilevered sidewalk, and stone masonry repair.
By Carlo Citto, PE, SE
Owner: City of Elkader
Project Engineer: Origin Design
Masonry Engineer: Atkinson-Noland & Associates
Prime Contractors: CJ Moyna and Sons and Taylor Construction Inc.
The iconic Keystone Bridge in Elkader, Iowa, was built in 1889 to provide the city with a long-lasting river crossing solution (Fig. 1). Before its construction, several timber and iron bridges were built to cross the Turkey River, but they all deteriorated rather quickly and were found structurally unsafe within a decade of their construction. In 1888, the city decided to replace the latest iron truss bridge with a more durable stone arch bridge. The historic bridge, one of the largest and best constructed twin arched stone bridges in the Midwest United States, has seen numerous repair campaigns over time but continuing material deterioration and the appearance of longitudinal cracks in each of the two arches prompted a low load posting of 5 tons. In 2015, the City of Elkader initiated plans for what was set to be the most extensive rehabilitation of the bridge since its construction in 1889. The city identified the AASHTO HS20 truck and the Iowa DOT legal vehicles as the target live loads for the rehabilitation. Construction started in April 2022; the structural repair scope of work included a new cast-in-place reinforced concrete deck, a new cantilever sidewalk attached to one of the spandrel walls, pinning of the spandrel walls, and stone masonry repair and strengthening to increase the longevity and load carrying capacity of the bridge to meet current design loads.
The Keystone Bridge’s location was particularly suitable for the use of stone masonry due to the presence of shallow, competent bedrock and a nearby quarry to source native limestone. Bids were requested, however, for the construction of either an iron bridge or a stone bridge: it turned out that the construction of an iron bridge was more expensive than a stone bridge with the same width, and the construction of the stone bridge was awarded for $13,000. The design by Mr. Tschirgi called for a double arch structure, 204 feet in length and 30 feet wide with a 6-foot sidewalk on the interior of the bridge. Each arch is 3 feet in thickness at the crown section and spans approximately 84 feet with a clear height of 28 feet. When the Keystone Bridge was constructed, it was described as “the longest and best constructed highway bridge west of the Mississippi River and east of the Rocky Mountains” (Engineering News 1891).
Construction of the bridge started in September 1888. The foundation was laid directly upon the native bedrock and filled with hydraulic cement grout. The centering—the temporary wooden structure used to support the arch during construction—consisted of only five frames, likely spaced at 7.5 feet on center, covered with closely spaced boards or planks upon which the stone units were laid (Fig. 2). About 50,000 feet of lumber were used for the centering and about 4,160 cubic yards of materials were used for the construction of the bridge. The weight of the bridge was estimated at more than 18,000,000 pounds. Although the bridge was originally expected to be completed in December 1888, it took until August of 1889 to finish the work. The total construction cost of the Keystone Bridge was reportedly around $16,300, equivalent to approximately $550,000 in today’s value.
Details of the bridge design became available when Mr. Tschirgi presented a paper to the Iowa Civil Engineers and Surveyors’ Association in 1889. With the horizontal thrust at the arch crown section estimated at 65,000 pounds and an assumed allowable compressive strength of 150 psi, the depth of the keystone, which is the wedge-shaped stone located at the apex of the arch, was calculated at 3 feet. This value is consistent with typical empirical rules available at the time of construction to define the thickness of the keystone.
Notably, the old iron bridge being replaced was reportedly kept open during construction to provide a convenient river crossing even though the new bridge was being erected in the same place as the old bridge. Evidence of this construction sequence is found in a historic photo showing the Keystone Bridge under construction: the deck and piers of the old bridge are still visible in the background (Fig. 2). The simultaneous construction of both arches was not possible due to the presence of the old bridge piers. This resulted in some concerns about the stability of a single arch and whether there was enough frictional resistance under the center pier to resist the horizontal thrust in the arch, with calculations showing only a safety factor of 1.8. Fortunately, construction was completed without problems and the bridge has served the City of Elkader ever since. Because of its historical significance, the bridge was listed on the National Register of Historic Places in 1976.
The evaluation process for the bridge’s rehabilitation began with the review of existing documents related to its construction and past repair campaigns to gather information in support of the structural analysis. The design team conducted a visual condition survey and found the stone masonry to be in fair condition overall, with localized areas of
cracks and deteriorated limestone. Surface cracking and spalling of the limestone were visible along the south face of both arches, most likely due to freeze-thaw cycles. Longitudinal cracks were observed on the underside of the arches, with the condition most severe near the arch midspan. The presence of water stains at the underside of the bridge was an indication of water migrating from the top of roadway surface down into the arches, providing an active source of moisture for damaging freeze-thaw cycles and material deterioration. The design team planned to conduct a thorough condition assessment of the bridge, including surface penetrating radar (SPR) investigation of the spandrel walls and arches to evaluate typical stone thickness and solidity of the stone masonry construction. Typically, the arch thickness, a key factor in the determination of the structural capacity of an arch bridge, can be measured at the spandrel walls. Radar scanning is used not only to confirm that the arch barrels have the same thickness away from the spandrels but also to evaluate any loss in cross-section due to material deterioration that could adversely impact the structural capacity of the arches. Results from such investigation provide valuable information to support the structural analysis of the bridge and reduce conservative assumptions. However, due to the low load posting of the bridge, the team faced significant limitations in accessing the bridge with an underbridge inspection vehicle and was unable to position the vehicle effectively to conduct a detailed evaluation of the bridge. Without a close evaluation, the full extent of the stone deterioration could not be fully captured. In one specific instance, the severity of deterioration that was uncovered during the stone repair work warranted the use of temporary shoring (Fig. 3). The shoring was put in place to provide support to the arch, allowing the team to safely replace six stone units that were structurally unsound and separated from the arch. The units were located at the south face of the arch, between the quarter point and the crown sections, and replaced with stone sourced locally. The shoring consisted of two triangular steel frames with the addition of a curved section on top to follow the arch profile. This solution closely resembled the wood centering used in the original construction of the bridge.
Stone arch bridges exhibit complex structural behavior under gravity and vehicle loads. Often, the inherent strength of the original materials and design is diminished by conservative assumptions made to simplify
the structural analysis. Less conservative results and load ratings can be obtained with a 3D finite element analysis (FEA) capable of capturing more realistic load and stress distributions within the structure. The main drawback of FEA is the higher modeling and computational costs. Considering the historic significance of the bridge, a full 3D analysis of the structure was seen as the most appropriate analysis method.
Atkinson-Noland created the computer model of the bridge in Midas FEA (Fig. 4). Continuum elements were used to model the arches, piers, spandrel walls, soil, and concrete deck. The stone masonry was modeled as a homogeneous material, without distinction between mortar joints and stone units. To account for the composite action, material properties representative of the mortar-stone assemblage were assigned to the masonry and selected based on typical published values for similar materials. The load rating analysis was conducted following the AASHTO Manual for Bridge Evaluation and only dead and live loads were considered. The analysis incrementally moved live loads along each design lane in 3.5-foot increments to find the maximum internal forces at critical arch sections (i.e., crown, quarter points, and springlines) to be used in the rating calculations.
Atkinson-Noland calculated rating factors based on an evaluation of maximum tensile and compressive stresses developing in the arches in the longitudinal and transverse directions. To account for uncertainty in the collection of as-built data and other unknowns, a knowledge factor, l, equal to 0.9 was used to adjust the bridge capacity. In calculating component capacities, a knowledge factor is used to express the confidence with which the properties of a structure or structural components and the presence and extent of damage are known. Engineering judgment is used to establish the knowledge factor value from the information obtained from original construction documents and/or condition assessments, including destructive and nondestructive testing of representative components. Atkinson-Noland also used the finite element model to evaluate the construction sequence and construction loads for potential overloading of the arches as a result of an unbalanced load condition or localized stress concentrations from construction vehicles (Fig. 5). An analysis was also carried out to provide design loads for the temporary shoring needed to support the arches during the replacement of full stone units. Shoring an arch bridge requires support in both horizontal and vertical directions at the bracing points. The 3D model of the bridge was used to evaluate the shoring approach, provide shoring loads, and help optimize the shoring design to mitigate additional construction costs.
The rehabilitation work included repointing of all deteriorated mortar joints with compatible mortar. Damaged and deteriorated stone, such as spalled and cracked units, were repaired with dutchmen, where deteriorated material is removed and a new stone keyed into the existing masonry and secured with stainless steel pins designed to facilitate mechanical locking and prevent possible slippage. Because the bridge is listed on the National Register of Historic Places, the rehabilitation plan followed the principles of minimal intervention as defined in the Secretary of the Interior’s Standards for the Treatment of Historic Properties. The compatibility of the replacement stone was evaluated through axial compression and absorption tests carried out by the masonry contractor. Atkinson-Noland conducted mortar analysis to identify binder to aggregate ratio, aggregate color, and aggregate size gradation to provide a compatible replacement mortar formulation. The longitudinal cracks in the arch rings below the spandrels were repaired by installing stainless steel anchors grouted in core holes drilled from the face of the arch ring and extending several feet into the arch. The end piece (or plug) from cores was retained and reinstalled with restoration mortar matching the color of the surrounding limestone to cover the anchor at the face of the wall and conceal the application. The anchors were also designed to enhance the flexural tensile capacity of the arches in the transverse direction. The historic Keystone Bridge is an iconic structure at the heart of the City of Elkader’s historic district. After a successful rehabilitation project, the 1889 bridge was repaired and strengthened to increase its load carrying capacity to meet AASHTO and Iowa DOT load requirements and will continue to serve the city for many years to come. The bridge was rededicated in 2024—the 135th anniversary of its original construction—and the rehabilitation project was awarded the 2024 Excellence in Archaeology and Historic Preservation Award by the State Historical Society of Iowa Board of Trustees.
Full references are included in the online version of the article at STRUCTUREmag.org .
Carlo Citto, PE, SE, is a Structural Engineer and Principal at Atkinson-Noland & Associates. He has over 15 years of experience in structural analysis and load rating of stone masonry arch bridges and has been involved in the rehabilitation of many historic arch bridges throughout the country. He can be reached at (ccitto@ana-usa. com).
A plug-and-play structural bridge replacement meets the needs of fastpaced industrial and consumer demands.
By Ken Kennardi, PE; Scott A. Collins, PE, SE; and Ann L. Collins, PhD
Astructural engineer typically designs new structures or renovations to existing structures. This involves consideration, not only of the stability but the economy of building the structure. However, when disaster hits, the things to consider become more complex. Safety concerns arising from the damaged structure itself and the economic impact of business interruptions have to be added into the already complex equation of safety and economics. What happens when accidents happen to a manufacturing facility? Now, the engineer must worry about the economics of a manufacturing shutdown, often of much higher losses than the repairs themselves.
The owner of a manufacturing facility, NC Moulding, in North Carolina experienced these losses and concerns firsthand when a dumpster truck struck and damaged part of their facility. In August 2023, a truck carrying dumpsters left its back (tilting-frame) lifted and struck a 2nd-floor pedestrian bridge that provided an essential path for product transport and storage between two manufacturing stages located in neighboring buildings. The impact destabilized the bridge by deforming a lower chord
on the bridge structure and partially rotating the trussed bridge (Fig. 1). This also displaced the dust collector system equipment, important for reducing breathing and fire hazards, due to the damage to the chord that supported them. The damage created a significant safety hazard, thus shutting down the bridge. This crippled the manufacturing facility, disrupted traffic through and below the bridge, and disrupted the use of the dust collectors. The stairwells were too narrow to allow efficient travel of the product to street level and back up and therefore alternative routes were not feasible. Upcoming on their peak season through December 2023, the immediate losses were building up. Without a quick solution, a prolonged shutdown would exacerbate these losses and would begin to affect employee livelihood and retention as well as relationships with key customers, potentially leading to damage to the business’s long-term stability.
Given the severe consequences of delay, the Owner and insurance representatives brought in disaster recovery specialists, including Collins Structural Consulting, PLLC (CSC) as the structural specialist, to figure
out how to get the plant up and running quickly and safely, with the ultimate goal of a complete repair and as few losses to the business as possible. When CSC arrived on site, it was quickly determined that the bridge, structurally, needed a complete replacement. However, the typical time frame for replacing an enclosed pedestrian bridge easily includes several months of construction, not counting the design and planning phases. Therefore, CSC recommended a temporary reinforcement of the existing bridge which could allow production while the long-term solution was generated.
CSC focused the temporary design on providing additional support at the point of impact (Fig. 2) near the bridge’s midspan, using shoring posts anchored to shallow concrete footings near the bridge’s midspan and shoring posts connected to longitudinal beams that were momentconnected to the intermediate bridge girders. However, the nature of the manufacturing plant meant that flammable, chemical-treated wood dust blanketed the site; therefore, field welding was not a safe option. Yet the tight overhead clearances also meant that standard bolted connections were not feasible. Therefore, CSC incorporated an innovative product, Shuriken nut covers (Fig. 2), into the design. This product was designed to allow welding without loss of strength. In this case, the nuts were welded onto plates offsite, which fixed the nut from rotating, allowing bolts to be installed in tight spaces where wrenches do not fit onto the nut side of the bolt. Because CSC designed the structure from field measurements, despite that complex geometry and deformed structure from impact, CSC incorporated Lindapter girder clamps onto locations where lack of exact measurement would impact the ability of the bolts to align. These clamps connect to the flange of a beam instead of requiring an exact bolt-hole location. This temporary repair design allowed a quick return of the bridge’s functions to provide transport across the bridge, safe passage for traffic below the bridge, and stable support to at least one of the dust collectors.
Once the temporary repairs were in place and the plant had returned operational (albeit at a slightly lower pace because the bridge could not be used to store product), the problem still required a permanent solution to replace the bridge without a long plant shutdown. Knowing that the plant would have a scheduled two-week shutdown over the last two weeks of December, the disaster recovery team had approximately three months to develop a plan, have all the parts and pieces ready for installation, and come as close to a two-week install as possible. Meeting these goals depended on the accuracy and precision of the survey, measurements, and fabrication, decreasing manufacturing and installation times, and incorporating preassembly before the facility shutdown.
The first step, before design or construction, was to ensure the information on the existing buildings, bridge, and support equipment was as complete as possible. This was complicated by the complex geometry generated due to the differential heights and skewed angles of the existing
buildings and their openings for the bridge. The survey team used a Leica BLK 360 scanner to produce a thorough and accurate three-dimensional scan of the area. The scanner had an enclosed LiDAR sensor that captured the data on the surrounding environment at 680,000 points per second to produce accurate 3D scans. The surveyor recorded data points from six locations: the inside of the bridge at each end, the inside of the bridge at midspan, and the outside of the bridge at each of the four corners from the ground level. The resulting data produced a clear image of the bridge's structure, including not only the dimensions of the bridge at all locations and distances between the two buildings but also the degree of damage (Fig. 2). This included a rotation of the bridge structure due to the impact, with a full nine inches of relative displacement to the bottom chords, as compared to the top chords. It also showed the installation locations of existing mechanical, electrical, and dust collector equipment within and outside the bridge. The design and construction teams quickly shifted into design mode, knowing the design must prioritize efficiency and reduction of construction errors that would affect speed or quality.
The bridge’s structural design consisted of an upper span of the bridge built from two Warren trusses with alternating compression and tension diagonal members supported by an A-frame design with four new columns and footings, two located at each end of the bridge, adjacent to the respective buildings. The 3D scan depicted the skew between the two buildings which do not run parallel to each other, producing one truss length spanning 51 feet, 3 inches and the other at 62 feet, 2 inches. The truss’s vertical and diagonal members were designed as Hollow-StructuralSteel (HSS) tubes shop-welded onto the upper and lower chords of the truss. The floor beams, roof beams, upper chords, and lower chords are all W-shaped steel beams that were mostly detailed as bolted connections, allowing for full shop fabrication of the two trusses and therefore, quicker field installation.
CSC designed the bridge such that the structural steel and light gauge steel-framed walls could be preassembled as three main pieces: two support pieces (A-frames consisting of the columns and cross beams to be installed onto the footings) and the full elevated length of the trussed bridge (to be placed onto the two A-frames at each end). This design, which creates a bridge structurally independent of the adjacent buildings, was selected to eliminate uncertainty of the unexposed structural elements of the existing building and to decrease the assembly time required during the plant shutdown. Not including the existing building as part of the bridge’s structure eliminated the chance of discovering existing issues within the building that would have required repairs or corrections during installation. Also, a bridge bearing on the existing structures would need to be longer than the space between the buildings to reach the support beams in the existing walls, eliminating the ability to maneuver the full span of the bridge into place after assembly. By designing new supports and ensuring the full span of the bridge was slightly shorter than the clear span between the buildings, the full span of the bridge could be assembled in a nearby lot before the shutdown and lifted into place as one piece, decreasing assembly time needed during the plant shutdown. This design, however, created additional design considerations, as the structure had to meet requirements for two load-path design scenarios: 1) the construction loads as the pieces were being lifted into place suspended by the crane and 2) the in-place, in-use loads of the pieces, each being supported on the new columns and supporting the main gravity live and dead loads of the bridge.
The structural team worked collaboratively with the steel fabricator and contractors to ensure that each decision in the design did not slow down the process. CSC consulted with North State Steel (NSS), an experienced fabricator for time-sensitive projects, and generated iterations of connection designs to be used only for connections with a quick fabrication process in the final design. NSS used imported Revit drawings directly
from CSC to ensure accurate shop drawings. This close collaboration allowed for the swift generation and approval of the drawings. CSC also consulted with the contractor (Belfor Property Restoration) to ensure the design incorporated high-early-strength concrete that would not require extended cure times.
The two-week shutdown was scheduled for December 21, 2023, to January 2, 2024. So, when the manufacturing workers started their break, the disaster-recovery teams picked up the pace and initiated the installation. All shop-fabricated steel elements of the bridge arrived on site on December 18, 2023, three days before the shutdown, to begin pre-assembly in the site’s parking lot. Demolition of the existing bridge began as soon as the shutdown allowed. The demolition was completed in five days, allowing for the pouring of the concrete footings on December 26, 2023. The high-early-strength concrete allowed the footings to be ready for loads the next day. With five days left before the manufacturing workers returned, the footings and the pre-assembled bridge were ready for installation on the morning of December 29, 2023 (Fig. 3). With members of the construction and design teams on site to ensure accuracy and speed of problem-solving, the construction team installed the A-framebridge support frames onto the footings and rigged, lifted, and bolted the full elevated length of the trussed bridge into place. The team’s preparation paved the way for the installation of the main structure in just a few hours. This left more than four days for the mechanical and equipment contractors to reconnect existing equipment onto the bridge structure, which was guided by the detailed scans of the original placement. While some architectural and finish work was performed after the workers returned, full operation of the bridge and therefore the manufacturing facility was returned in less than the aimed two weeks, allowing the manufacturing plant to reopen on schedule on January 2, 2024 (Fig. 4).
The team used intense collaboration throughout the design and build process, an integral piece to achieving the speed required for success on this repair and replacement project. The owner's needs drove the design process and dictated the construction schedule. By prioritizing this speed, the design team managed to protect the livelihood of the business and the employees while preventing additional losses from the owner and insurance company. The need to put the manufacturer back into production quickly to avoid loss of business meant shifting design economics from the standards used for new construction to a focus on reducing downtime. By providing quick temporary repairs to allow the business to function until a scheduled shutdown and using the intervening time to carefully plan and pre-assemble as much as possible, the demolition and construction of the permanent repair required less than the two weeks of the scheduled shutdown time, thus achieving the goal of repairing the damage with as few disruptions to the owner and their employees as possible. ■
Ken Kennardi, PE, is a structural engineer for CSC working out of Durham, North Carolina. (kkennardi@collinsstructural.com)
Scott A Collins, PE, SE, is the principal engineer for CSC and a Structural Specialist for the NC Emergency Management teams. (scollins@collinsstructural.com)
Ann L Collins, Ph.D, is the Director of Finance for CSC and serves as the primary technical writer for the company. (acollins@collinsstructural.com)
Bnear 200-year history to continue to serve the people of Boston and visitors to the birthplace of the American Revolution. Quincy Market (c. 1826) is part of Faneuil Hall Marketplace which also includes Faneuil Hall (c. 1742), North Market (c. 1826), South Market (c. 1826), and Pavilion (c. 2016). Located along Boston’s Freedom Trail and adjacent to the Rose Kennedy Greenway, Faneuil Hall Marketplace is an example of successful adaptive reuse. Quincy Market has transformed from a market pavilion of vendor stalls at the center of trade in Boston to a mixed-use shopping and dining festival style marketplace. Over that time, the structure has been repaired, reinforced, and replaced to support the changing building function while the overall form and historic appearance has been preserved.
In 1734, market buildings were established in Boston near Orange Street, Dock Square, and the Old North Meetinghouse. Within three years, one building was converted into retail stores, the second disassembled and the third destroyed by a mob. Peter Faneuil, a wealthy merchant realized the need for a proper market building to support the Boston economy and offered to build one at his own expense. Designed by artist John Smybert,
the 40 foot by 100 foot building was constructed in 1742, destroyed by a fire in 1761, and rebuilt in 1763. Supplementing Faneuil Hall was a long wood-framed vegetable market to the north. The first floor of Faneuil Hall held vendor stalls while the second floor held a town hall assembly room. In addition to serving as a market, the building was the site of speeches by the Sons of Liberty to protest taxation without representation between 1765 and 1775, used by the British as a theatre in 1775 during the siege of Boston and held town meetings until 1822. Faneuil hall was expanded in 1806 by Charles Bulfinch by adding a third floor and increasing the width from three to seven bays.
By the early 1820s, it became clear Faneuil Hall and the surrounding market square were insufficient to support the needs of the growing city. Newly elected Mayor Josiah Quincy proposed an ambitious plan to extend the market district east of Faneuil Hall by filling in the harbor and reclaiming land. This required purchase of private land, buildings, and wharves to make way for the new market. The plan included widening the streets and constructing three new buildings: the North and South Market buildings with the main Quincy Market building at the center. Designed by Alexander Parris, Quincy Market is two stories tall, 535 feet long and covers 27,000 square feet. Constructed in the Greek Revival style, the market consists of a center 55 foot x 70 foot center rotunda with 50 foot x 232 foot east and west wings. A 12-feet wide colonnade runs east-west down the center of the market and is lined with Doric columns at 7 feet 6 inches spacing on either side. Retail merchant stalls lined the north and south sides of the colonnade. The center rotunda housed larger stalls and served as a receiving area. The second floor of the east and west wings remained unfinished while a center elliptical dome rose
above the center rotunda second floor.
The facade load-bearing walls of Quincy Market were constructed of white granite from quarries in Chelmsford, MA, and shipped down the Middlesex Canal. Four monolithic granite columns, each standing 26 feet tall, supported the roof at both the east and west entrances, echoing the design of Greek temples. Walls were supported with granite foundations consisting of large granite block strip foundations bearing on the natural sand/clay (Marine Deposit) soil. The cellar floor was underlaid by a system of timber cribbing and silty/sand fill to raise the grade. Over 16 feet of miscellaneous fill (sand, clay, organic silt, shells, wood) was brought in to infill the
harbor and raise the surrounding grade.
To minimize the number of columns in the basement, provide an open space for merchants to prepare and store goods, and limit foundations, Parris designed a unique structural system to hang the first floor from the second-floor structure. Cast-iron columns at the ground floor were located at every third colonnade column and sat on brick-masonry walls in the cellar. Tension iron hangers were located at the two colonnade “columns” between to hang the ground floor
Quincy Market is shown through it's phases in time: Top, right from left: circa 1828-1829, the Quincy Market Colonnade from 1850-1920; a Quincy Market merchant produce merchant (1934-1956). Below, from top to bottom: circa 1867-1895; 1890-1910; and 1940.
timber from timber trusses at the second floor, which spanned between cast-iron columns. Cast-iron columns and iron hangers between were clad in slender wooden Doric column cladding to give a uniform look to the ground floor colonnade. The result was an open cellar floor plan that maximized head-height while locating the cellar floor above the ground water elevation.
Parris used heavy timber trusses at 8 foot 6 inches on center to span the 55-foot width of the east and west wings to support the roof, creating an open second floor. The center rotunda was capped with a 55 foot x 70 foot elliptical dome. Parris leveraged the Delorme Method, invented by 16th-century inventor, Philibert Delorme, which laminated timber to create the double dome structure. Three layers of pine planks were laminated to create 77 arches at the exterior dome and 48 at the inner dome. The dome was stiffened with timber bracing connecting the upper and lower dome ribs. A tension ring at the base of the dome, atop the masonry walls, resisted the arch thrust.
In 1925, a significant fire tore through the east wing, compromising the existing roof framing and heavy timber roof trusses. The east roof structure was completely removed to the center rotunda. A new roof structure was provided, consisting of 2-inch tongue-and-groove timber decking spanning between steel channel purlins, stabilized with sag rods. New steel trusses were installed, approximately doubling the original spacing to 15 feet on center, and were comprised of steel angle chords and diagonals.
By the early 1930s, the age and neglect of Quincy Market had resulted in the deterioration of the building structure. As part of the New Deal, the Public Works Administration awarded the City of Boston a project to repair Quincy Market. Designed by McLaughlin & Burr and John M Gray Co. architects, the scope included replacement of the cellar, first, and second floor structure, de-coupling the first and second floor framing. In addition, canopies were added on the north and south sides of the building.
The first-floor structure was completely removed and replaced with a two-way 10 ½-inch concrete waffle slab supported by steel I-girders cast within the slab, eliminating the need for hangers to extend the second-floor structure. The secondfloor wood framed trusses and cast-iron columns were replaced with steel I-girders spanning to new concrete filled steel columns.
Steel trusses were added to reinforce the dome. The trusses were located below the dome coffered ceiling, with a new flat ceiling installed to hide the dome.
the facades of the North and South Market buildings and rebuild two units of the South Market that were destroyed by fire. Additions were removed and portions of buildings rebuilt per Parris’s original design.
In 1973, the Rouse Company of Columbia, MD, was awarded developer and management company for Quincy Market and the North and South Market buildings with a 99-year lease. Benjamin Thompson & Associates were appointed Architect with Nichols, Norton & Zaldastani, structural engineer. The project was one of the first urban renewal project of its kind and is considered the original “Festival Marketplace” with food vendors located at the ground floor of Quincy Market and specialty shops and retail lining the North and South Market buildings with office space above. A greenhouse “Flower Market” was added north of Faneuil Hall.
Elevators and stairs were added for accessibility. An elliptical floor opening was created at the second floor in the center rotunda, exposing the dome ceiling to the ground floor marketplace for the first time. The dome was strengthened with a steel frame inserted between the two domes and exposed tie-rods. Glass arcades with exposed steel HSS framing were added along the north and south sides of Quincy Market to expand space for indoor market vendors. The project was a success and in 1977 was awarded the Harleston Parker Medal for most beautiful building in Boston.
Modifications to Quincy Market and Faneuil Hall Marketplace have continued over the nearly 50-year period
after the conversion to a Festival Market. Monumental stairs between the first and second floors were added at the rotunda to complete Benjamin Thompson’s original vision. Ben Thompson’s Flower Market greenhouse was replaced in 2015 with a retail pavilion. Vendors have come and gone requiring multiple tenant fit-out projects and modifications to the building. Mechanical systems have been replaced and upgraded requiring modifications for system distribution and dunnage framing for unit support. Visitors are welcomed to Boston and encouraged to embark on the Freedom Trail, taking in the sites of the birthplace of the American Revolution. The journey begins at Boston Common, the oldest public park in America. Along the way, taking in the steeple of the Old North Church, one can reflect on where Paul Revere's midnight ride ignited the flames of independence with the signal, "One if by land, two if by sea." Boarding the USS Constitution, known as "Old Ironsides," offers a chance to stand in awe of the oldest commissioned warship still afloat. The journey concludes at Bunker Hill, where the echoes of the first major battle of the Revolutionary War still reverberate through time.
Amidst this journey, a stop at Faneuil Hall Marketplace where a visit to the Quincy Market is a must. Here, visitors can shop at local retail vendors, watch street performers, and grab a bite to eat at one of the many diverse food stalls, all while marveling at a building whose structural evolution mirrors the spirit of resilience and vibrant history of Boston. ■
With a metal building anchoring its warehouse, Costco has designed its ½-million-square-foot roof for public recreational use in Mexico City’s Parque La Mexicana.
By W. Lee Shoemaker, Ph.D., PE
Strolling through the sprawling 70-acre public Parque La Mexicana in the Santa Fe neighborhood of Mexico City, locals and visitors enjoy paved trails, vegetation, sculptures, fountains, restaurants and lakes with the city skyline serving as a beautiful backdrop.
Amongst all the greenery, one would be hard pressed to spot a ½-million-acre Costco warehouse, parking garage and loading dock. Why? Because it’s largely concealed under what is arguably the largest green roof ever to cover a retail building.
Not only is the green roof a sight to behold, but it provides a healthy home for thousands of native plants, primarily succulents spanning 10 species, and a total of 15 insect hotels that were created in partnership with Mexico City’s environmental agency. A welcome addition to the city, the unique green roof was designed in the spirit of an agreement with the local parks commission which allocated 30% of Parque La Mexicana for commercial development in exchange for companies covering the park’s operating costs.
“Designs that provide green space and the use of cool and green roof technologies in cities can reduce heat-island effects, producing multiple benefits and cost reductions by helping to reduce emissions and air pollution, human health risks, and economic losses due to reduced labor productivity,” cited researchers in the Fourth National Climate
Assessment by the National Oceanic and Atmospheric Administration. While the green roof is not open to the public, action abounds next to it on a public soccer field, two basketball/volleyball courts, a padel court and a children’s skate park—all connected with walking, running and biking paths.
In building the massive Costco structure, the design team analyzed seismic codes and evaluated the options for the most efficient ways to use the space. Their research led to the design of three separate buildings: a metal building for the retail warehouse, a post-tensioned concrete parking garage and a loading dock of conventional concrete and metal decking. The warehouse is 144,522 square feet, and the entire project is 524,549 square feet.
The metal building’s advantages include sustainability, flexibility, durability, longevity, aesthetics and affordability, among others.
“The metal structure is made from 80% recycled steel,” said MG2 Architects/ Maribel Barba, AIA, in an Arcat Detailed podcast interview. “This minimizes the amount of new material utilized. In addition, the decreased thermal mass of the metal reduces building
heat absorption, as compared to a typical masonry block wall.”
Working with the building manufacturer, Butler Manufacturing, and the structural engineer, Engineers Northwest, Inc., Span Construction and Engineering erected the metal building system, which comprised columns, beams, roof joists, roofing panels, an integral gutter system, wall girts and metal wall panels. These elements form the building shell. Because the metal building can support large spans, it significantly decreased the number of joists and columns required for the building when compared to other forms of construction. As a result, Costco is able to create a more seamless shopping experience due to a reduced need for structural columns breaking up the floor plate.
Each roof for the warehouse, parking garage, and receiving area support distinctive loads and were consequently constructed with different materials. While there was initially a concern about the metal roof’s ability to support the load of a green roof, it turned out the loads were not as high as expected. As a result, MG2 could design the warehouse with the same amount of steel as the firm typically uses for most of the Costco projects in which they are involved. The warehouse, with the green roof above it, is made of metal that is composed of 80% recycled steel. While 20 psf is a base roof live load, the super imposed roof load was 30 psf overall and 49 psf for a 2-foot perimeter around the roof.
Durability also tops the list of key metal roofing benefits. According to a study conducted by the Metal Construction Association and the Zinc Aluminum Coaters Association, the projected service life of a standing seam metal roofs (SSMR) is a minimum of 60 years. This is generally considered the longest service life of any commonly used roof system currently on the market. Placing a green roof over the roof should not have an impact on the lifespan of the roof due to the introduction of a complex drainage system and the inclusion of a Sarnifil membrane, a multi-layer, synthetic roof waterproofing product.
At the same time, the three-level parking garage roof houses the sports courts and had to support the adults, athletes, and children
using the courts and occupying the space as spectators. To support these loads and meet city restrictions on how high the structure could be, MG2 went with post-tensioned concrete.
“This is a very efficient design that utilizes less concrete than other common concrete structures while still holding heavy loads from the sports park,” Barba said. “The thickness of the floors is a little over 5 inches, which is quite thin compared to other concrete structures.”
As for the loading dock underneath the skate park, the team was challenged to create a column-free span large enough to enable a semi-truck to enter, unload its merchandise, make a U-turn and exit the structure. It was constructed by combining metal steel columns and conventional concrete metal decking.
The solution involved custom fabricating a 90-foot-long, 12-foot-tall beam, providing adequate clearance and maneuverability inside the dock area. The three-level parking structure is post-tension concrete. “The rest of the receiving area was designed with steel columns and conventional concrete metal decking to hold up the weight of the children’s skate park,” Barba said.
With the facility built on the site of a former sand quarry that later became a landfill, the civil and structural teams also had to address the site’s poor soil conditions. In addition, Mexico City and its surrounding suburbs are in an earthquake-prone region considered to be one of the highest seismic zones in the world. In 2017, a 7.1 magnitude earthquake devastated Mexico City, killing 369 people and damaging hundreds of buildings. And in 1985, an even larger 8.0 magnitude event took the lives of at least 5,000.
For the Costco project, the team worked with three different building types, each of which react differently to earthquakes. “Per code, we needed to add a seismic gap between each building type,” Barba said. “However, people still needed to be able to move between the buildings, so different types of seismic joints were used throughout the project.” The use of a metal roof provided an extra value from a variety of perspectives.
“Today’s metal roofing is a strong choice for even the most vulnerable structure,” wrote Robert Zabcik, PE, in a recent white paper “Metal Roofing Performance and Wind Events.” He added, “It is well documented that buildings with secure, storm-resistant roofing materials like metal are less prone to damage from hurricanes and high-wind events. Metal roofing’s strength and durability provide safety and security for building owners and occupants alike.”
In the case of the metal building, it is important to consider that modern seismic design focuses on providing sufficient ductility in a structure to absorb and dissipate the massive energy produced by an earthquake. The International Building Code (IBC) serves as the basis for legislative building codes in Mexico and three steel moment frame systems are currently defined and permitted in the building code for resisting seismic lateral loads. Each has a different design rule that recognizes the amount of ductility that is anticipated, primarily based on the inelastic rotation that can be expected at the beam-column connections.
Mexico City's local code doesn't use the term Seismic Design Category (SDC) as in the United States' ASCE 7, but it does use it as a reference to provide the required level of detailing. The current version of the Mexico City building code includes two design procedures with different levels of complexity, with the most complex rules aimed at large, important structures.
Note also that Seismic Code Evaluation–Mexico, created through an evaluation conducted by Jorge Gutiérrez, confirms that “the evaluated Norms may be considered state of the art but they are specifically meant for Mexico City, with very specific seismicity and site conditions. National authorities should be encouraged to issue a National Code with due consideration to the country’s seismicity and site conditions.”
Also note that the steel moment frames used in metal building systems differ from the prototype steel frames evaluated in the post-Northridge research program. Metal building system frames are optimized to match the strength required at any location on the frame, so the frame members are built up from welded plates that are commonly web-tapered, with the web thickness and flange size selected to optimize material along the length. The members are slender, with thinner flanges and webs than hot-rolled steel shapes that are typically used in multi-tiered conventional steel construction.
All structural systems defined in the building code for carrying seismic lateral loads are assigned design rules. These rules, including the maximum building height permitted, depend on the seismic design category which includes the seismic hazard at that location and inherent ductility that each system embodies.
Research by the Metal Building Manufacturers Association (MBMA) and industry partner American Iron and Steel Institute (AISI) evaluated the seismic behavior of full-scale metal building frames at the University of California, San Diego (UCSD) under the leadership of Dr. Chia-Ming Uang. Many observations were made in this study, including that metal building moment frames behave quite differently regarding ductile design philosophy. The metal building frames demonstrated little conventional ductility. A hot-rolled shape in a multi-tiered moment frame exhibits ductility by forming a plastic hinge at the location of highest stress, typically in a beam near the connection to a column. However in a metal building, the performance of slender, built-up tapered frame members is governed by buckling of the flange or web, or a combination of both, before a conventional plastic hinge can be achieved. Also, the location of the buckle in a metal building gable frame is closer to a web depth transition that can be several feet away from the column.
loads.
More recent research sponsored by MBMA and conducted by a team led by Dr. Benjamin Schafer of Johns Hopkins University used high fidelity finite element models of metal building frames that were able to reproduce the inelastic response noted here. This study confirmed that the current R-values used for seismic design of ordinary moment frames is appropriate for the metal building type frames.
In line with the community building aspect of the project to develop the rooftop for a variety of public recreational uses, the design team was also challenged to blend the buildings into the natural landscape.
In addition to siting some of the building underground, small berms, called Montañitas, were planted around the walls to conceal the building even more. These green screens offer benefits of natural ventilation and daylighting inside the facility. As the vegetation grows, the look of the building will change over time, resulting in a dynamic and ever-evolving feature. Costco is also making an additional contribution to Parque La Mexicana in the form of water. The three different types of roofs and surfaces were custom engineered, using a combination of siphonic drainage and gravity drainage systems, to collect and transport stormwater runoff to the park to help sustain its fountains and lakes.
In addition, a wastewater treatment system creates greywater for reuse, thereby reducing the building’s water consumption in line with building regulations governing this drought-sensitive locale.
In gaining a primary location in the heart of Santa Fe—and in exchange for providing greenery and sports courts to the community, and funding for park maintenance—Costco’s arrangement with Parque La Mexicana is an ideal private-public partnership.
“Every hurdle we overcame and every milestone we reached added to a growing sense of achievement that culminated when we witnessed the impact of our work firsthand—the creation of a space that enriches lives and fosters a strong community spirit,” Barba said.
W. Lee Shoemaker, Ph.D., PE, F. SEI, is Director of Research and Engineering, Metal Building Manufacturers Association.
Are our universities actually hurting the pipeline to the profession?
By Chris Cerino
hear many discussions regarding the crisis of the engineering pipeline. And while I am not sure there is actually a crisis, I do think that the legacy format of university programs hurts overall retention of college students that are considering entering the profession.
As a structural engineering hiring manager for 25 years, and as an active university career advisor, I have seen many variations of college engineering programs. When reviewing the transcripts of job applicants, I have seen many cases where new graduates are missing courses that I consider fundamental to the structural engineering profession, and in some extreme cases I think the graduate was not appropriately prepared by their university to enter the workforce with their four-year degree.
I sit in the middle of the philosophical divide between supporting a classical engineering program that demands an expert knowledge of the fundamentals and a program that is progressive, inspiring, and inviting to the next generation. With this lens, collegiate engineering programs have two major challenges that should be reconsidered – general education and ABET requirements.
Maybe there was a time that needed the ‘weed out’ classes that are the majority of the first two years in engineering, but now with flashier, and possibly more lucrative, careers in all mainstream conversations—should programs still look to eradicate ‘unfit’ prospective engineers? And does success in a class like Ordinary Differential Equations provide a good barometer for the ‘fitness’ of a potential engineer?
Consider the stats of a representative engineering program:
• Credits required to graduate with a bachelor’s
degree: 120-130 (or 15-16 per semester).
• General education credits required: 35-42 (roughly 30% of the total).
And in the first two years of study, consider this representative course breakdown (23 total classes):
• Six chemistry and physics classes (including Statics and Dynamics)—These are a good portion of the weed-out classes and are required by ABET for accreditation. Remember back to your days in these classes. They are usually large, you and the other, generally introverted, engineers-in-training (EITs) are trying to make friends, so you are not completely on your own, and a student’s success is very professor-dependent.
• Four math classes—The other large portion of the weed-out duo with similar challenges, and again required by ABET for accreditation. But chemistry and physics classes at times have interesting units or labs, while math classes are generally uninspiring to young engineers. Also, in many instances they are taught through the math department whose students and staff can be very different from those in engineering.
• Six general education (GE) classes—While there are certainly some interesting class offerings, as a freshman and sophomore you don’t get an early selection slot. So generally, you wind up with GEs you don’t really want—they are just available and fit in your schedule. And again, back to the introvert not knowing anyone but with even more randomness in classmates.
• Two English classes—I am definitely in favor of technical writing and public speaking classes if geared specifically to engineering majors; I am not in favor of having them in freshman and sophomore years. I think these classes would be better suited in the latter two years as I see them more as preparation for capstone, interviewing, and your career, not your specialized technical engineering classes.
• One computer science class—Very useful to know Python and other programming applications.
• One statistics class— Debatable if useful, generally not fun for the EITs, but required by ABET.
• And finally, three classes (out of 23) that are representative of the actual practice of civil or structural engineering, generally a survey of engineering disciplines course, freshman design, and then Strength of Materials.
So, in the first two years of an engineering program, the pipeline students of our profession must fight through roughly a dozen ‘weed out’ classes and six general education classes, while seeing only three classes that give them an appreciation of their actual major. Is it a wonder why students leave engineering? In many cases students persevere because they have a relative or mentor that is an engineer and can coach them through these years explaining that ‘it gets better when you hit your major classes, keep going!’ Without this connection, or a great internship, it is hard to comprehend the amazing career at the end of the journey based on the first two years.
The NCSEA Recommended Structural Engineering Core Curriculum document (www. ncsea.com/engage/committees/ academia-practice-partnerships/),
which comes from the national 2021 NCSEA Practitioner Survey (www.structuremag.org/ article/ncsea-2021-practitionersurvey/), consists of 12 core classes all new hires should have completed. In addition, I would include two classes in computer programming and computer analysis and two classes of capstone design.
So, that is roughly 50 credits, most of which must happen in years 3 and 4 in the representative system. I am sure you are seeing where this is headed. It is quickly obvious that after the 62-64 credits in your first two years, you would still need six more GE counting classes (18 credits) and 47 credits of core classes, for a total of 128 credits. I know this sounds like it is right on target, but it doesn’t account for the many tangential classes that employers consider important such as Engineering Economic Analysis, Construction Methods, Professional Ethics, or Sustainable Design.
So now the problem is clear. Either a fifth year/master’s degree is required or many key classes must be omitted, leaving the new graduate to learn on the job, saddling employers with this investment in the current era of squeezed schedules and fees.
I recommend that universities rethink general education requirements for engineering, and likely all, majors. Requiring 35-42 credits, roughly 30% of your classes, in the name of well-roundedness is a great disservice and misleading to the student about the adequacy of their four-year education. While courses like Visions of Nature, Historical Geology, and the History of Science could be very interesting, the far-reaching forced breadth of general education classes should be replaced with a slate of classes relevant to their desired career. With the average cost of college today, a student will spend over $50,000 on just
So now the problem is clear. Either a fifth year/master’s degree is required or many key classes must be omitted, leaving the new graduate to learn on the job, saddling employers with this investment in the current era of squeezed schedules and fees.
general education courses, a tremendous investment! Compounding this, students become frustrated with engineering as a major since these GE requirements kept them from taking the classes that would have helped to reinforce their choice of major and profession.
The ABET requirements should be reconsidered because they represent the majority of the weed-out classes. This is where I will side a bit with the traditionalists of engineering curriculum. I appreciate that many of these courses are the foundations of the core technical classes, and this riteof-passage learning should not be passed over. But these courses are very challenging to most future engineers, and they likely occur as they are making a difficult transition to living on their own for the first time. Shouldn’t we then be nurturing and inspiring these students with the best professors, ample teaching assistants, and targeted connectivity that makes sure they see the bigger picture and make it to the classes they will enjoy?
Colleges and universities are tasked with transforming young minds into adults that are ready to enter the workforce in a given profession. While
it is important that students do not falsely believe they are adequately prepared for a career in engineering when they are not, it is also important to not drive students away from engineering as a career based on legacy principles and curricula. As with the field of engineering itself, it is critical that engineering programs always look to evaluate and improve their offerings based on feedback from employers and the evolving needs of today’s students.
In today's world, the "better, faster, and cheaper" mentality goes only so far; structural engineering firms can turn to three additional values to set themselves apart.
By Peter Atherton, PE
"Better, faster, cheaper.” This is the old “All 3,” a timeless desire unlikely to ever change.
It wasn’t long ago that the standard response to All 3 requests was “pick two.” For a project to be better and more cost effective, it will take more time. To pull together some plans to meet the budget and time constraints, there will be the assumption of more risk. Or for a top quality project design that will meet the timeline, it will cost more as workflow and teams are reprioritized.
However, with the rapid emergence and accessibility of more powerful AI and advanced technology, more of our competitors will not be so constrained by past norms.
As structural engineers, ensuring our growth and prosperity in this new era of unparalleled marketplace and practice space changes requires something both new and different.
Knowledge, client focus, good communication, and responsiveness used to be enough to safeguard our business and professional success. Although these are still critical (and always will be!), more is needed.
Knowledge—the facts, information, and skills acquired by a person through experience or education –is used to differentiate us.
However, when the cost and availability of basic knowledge— technical or otherwise—is essentially free and immediate with an intelligent “prompt” into a powerful AI-powered large language model, what’s the advantage of paying or waiting?
The same is true in terms of design when a smart prompt placed within a powerful graphics model can instantly generate an image of possibility.
What WILL be worth paying for and appropriately waiting on is our ability to provide what I call the “New All 3”:
1. Deep expertise and expertly applied knowledge
2. Execution excellence
3. Trusted relationships Deep expertise tells us (and those we serve) what’s wrong or missing from even the best prompts and models. Expertly applied knowledge is what allows us as obligated professionals to ensure public health, safety, welfare, and policy goals, while meeting budget constraints and investment returns on projects and pursuits that, by their timing and nature, are never technically, politically, financially, geo/ecologically, and jurisdictionally the same.
Execution excellence includes client focus, good
communication, and responsiveness, and has become an expectation across disciplines.
Trusted relationships, both internal and external, are only increasing in importance as we become more digital and reliant on technology tools. Trust is also inherent in our duty to serve.
Succeeding with “better, faster, cheaper” is a good thing if done strategically and in sequence. If not, it can ultimately limit and commoditize us.
Whether project, proposal, or talent related, our basic internal operations have many known inputs and well understood outputs and variables making them ripe for both automation and augmentation.
Thinking more strategically to solve for the old All 3 internally can yield process and systems optimization and essential learnings—and in doing so, the promise of newfound internal capabilities and time and financial resources. These new treasures can then be used to increase capacity, lighten the load, and reinvest.
Similarly solving from an external marketplace perspective with so many different and less understood inputs, outputs, and variables, often related to evolving client desires and emergent project and location constraints, is less fruitful—and trying to do so limits us to what’s present and known and to relatively simple and straightforward projects.
There will be demand for simplicity—and if this is the game we choose (or default to), we must be ready for the emergence of our industry’s version of Walmart and Target that will look to fill this market niche and put downward pressure on pricing.
For most of us, our best move is to leverage our newfound insights and resources to play a
Solving bigger and more meaningful, timely, and complex problems in more creative, thoughtful, and cost-effective ways is of great (and increasing) value to all asset owners and developers.
different and much more valuable and strategic game—one that expands client opportunities and choice.
This game is about providing “net new value.”
The fact is most of our projects in engineering and architecture are not so simple. It’s also true that advancing them in silos or without grander consideration of community and resiliency needs, decarbonization trends, and the energy transition won’t serve us well individually—or collectively.
Solving for these, especially in the face of increasing costs, regulation, and technological change certainly adds complexity—but is also our opportunity.
Solving bigger and more meaningful, timely, and complex problems in more creative, thoughtful, and cost-effective ways—and doing so in consideration of all five phases: planning, design, construction, operations, and maintenance—is of great (and increasing) value to all asset owners and developers.
To become (or remain) a more valued project partner, we must resist “supply chain” interchangeability. Each discipline must ensure it provides value both up and downstream of any previously distinct roles or functions. For any design firm this could include leveraging its unique expertise through and alongside technology, either commercially available or bespoke, to provide expanded possibilities and increased certainty faster and more effectively during the planning stages so
that ultimate or enhanced asset design, construction, operation, and maintenance goals are met. Delivering on such allows for a different value proposition. This then opens the door for more evolved business models designed to better capture the value we create—further adding to our opportunity.
The use of AI and advanced technology will be a key element to achieving this new level of success.
As entrusted professionals, it’s on us to learn how to best leverage the technology and not fall prey to the hype.
Real change is coming and as we explore and educate others on all that’s possible, we must keep in mind that:
• What’s easy and quick is rarely the best or in compliance with the standard of care
• FOMO (the fear of missing out) doesn’t inspire
• MVPs (minimum viable products) are not full-on solutions
• Opportunists, well-meaning or otherwise who understand much less about what we do, may make promises too good to be true.
Providing new net value can
help us to elevate on macro and micro levels while also achieving both incremental and exponential gains for ourselves and all those we serve.
To grow and prosper during this time of great change requires that we:
1. Recognize the reality that the old All 3 is not enough.
2. Understand and proactively build towards the “New All 3.”
3. Become better, faster, cheaper internally.
4. Leverage internal insights and resources to practice differently to solve bigger problems and create more meaningful opportunities externally.
5. Evolve our business models to capture more of the value we create.
6. Grow in our ability to lead our people and teams differently.
7. Strategically redesign our organizations to support our new ways of working. Unplanned change, disruption, and disintermediation don’t need to be our fate.
Our choice to individually and collectively evolve beyond the old All 3 is a powerful first step. Winning with the “New All 3” is more of a marathon than a sprint – but we need to make sure we’re moving… and starting now to apply these elements strategically and effectively is our key to enjoying greater next-level success moving forward.
This article was originally posted as a Next Level Blog on the ActionsProve website.
Peter C. Atherton, P.E. has over 30 years of AEC industry experience, 24 as a successful professional civil engineer, project manager, principal, practice group leader, major owner, and member of the board of directors with high-achieving firms. He is now the President and Founder of ActionsProve, LLC, a strategic planning, advisory, and leadership development practice focused exclusively in AEC. (pete@actionsprove.com).
M agnusson Klemencic Associates (MKA) announced the opening of the Seattle Aquarium’s new Ocean Pavilion. This 50,000-squarefoot addition includes three new immersive habitats and increases the Aquarium’s capacity by approximately 50 percent. MKA is the Structural and Civil Engineer of Record for the project.
MKA’s unique aquarium design—a geometrically complex, cantilevered concrete habitat serving as the building’s primary gravity- and lateral-force-resisting systems— incorporates 3D printing and Computerized Numerical Control for custom-cut formwork. The habitat’s unusual geometry and sloshing water effects greatly impacted the seismic design and detailing complexities.
The Ocean Pavilion is targeting Living Building certification in critical performance areas, focusing on planet-positive energy use.
“From a structural engineering perspective, it’s no surprise
the new Ocean Pavilion’s main habitat, The Reef, gets most of the attention,” said Hannah Bonotto, Ocean Pavilion Construction Project Manager.
“But I hope visitors also appreciate the exposed structural steel throughout the building. From the 80-foot-long kinked roof beams to the skewed back-ofhouse steel, no two beams on this project are alike—it’s an extraordinary project.”
The project’s civil design included extensive coordination with adjacent and concurrent projects—the relocated Alaskan Way and Elliott Way, as well as the Overlook Walk project. MKA arranged the relocation of utilities to align with the development of these projects and new utility services to the Ocean Pavilion. MKA also provided grading and hardscape improvements in the adjacent Promenade and the Alaskan Way sidewalks to meet accessibility requirements for implementation by the Alaskan Way relocation project and worked with the City of Seattle to permit the project through the Street
Improvement Process.
“Contributing to an iconic anchor of the revitalized Seattle Waterfront is a once-in-a-lifetime opportunity,” echoed Civil Project Manager Rita Greene. “Looking out (our) office window and seeing the Ocean Pavilion is a reminder of all the collective hard work and brain power everyone involved put into the project.”
The Ocean Pavilion also includes an accessible rooftop garden that functions as an extension of another MKA project, the Overlook Walk.
“The geometrically unique 350,000-gallon aquarium habitat with a 32-foot cantilevered section—complete with oculus—hovering over the main entrance was one of the most challenging concrete structures ever designed and executed by MKA engineers and modelers,” said MKA retired Senior Principal Jay Taylor. “That said, the structure’s most significant accomplishment may not be its gravity-defying design, but the building’s seamless integration with an unobstructed, transformative connection between the Pike Place Market and the reimagined Seattle waterfront.”
Other nearby MKA-led projects include Benaroya Hall, the renovation and expansion of the Seattle Art Museum, the Harbor Steps, Pike Place Market’s MarketFront Entrance and Garage, the Olympic Sculpture Park, and the Elliot Bay Central Seawall, another critical component of Seattle’s waterfront renaissance.
“The Ocean Pavilion is an accessible, immersive educational experience for all,” Bonotto concluded. “I’m so proud of what the project team achieved for the people of Seattle, and I can’t wait to show my friends and family this beautiful new addition to the downtown waterfront.”
In September, Williams Crossing welcomed its first public users across the Arkansas Riv-er. As part of the City of Tulsa’s vision for the improved Zink Dam area, schlaich berger-mann partner (sbp) was asked to design a new pedestrian bridge that would provide a safe and modern crossing between the east and west sides of the river and replace an existing bridge that was deemed structurally unsound and in need of major repair. The result is the 1,440-ft-long Williams Crossing.
This new symbol for Tulsa provides a connection to Gathering Place, an immense park de-signed by Michael Van Valkenburgh Associates (MVVA), as well as links to the River Parks and Midland Valley Trails. Williams Crossing is designed for both pedestrian and cyclist access and offers opportunities to stop and
enjoy the dramatic river views with seating and lookout areas along the curved 18-ft-wide bridge deck. sbp and MVVA collaborated closely on the design of Williams Crossing to integrate the bridge into the park landscape for a seamless user experience and create a bridge that is an elegant and unique structure for the community of Tulsa.
The bridge was designed in homage to Robert Maillart’s 1933 Schwandbach Bridge near Bern, Switzerland, which is a curved, deck-stiffened reinforced concrete arch bridge. Over the years, sbp has continued to refine Maillart’s elegant deck-stiffened arch concept, first with the 2003 concrete Auerbachstrasse Bridge in Stuttgart, Germany and again with the 2013 hybrid concrete and steel half-arch Footbridge Josenbrücke in Schwäbisch
Gmünd, Germany. The extreme slenderness of Williams Crossing’s deck-stiffened steel arches takes this typology a step further.
The deck-stiffened, plate-arch structure is the first of its kind in the U.S., and the bridge is also the first multi-span, plate-arch bridge built in the country.
Lightweight design principles were applied to the bridge’s superstructure to increase the efficiency of the bridge. The semi-integral bridge, which is supported by eleven 120-footlong arch spans and one 120-foot approach span, was designed with extremely slender arches built from 3-1/4-inch plate of weathering steel. The thickness of the vertical spandrel plates varies throughout the bridge to provide sufficient stability for live loads and at the same time allow enough flexibility to minimize
restraining forces during temperature changes. The composite deck consists of an 18-inchesthick reinforced concrete deck and 8-foot wide steel plate with stiffeners that were stiff enough to support the concrete weight during construction. Optimized and structurally expressed steel nodes allow for the transfer of loads from the superstructure to the substructure where the arch spans meet. The original concept for the nodes considered cast steel fabrication, but due to cost and lead time advantages, milled nodes from forged steel blocks were utilized instead. To im-prove the natural hydrology of the river, the total footprint of the piers was reduced (compared to the existing bridge) and the vertical deck clearance was increased to improve re-siliency during more frequent 500-year flood events.
Global integrated design firm Stantec was selected by Boston Unity Soccer Partners to redevelop White Stadium into a pre mier soccer venue for the city’s new National Women’s Soccer League (NWSL) team. The firm is providing architecture and landscape architecture services.
Designed by Fredrick Law Olmsted in the 1880s, the historic land mark Franklin Park is home to White Stadium, which was built in 1949 and has since fallen into disrepair. The existing building lacks the spaces, accessibility, systems, and amenities needed to be a city wide resource. When Boston was chosen as one of three cities for a NWSL expansion team, the stadium offered the ideal location for the team’s home.
Stantec’s design team developed a holistic proposal with the City’s design team to reactivate White Stadium through program develop ment and physical interventions. Improvements to White Stadium include roof canopies over new grandstand seating areas that will hold 10,000 spectators and the addition of a mixed-use space with food and beverage offerings that opens out to the Playstead playing fields. Called “The Grove,” the event lawn will host events such as tai chi, children’s storytelling, small performances, seasonal markets, and cultural gatherings, programmed with a neighborhood advisory group. The team will take special care to preserve White Stadium’s art deco façade as well as Franklin Park’s heritage trees and pastoral paths. Boston Unity Soccer Partners reached an agreement with the City of Boston that promises 20 days out of the year to the NWSL team, while the remainder are open to the Boston Public Schools (BPS) sports programs.
AThe West Grandstand and Grove will be renovated into 75,000 square feet of team areas, concessions, and fan lounges, leased by the professional team and renovated/rebuilt for their needs. The East Grandstand and field will be demolished and rebuilt by the City to accommodate a 75-yard-wide regulation field and track. It will focus on BPS’ needs with student wellness areas, locker rooms, athletic equipment storage, and BPS offices.
Construction is expected to be substantially completed by March of 2026.
groundbreaking Adaptive Sports Complex recently opened in South St. Louis County, Missouri, thanks to a collaborative effort between the St. Louis Cardinals' charitable foundation Cardinals Care, Gateway Region YMCA, Boniface Foundation, and Miracle League. KAI, a leading architecture, engineering, and construction firm, played a crucial role in bringing this unique project to life by providing architecture, MEP engineering, and project management services. St. Louis-based Planning Design Studio led the project and provided landscape architecture services.
The $5.5 million South County YMCA Adaptive Sports Complex is the first of its kind in Missouri and one of only 46 in the United States. This state-of-the-art complex offers children with disabilities the opportunity to experience the joys and benefits of playing sports in an inclusive environment.
At the heart of the complex lies a custom-designed Miracle League baseball field featuring a cushioned, barrier-free surface and accessible dugouts to accommodate players of all abilities. The field captures the essence of Busch Stadium, home of the St. Louis Cardinals, complete with a silhouette of the iconic Gateway Arch in the outfield.
The field was officially dedicated Paul Goldschmidt Field on June 25, honoring the St. Louis Cardinals’ MVP first baseman.
Beyond the baseball field, the complex boasts a soccer field, running track, outdoor fitness area, playground, office space, concession building, covered pavilion, family-style restrooms, and parking – all designed with accessibility as a top priority. St. Louis-based Musick Construction served as the general contractor.
The Engineered Wood Association (APA) recently updated The Performance Path to Energy Code Compliance publication. This free guide assists builders in evaluating the energy efficiency of a whole home as a system, enabling them to specify costeffective assemblies utilizing wood structural panels, eliminating the need for continuous insulation in the Northern climate zone while still complying with IECC requirements. Updates incorporate changes made in the newly released 2024 International Energy Conservation Code (IECC).
Download this free guide from APA’s website, www.apawood.org.
COWI, a leading global engineering firm, has been selected to support the City of Berkeley in its ambitions to transform the Berkeley Municipal Pier into a dual-purpose pier featuring ferry access as well as public recreation.
The project will contribute to the rejuvenation of the Berkeley Waterfront, offering a distinctive and memorable experience for residents and visitors. The project aims to create a destination that offers entertainment and relaxation for residents and visitors while also addressing the congestion on the OaklandSan Francisco Bay Bridge while.
The City of Berkeley commencement of the engineering design contract with COWI represents a significant milestone after a rigorous two-year undertaking, including a comprehensive one-year public process for the first ferry terminal designing for all-electric ferry use.
COWI is tasked with providing engineering services to advance the project through concept development, final design, bid, and construction. COWI will work closely with City staff, Water Emergency Transportation Authority (WETA), and the environmental team to deliver a facility that will be a key transportation link, after a large seismic event.
JLG Industries, Inc., an Oshkosh Corporation business [NYSE:OSK] and a leading global manufacturer of mobile elevating work platforms (MEWPs) and telehandlers, announced that Oshkosh has completed the acquisition of AUSACORP S.L. (AUSA), adding the market-leading brand’s compact allterrain machines for the transportation and material handling to the company’s Access segment. Following the acquisition of Hinowa in 2023, the AUSA acquisition further supports the Oshkosh accelerated growth strategy and strengthens the JLG® equipment portfolio.
IAPMO has published a new white paper titled Adaptive Reuse: Converting Offices to Multi-Residential Family, a comprehensive analysis of the transformative approach to addressing housing shortages through the conversion of office spaces into residential units. Authored by Christoph Lohr, P.E., CPD, LEED AP BD+C, ASSE 12080, vice president of IAPMO Technical Services and Research, the white paper delves into the challenges and opportunities presented by adaptive reuse projects. the white paper may be downloaded at https://iapmo. org/media/32568/adaptive-reusewhite-paper.pdf.
John Huntoon, Ph.D., EIT, a recent graduate of the geosystems engineering program at the Georgia Institute of Technology and current president of BioBilt Infrastructure Systems, is the winner of the DFI Educational Trust Student Paper Competition. His paper is titled, “Analytical Model of In-Situ Root-Inspired Ground Anchor Actuation and Pullout.” The 2024 winner receives a $1,000 travel stipend and a complimentary 2-year DFI Individual Membership.
NCSEA recently released the results of a survey that highlights the critical role structural engineers play in the design process, particu larly when engaged early. The survey reveals that over half of the respondents (52 per cent) believe they add significant value when included in initial discussions about major design changes. Additionally, 45 percent noted that early involvement helps ensure a unified vision across the design team.
The survey also found that nearly 40 per cent of structural engineers see “simple” plan changes as having substantial ramifications on structural design. Early and open com munication with architects can mitigate these challenges, keeping projects on track and within budget.
This survey is part of NCSEA’s ongoing efforts to highlight the role of structural engineers and improve collaboration within the industry. By sharing these insights with architects and other stakeholders through the We SEE Above & Beyond campaign, NCSEA aims to foster more successful projects and a stronger partnership between disciplines. To learn more, visit www. weseeaboveandbeyond.com.
NCSEA is pleased to announce the finalists for this year’s Young Member Group of the Year award, which honors YMGs that contribute to the advancement of their young members, SEAs, and communities. The finalists for 2024 are:
• Structural Engineers Association of Colorado
• Structural Engineers Association of New York
• Structural Engineers Association of Northern California
To ensure structural engineers receive the most relevant and targeted information from NCSEA, it’s important to regularly log in and update profile details. Confirming information such as title, job function, and birthdate allows NCSEA to better customize communications to meet individual needs.
How to update a profile:
(1) Log in at NCSEA.com (or select “My Account” if already logged in).
(2) Scroll to the “My Account” menu in the Customer Center.
(3) Select “Edit Profile” and update the information. Keeping profiles current helps NCSEA provide better service— thank you for taking the time!
Finalist groups receive complimentary registration for one representative to attend the NCSEA Summit, along with a $1,000 travel stipend to cover transportation and hotel costs, generously sponsored by Computers & Structures, Inc. (CSI).
The winning Young Member Group will be announced at the 2024 Summit and will receive an additional $2,500 to use for future activities.
The NCSEA Code Advisory Committee launched a new six-part webinar series, Mastering Building Assessments:
Essential Skills and Best Practices for Structural Engineers. While the series ran from August 20-September 24, folks can watch any of the recorded webinars that they missed at www.NCSEA.com.
Designed to provide structural engineers with critical assessment skills, the series covers key topics such as legal considerations and the evaluation of various building materials, including concrete, wood, steel, and masonry. Each session is led by industry experts, offering practical insights into conducting thorough and accurate building assessments.
Webinar episodes are:
• Building Assessments 101
• Legal Aspects of Building Assessment Reports
• Building Envelope Assessments
• Assessing Concrete and Parking Structures
• Assessing Wood Structures, Decks, and Balconies
• Condition Assessment of Existing Steel Structures and Masonry Buildings
Individual webinars are available for $150 (members) and $250 (nonmembers), and the full series is available for $495 (members), $895 nonmembers. NCSEA also offers a webinar subscription at $1,795 that includes all live and on-demand NCSEA webinars for a full-year after the purchase date.
As part of a strategic partnership with WTW Architects & Engineers (WTW A&E), a unique session will be offered at the NCSEA Structural Engineering Summit on Nov. 8 at 9:45 a.m. This full disclosure presentation, “Sharing the Story of an Actual Claim Against a Structural Engineer,” will dive into an actual claim against a structural engineer, led by the defending engineer and defense attorney. Unlike typical sessions, this one won’t redact names, giving participants a complete look at both the technical and legal details. However, attendees must agree to keep the specifics confidential. The session aims to provide valuable lessons on professional practice and claims management, helping attendees mitigate risks in their own work. Register for the Summit by Sept. 30 to qualify for an early registration discount and secure your spot at this must-attend event Nov. 5-8 in Las Vegas. Registration and more details are available at NCSEASummit.com.
of live NCSEA education webinars (25+) and a recorded library of past webinars (170+) – all developed by leading experts; available whenever, wherever you need them!
As my term as President of the Structural Engineering Institute (SEI) comes to an end on September 30, 2024, I am enthusiastic about the major innovations occurring throughout the structural engineering profession, and the breadth of activities occurring in SEI to drive the profession forward.
SEI leads the way on topics of addressing sustainability and resilience, new technologies in the AEC industry, educational initiatives in structural engineering, creating national design standards for the profession, and other initiatives. In the past year, SEI has held several workshops related to wind engineering and tornado design in conjunction with NIST and other federal organizations to establish a path to enhanced resilience and improved design standards for extreme wind events. On July 22-24, 2024, SEI led a workshop at Northeastern University in Boston entitled “Towards Zero Carbon: Developing a Roadmap for the Structural Engineering Profession and the Structural Engineering Institute, ASCE.” The workshop provided an opportunity for leaders across diverse fields—from materials experts and design and construction professionals to educators and policy advocates—to brainstorm on priority initiatives that will help drive towards zero carbon in the built environment and help shape the role of the Structural Engineering Institute in leading relevant initiatives. The speaking program was led by Brendan Owens, the Assistant Secretary of Defense for Energy, Installations, and Environment Chief Sustainability Officer within the Department of Defense, who is responsible for sustainability initiatives and design for over 500,000 buildings overseen by the Department of Defense. We also heard from architects, contractors, policy experts, and engineers who highlighted that the industry is poised for major initiatives to address embodied and operational carbon in design, construction, and use of buildings and civil infrastructure. Participants developed a range of ideas that will be published in a report to highlight continued opportunities to address sustainability in the built environment. As workshop co-chairs, Jennifer Goupil, SEI Managing Director, and I will be following up with participants, other volunteers, and other structural engineering organizations internationally to continue to develop tools and strategies, policy initiatives, national standards, and educational opportunities to address this critical topic.
If you are interested in learning more and getting involved with SEI, please join one of our committees, attend our
workshops and conferences, and help us lead the way to achieve a more sustainable and resilient future. It is straightforward to apply to be on an SEI committee—we especially encourage people who are newer to the profession to join any of a number of our committees in the SEI Technical or Professional Communities. Apply here: go.asce.org/sei-committees. In that spirit, please mark your calendar for the 2025 SEI Structures Congress, which will take place April 9-11, 2025 in Phoenix, Arizona. There will be many sessions and committee meetings addressing a wide range of topics in our profession. Receive Updates at: www.structurescongress.org/.
I am also pleased to let you know that the SEI Board of Governors recently established two new awards in honor of leaders who made significant contributions to the structural engineering profession:
• The James A. Rossberg Award for Collaboration | ASCE recognizes an individual who has advanced the profession through collaboration with engineering entities and/or professional associations. The award was created in honor of the late Jim Rossberg, the founding Director of the Structural Engineering Institute and a long-time leader and convener within the profession, ASCE, and SEI. Learn more: go.asce.org/JamesARossbergAward.
• The David P. Billington Award | ASCE recognizes the excellence, profound public impact, and sustained achievements of a professional who best demonstrates the late David Billington’s legacy as applied to structural engineering education, research, and practice. Professor Billington was a pioneering scholar of Structural Art at Princeton University, a world-renowned educator, and a generous and inspirational mentor. He was a tireless public advocate for structural engineering, its societal relevance, and the importance of its interfaces with other disciplines. Learn more: go.asce.org/ david-p-billington-award
Please consider nominating worthy individuals for these and other SEI awards at www.asce.org/SEIAwards by November 1.
I would like to close by thanking the SEI Past-President, Don Scott, who will be rotating off the SEI Board of Governors after years of service, including leading our recent SEI reorganization. I would also like to thank Greg Soules, who will be rotating off the SEI Board of Governors and has been an outstanding contributor during his term on the Board. Greg also served as the inaugural leader of the SEI Technical Community Executive Committee, a key role during the reorganization of SEI. Finally, I would like to thank the SEI Board of Governors, the SEI Community Executive Committees, the SEI committee chairs and members, and the many other SEI volunteers with whom I have interacted during my term as President. I would especially like to thank Jennifer Goupil and the entire SEI staff for their critical contributions to our profession and our institute. Jennifer’s leadership of the Institute and her work on resilience within ASCE remain a hallmark of excellence within the profession.
Congratulations and best wishes to incoming President Stephanie Slocum, the first woman President in the history of SEI! Stephanie has outstanding vision for the profession and Institute, and we look forward to her leadership during this pivotal time for structural engineering.
Jerome F. Hajjar, Ph.D., PE, NAE, F.SEI, F.ASCE SEI President
• Advance and be recognized as an SEI Fellow - Must be current SEI member, actively involved in SEI, 10 years responsible charge (typically post P.E./S.E.) www.asce.org/ SEIMembership
• O.H. Ammann Research Fellowship in Structural Design and Construction
• SEI and ASCE Structural Awards
ASCE conducts a minimum 45-day Public Comment period on any new, revised, or reaffirmed standard. Public Comment for ASCE-SEI 24 Flood Resistant Design and Construction is currently open with Comment Deadline Sept. 9, 2024.
Access the ASCE Public Comment System here: go.asce.org/ codesandstandards.
The errata is in effect as of July 2024 so be sure you head to ascelibrary. org to look at all of the changes to the standard and Supplement 1. When using the standard please be sure to refer to the errata for accurate use and application of the provisions.
The revision cycle for Standard for Mitigation of Disproportionate Collapse Potential in Buildings and Other Structures (ASCE/SEI 76) will begin in 2024. The committee is seeking new members to begin work on the next edition of the standard. If you are interested in applying for the main committee, or any of the subcommittees or task committees, please apply by November 30, 2024. Apply here: go.asce.org/sei-committees.
The SEI Performance Based Design Committee is seeking new members. If you are passionate about PBD and would like to participate in an SEI Technical Committee revision, submit your application online anytime. Apply here: go.asce.org/sei-committees
Thanks to our many generous donors, and especially Ashraf Habibullah and Compters & Structures, Inc for the 3 to 1 donation match in 2023, for FY2025 the SEI Futures Fund Board has been able to commit a record amount more than $400,000 for these strategic programs:
• More than 100 Student & Young Professional Scholarships to engage at Structures Congress 2025
• Student Scholarships to SEI ETS Conference
• Performance-Based Design Roadmap Development Workshop
• Towards Zero Carbon Bootcamp
• Young Professional Travel Support on SEI Standards Committees
• Publish Prestandard for Calculation Methodology for Structural Systems in Whole-Building Life Cycle Assessment
• SEI Chapter Innovative Programs for Student and Professional Involvement
• Finalize, Publish, Distribute ASCE/SEI 7 Student Primer
• Business Practice Education for Students & Young Professionals
October 15-18, 2024
Hilton Riverside, New Orleans, LA
Registration Link: https://conference.acec.org
Held at the Hilton Riverside in New Orleans, LA this year’s conference offers unique educational opportunities, including the two sessions from the Coalition of American Structural Engineers (CASE). Both sessions will deliver critical insights to sharpen your expertise and strengthen your firm’s future.
Navigating the Legal Landscape: A Structural Engineer’s Guide to Risk Management
Speaker: David Eckmann, SE, AIA, PE, Principal at Magnusson Klemencic Associates
This session is essential for anyone looking to minimize legal exposure in structural engineering. David Eckmann, a seasoned leader in highprofile structural projects, provides actionable strategies for managing risk, negotiating contracts, and protecting your firm from potential liabilities. This is a must-attend for engineers at all levels who want to build a more legally sound practice.
Emerging Trends in Structural Engineering: Sustainability, Resilience, and the Future
Speaker: Emily Guglielmo, SE, Principal at Martin/Martin Consulting Engineers
With sustainability and resilience becoming top priorities, Emily Guglielmo leads a session exploring innovative design approaches and materials that align with the industry’s green future. Engineers looking to stay competitive while incorporating sustainable practices into their designs will find this session particularly valuable.
Beyond CASE
In addition to a broad array of educational sessions, the conference boasts a packed schedule of keynote speakers, networking events, and insights into ACEC’s advocacy efforts. From expert-led CASE sessions to inspiring keynotes, you’ll walk away with actionable strategies, deepened expertise, and invaluable connections. Plus, you’ll gain insight into how ACEC’s advocacy efforts are driving positive change across the industry—ensuring a brighter future for engineering firms.
Don’t miss out! Register today and secure your place at the ACEC 2024 Fall Conference, the premier event for engineering professionals!
October 10, 2024, 2:00 – 3:00 PM ET
CASE invites all members and firms interested in joining to attend the CASE Membership Meeting on October 10, 2024. This virtual meeting is a key opportunity for structural engineers to learn more about the coalition’s initiatives, stay informed about the latest developments in the industry, and network with peers.
Stay Updated on Industry Trends and Advocacy: CASE members will receive important updates on key industry trends, a legislative advocacy update from ACEC’s advocacy team, and hear how CASE is actively shaping the future of structural engineering. Hear how CASE is supporting firms in navigating current challenges, from risk management to emerging technologies.
Learn About CASE Publications and Resources: Learn about new and updated CASE publications, including essential risk management tools, contract templates, and professional practice guidelines. . Network and Collaborate: The CASE Membership Meeting offers an opportunity for firms to connect with other professionals in the structural engineering community. Attendees can share experiences, discuss challenges, and collaborate on solutions that strengthen the entire industry.
Don’t miss out on this chance to engage with the structural engineering community and learn how CASE can help elevate your practice. Register now and mark your calendars for October 10, 2024, at 2:00 PM ET!
For more information and to register, visit the ACEC website at www.acec.org.
Join us for a session focused on the importance of building documentation and the stakeholders involved. This session targets architects, engineers, contractors, and building owners engaged in design, construction, or maintenance. Participants will gain insights into how building documentation supports structural integrity, safety, and regulatory compliance. The session will cover the role of documentation in risk mitigation and its impact on the quality and longevity of built environments.
Highlights:
• Structural Integrity & Safety: Understand the role of building documentation in maintaining project safety.
• Compliance: Learn how thorough documentation helps ensure regulatory compliance and reduces legal risks.
• Quality & Longevity: Discover how effective documentation
practices enhance the quality and durability of built environments.
• Competitive Edge: Stay informed about industry developments to maintain a competitive advantage in building design and construction.
For more information about the session check out the podcast https://www.youtube.com/watch?v=xarZhHGIURs&t=125s.
The U.S. Institute of Building Documentation (USIBD) is a non-profit organization that promotes building documentation as an industry standard. USIBD works with professionals across various fields to encourage the use of documentation technologies and methods, aiming to improve efficiency and communication throughout the building lifecycle. The organization offers resources, education, and networking opportunities for its members.
Be sure to explore the latest CASE publications! If you’re not a CASE member, don’t forget to use your discount code, NCSEASEI2022, to save on your purchase.
Flexible work schedules and locations are a top priority for employees today. Many companies are navigating this shift and exploring long-term solutions, especially in structural engineering, where filling positions is increasingly challenging. Expanding candidate pools beyond local offices can yield quality hires, but this shift brings challenges in business, insurance, IT/security, productivity, training, and culture. This tool offers guidance in these areas.
Succession planning is a key aspect of talent management. It includes training, career planning, and replacement strategies. Effective succession planning helps organizations manage talent scarcity, identify skill gaps, promote knowledge transfer, boost employee morale, and build a robust skill set.
This tool provides guidelines for verifying analysis and design software results, focusing on linear elastic structures and small deformation/ small strain analysis, which are prevalent in design work.
Teaming agreements are essential for joint projects between contractors, design professionals, and structural engineers. These agreements outline the roles of parties before contract award and are often used in design-build projects. This commentary reviews standard teaming agreements from various organizations, including:
• AIA: Contract C102-2015
• EJCDC: Contracts D-580 and E-580
• DBIA: Contract No. 580
• Consensus Docs: Contracts 296 and 498
You can purchase these and other Risk Management Tools at CASE Resources, https://www.acec.org/member-center/get-involved/coalitions/case/resources/.
If you have suggestions for tools that could benefit your business, CASE welcomes your input! Contact coalitions@acec.org.
By Dr. Frank Griggs, Dist. M. ASCE
As early as 1868 an Act was passed in the Minnesota Legislature authorizing:
"…Be it enacted by the Senate and House of Representatives of the United States of America in Congress assembled, That the Southern Minnesota Railroad Company, a corporation existing under the laws of the State of Minnesota, is hereby authorized to construct and operate a railroad-bridge across the Mississippi River, between the city of La Crosse, Wisconsin, and a point opposite, in the State of Minnesota, with the consent of the legislatures of the States of Minnesota and Wisconsin, and said bridge, by this act authorized to be constructed, is hereby declared a post-route, and subject to all the terms, conditions, restrictions, and requirements, and entitled to all the privileges named in an act approved July 25, 1866, entitled “An act to authorize the construction of certain bridges and to establish them as post-roads."
The 1866 Federal Act had set standards for high level and low-level bridges. On April 1, 1872, that act was modified and on June 4, 1872, it was further modified as follows,
"AN ACT further regulating the construction of bridges across the Mississippi River.
Be it enacted by the Senate and House of Representatives of the United States of America in Congress assembled, That all bridges hereafter constructed over and across the Mississippi River, under authority of any act of Congress, shall be subject to all the terms, restrictions, and requirements contained in the fifth section of an act entitled “An act to authorize the construction of a bridge across the Mississippi River at or near the town of Clinton, in the State of Iowa, and other bridges across said river, and to establish them as post-roads,” approved April 1, 1872; and in locating any such bridge the Secretary of War shall have due regard to the security and convenience of navigation, to convenience of access, and to the wants of all railways and highways crossing said river."
and its Chief Engineer J. T. Dodge selected a site north of the city crossing the Black River over an island and then the Mississippi River “from the engine or round-house, in the Fifth Ward (formerly North La Crosse), across the lower end of French Island, to a point on the Minnesota bank, a short distance above the old warehouse, near the La Crescent Ferry landing. This site he had selected with sole reference to the desire of the company to secure the shortest and most direct route between Chicago and St. Paul, and without any special regard for the interests of other existing or contemplated railways, on either or both sides of the Mississippi, centering at or near La Crosse.” The railroad then applied to Congress to approve the location of their bridge. Gen. G. K. Warren, an expert on the Mississippi River, was in the process of reviewing the Mississippi River bridges and reported on June 29, 1872, “This location of the bridge and draw is not objectionable to navigation on the Mississippi River, provided the proper works are built to always maintain the channel through the draw.”
The city of Lacrosse and others, however, wanted the line to run through the city and cross the river and petitioned the Congress to intervene. The Minnesota Southern Railroad also wanted a crossing south of Lacrosse. A Board of three Army engineers was appointed and interpreted the June 18, 1872, law as follows, “This would seem to require that all future railway bridges over the Mississippi shall also be highway bridges. As it is not so stated directly, the board thought it best to get an official interpretation of the meaning of this act, and therefore telegraphed the Chief of Engineers for such interpretation.” A. A Humphreys sent a letter to the Secretary of War stating, “a question has been raised as to the meaning of the act of June 4,1872, a copy of which is enclosed herewith. It is claimed by some that the act in question requires that all future bridges over the Mississippi River shall be highway as well as railway bridges. I beg leave to suggest that the law be submitted to the Attorney -General for his opinion in the case.”
The last lines added a new consideration for the Secretary of War to consider, namely “security and convenience of navigation, to convenience of access, and to the wants of all railways and highways crossing said river.” By 1872 the Chicago, Milwaukee & St. Paul had a line connecting Chicago and St. Paul, as well as a line from Lacrosse on the Mississippi to Milwaukee and used the Winona Bridge. Lacrosse was across the river from LaCresent, Minnesota, where there was a line from St. Paul. It looked for a way to cross the river at Lacrosse
The case was sent to Department of Justice and the Attorney General wrote in part on August 7, 1872, “Moreover, had Congress intended so important a modification of previous acts as a requirement that the bridges in question should all be constructed for both railways and highways, it is hardly to be supposed that it would have been content to make so vague a provision for the purpose as the words of this clause would constitute…It would, in fact, be leaving interests of very great value and consequence to the chances of a doubtful implication and as most of these bridges are authorized to be built by railway companies interested only in the accommodation of their own business, it is not to be presumed, in the, absence of explicit provision to that effect, that Congress meant to burden them with the heavy additional expense
that would have to be incurred to accommodate their bridges to the uses of common highways or wagon-roads. The true intent of the provision of the act relative to the location of bridges is, it seems clear, to define more particularly the duty of the Secretary of War in making such locations.” In other words the Board of Engineers had misinterpreted the intent of the legislation.
A letter was sent to the Chief of Engineers stating, “In view of the opinion of the honorable Attorney-General, dated August 7, 1872, regarding the construction of the laws relating to bridges across the Mississippi River, whether, under act of June 4, 1872, all such bridges as are therein referred to are required to be highway as well as railway bridges, and the fact that this opinion was not received in time to be laid before the Board of Engineers which has recently made a report upon the question of location of bridge or bridges at or near La Crosse, Wis., and that the board has entertained a construction of the law different from that of the Attorney-General, it seems proper that it should be reconvened to reconsider the subject. It is, therefore, respectfully recommended that the board be reconvened, and with your sanction it will be so ordered.”
The Board of Engineers was reconvened in September 1872 to consider their recommendation for the bridge site given the opinion of the Attorney General. Their report was presented to the Chief of Engineers with the recommendation, “After a full consideration of all that has been urged by all the parties in interest, the board feel constrained to adhere to their original decision, that the only bridge that should be permitted across the Mississippi at or near La Crosse should be at the foot of Mount Vernon street." They also wrote, “It was argued on the part of the Milwaukee and Saint Paul Company that we had no right to consider the future and its probabilities, but must control our decision by the wants of existing interests only. For the reasons given above, the board entirely disagree with this view. We believe that we must anticipate the future, and try to foresee it, so that it may be provided for now, and thus the need of another bridge near La Crosse be obviated.” Humphreys agreed and on October 11, 1872, a letter was sent to the Secretary of War with this opinion stating, “in the views of the board I concur, and recommend to your favorable consideration and approval the site selected at the foot of Mount Vernon street.”
An attempt was made to repeal the June 4 Act giving the Secretary of War power to control matters other than clearances but this failed. The City of Lacrosse gave the railroad the land at the foot of Mount Vernon Street in February 1873. The Wisconsin legislature passed, over the Governor’s veto, a bill to have the bridge at the lower site and the War Department approved this site.
In spite of these reversals the railroad started building its bridge at its proposed site. The Attorney General to test his conclusion asked for an injunction in the United States District Court that stated, “It is hereby ordered, adjudged and decreed that an injunction be issued pursuant to the prayer of the bill herein strictly forbidding, enjoining and restraining the said defendant, the Milwaukee & St. Paul Railway Company, its agents, contractors, laborers, servants and officers, and each and every one of them, under the pains and penalties which may fall upon them, from placing any stone, piling or bridge materials, and from proceeding to construct any bridge in or across the Mississippi River in the vicinity of the city of La Crosse, in said Western District of Wisconsin, at or near the locality described in the bill of complaint in this suit, until further order of the Court."
The judges in hearing this injunction agreed with the railroad stating, “Had we to decide, we should say that their location is far
less injurious to navigation than the one indicated by the Secretary of War, and that it is equally as convenient and accessible to other railroads and highways.” The injunction was lifted and on March 6, 1874, the Wisconsin Legislature passed a bill that “gave the authority to railroad to bridge the Mississippi where ever they chose.” The War Department, still dragging its feet, did not authorize construction at the site until late 1875.
As was usual process at the time, the company requested proposals from several bridge companies who submitted their designs in accordance with the specifications prepared by the railroad. The American Bridge Company received the contract to fabricate and erect all the bridges. Instead of their usual Post Truss they submitted designs using Pratt trusses and a variation of a Pennsylvania Truss. Their swing span, with its 360-feet length was patterned after the design the Kellogg Bridge Company had prepared for the Louisiana Bridge.
Work began in January 1876 but, due to flooding, etc., work was not resumed until June 10, 1876. Their bridge was just over a mile and ¾ long from the easterly banks of the Black river across Campbell’s island to the westerly banks in Minnesota. Over the eastern channel there were five spans of 150 feet. This was followed by 660 feet of wooden trestle across the island. Across the westerly channel there were two spans of 164 feet from the west bank of the island, one span of 250 feet and a swing span of 360 feet with the swing span next to the Minnesota shore. The 164-feet spans were Pratt Trusses and the 250-feet span a broken chord Pennsylvania Truss.
Construction started on June 10, 1876. The contract for the design and construction of the superstructure was signed in early July and the bridge was opened on November 28, 1876.
A History of La Crosse County concluded, “There is nothing particularly showy about the entire fabric, but everything is constructed in a handsome and substantial manner, that does credit not only to the company, but to the contractors who so faithfully performed their work. The contract test of the bridge was made by a double-loaded freight train, and the strain was found to be remarkably sustained by every portion. The completion of this magnificent structure represents a total cost of over half a million of dollars."
It was replaced in 1902 with steel spans. Plans have been proposed to replace the swing span with a lift span, but they have not been approved to date. ■
Dr. Frank Griggs, Dist.M. ASCE, specializes in the restoration of historic bridges, having restored many 19th Century cast and wrought iron bridges. He is now an Independent Consulting Engineer (fgriggsjr@verizon.net).
Questions you always wanted to ask.
By Jennifer Goupil, PE
This quarterly article addresses some of the questions received about structural standards developed by the Structural Engineering Institute (SEI) of the American Society of Civil Engineers (ASCE). Questions from engineers, building officials, and other design professionals are often considered to develop future editions.
This month includes many topics about the code adoption process for ASCE referenced standards. These topics and more are discussed on the ASCE Peer-to-Peer Standards Exchange Forum. ASCE/SEI members can ask and answer questions in the forum. Visit https:// collaborate.asce.org/standards-exchange/home to learn more and read about other topics.
I often notice the Call for Members for SEI Standards Committees but cannot figure out the timing. It seems random and I do not know when to expect it. I do not want to miss the opportunity to apply to join a committee because I do not understand the timing. Can you explain the process and timing?
I have heard that ASCE 11 is being updated, but that there is also a new Manual of Practice (MOP) for structural condition assessment. What is the difference in these two documents? That is correct! ASCE/SEI 11 Guideline for Structural Condition Assessment of Existing Buildings has started its revision cycle. The current edition of ASCE/SEI 11 is the 1999 edition, which is long overdue for an update. Recently ASCE published ASCE/SEI MOP 158: Structural Condition Assessment of Existing Building: A Guideline, which is described as “a more user-friendly, accessible format for utilization by qualified professional structural engineers and regulatory officials concerned with existing structures.” The MOP 158 was developed and published because ASCE/SEI 11-99 needed to be updated, including new references, but since ASCE/ SEI 11-99 is a guideline and not written in mandatory language, the content was most appropriate to be updated as a peer-reviewed manual of practice. So think of MOP 158 as an update of the guidelines in ASCE/SEI 11-99. Presently, the ASCE/SEI 11 Standards Committee is updating the content of ASCE/SEI 11 and developing it as a mandatory standard. Among others, one can understand the difference between mandatory provisions and guidelines in the language used. Standards that include “shall” and “must” are mandatory, while the inclusion of “should” and “may” are considered guidelines (or non-mandatory). At SEI, mandatory, consensus standards development is governed by ANSI-accredited ASCE Rules. Mandatory language can be more easily regulated, enabling jurisdictions to enforce consistency across projects. Guidelines and Manuals of Practice represent best practices and are written and reviewed by subject matter experts outside of a consensus process. Both are relevant and appropriate for different applications. So while the title of each document include structural condition assessment, the type of document is determined by the language used and development process.
This article’s information is provided for general informational purposes only and is not intended in any fashion to be a substitute for professional consultation. Information provided does not constitute a formal interpretation of the standard. Under no circumstances does ASCE/ SEI, its affiliates, officers, directors, employees, or volunteers warrant the completeness, accuracy, or relevancy of any information or advice provided herein or its usefulness for any particular purpose. ASCE/SEI, its affiliates, officers, directors, employees, and volunteers expressly disclaim any and all responsibility for any liability, loss, or damage that you may cause or incur in reliance on any information or advice provided herein. If you have a question you want to be considered in a future issue, please send it to sei@asce.org with FAQ in the subject line. Visit asce.org/sei to learn more about ASCE/SEI Standards. ■
This is great question. The answer is twofold: each standard is on a development cycle that aligns with its applicable, referenced, regulatory cycle (yet applications are accepted on a rolling basis) and new applications are considered for the next cycle shortly after publication. First, the timeline for each standard depends on where is it adopted. Generally speaking, if an ASCE/SEI standard is adopted by reference into any of the International Code Council’s (ICC) I-Codes—such as the International Building Code (IBC) or the International Existing Building Code (IEBC)—the standard development cycle and publication is timed to align with the development cycle for the I-Codes, which for ASCE/SEI standards occurs every six years. ASCE 7, ASCE 41, ASCE 24 and many other ASCE/SEI Standards follow this timeline. Other standards are developed on a five-year cycle to align with the ANSI Requirements — the accredited process that ASCE follows for consensus standard development. This determines when the cycles get started, however, new applications are typically evaluated and accepted at the beginning of each new development cycle, which occurs several months following the publication of the document. Additionally, many committees will accept associate members up until the first committee ballot, which could take a year or more. Having said all this, you should know that the application process is always open on the SEI website and will accept submissions on a rolling basis. So, for example, ASCE 7 is underway and not currently accepting new members, however the application site is always open. If you are interested in the next cycle, you can apply today or anytime over the next few years, and your application will be kept on file until the next cycle and then evaluated with all others received. In summary, while SEI makes active announcements for “Call for Members” during the beginning of each cycle, you can apply at any time to be considered for the next cycle of an ASCE/SEI Standard committee. To apply anytime, use this QR Code to find the ASCE Standards Committee Application page, select “SEI” in the drop down and select the standard.
Jennifer Goupil, PE, F.SEI, F.ASCE, is the Managing Director of the Structural Engineering Institute and the Chief Resilience Officer for the American Society of Civil Engineers.
Industry Resources for SEs and
Profiles from STRUCTURE’s
Advertising Partners
From the original rebar tying tool, the RB262 in 1993, every generation of MAX rebar tying tools brings greater efficiency and productivity to job sites around the world. Building upon the success of its predecessors, the RB443T sets a new standard in rebar tying technology with its unparalleled capabilities and enhanced performance features. Since the initial announcement of the TWINTIER® platform in 2017, MAX has continuously pushed the boundaries of innovation, introducing groundbreaking tools such as the RB441T, RB611T, and the world’s first stand-up rebar tying tool the RB401T-E. Now, with the release of the RB443T, MAX reaffirms its commitment to providing cutting-edge solutions to the construction industry. Our newest platform, TWINTIER®, features three new innovative mechanisms that have raised the bar. These features include:
NEW FASTER TYING: The RB443T tying speed was reduced to less than 1/2 second—approximately 40% faster than the RB441T.
NEW LOAD ASSIST MECHANISM: New wire loading system automates wire loading for tool.
NEW EASY PULL TRIGGER: Reduced trigger effort is required, improving operational ergonomics.
BUIILT TO LAST: The TWINTIER® is built to last with durability that you can count on time and time again with MAX.
COST SAVINGS: The TWINTIER’s Wire Pull-Back Mechanism dispenses the precise amount of wire needed to form a tie, reducing wire usage.
The MAX TwinTier® is not limited to the standard applications. Over 30 years we have become a versatile tool. We started as the go to for tying rebar at precast plants, road and bridges, and tilt-up locations. Since then, we have been introduced to a multitude of markets such as electrical conduit, radiant tubing, and maple syrup lines. We are looking forward to seeing what other applications our users will use.
www.maxusacorp.com/rebar_tying_tools/
Computers and Structures, Inc. develops software for structural and earthquake engineering. CSI’s software is backed by more than four decades of research and development, making it the trusted choice of sophisticated design professionals everywhere.
CSI is based in Walnut Creek, California, with offices in New York, Houston, Madrid, New Delhi, and the Caribbean.
Through the 1960s and into the 1970s, the University of California at Berkeley campus attracted some of the most brilliant minds in structural engineering. With the space program in full swing and nuclear power plants cropping up all over the country, it was not surprising that UC Berkeley became a hotbed of innovation that resulted in some of the most state-of-the-art computer solutions for complex engineering problems.
In 1970, following the development of a series of many unique and innovative numerical methods and algorithms, Dr. Edward L. Wilson, a distinguished professor of structural engineering at UC Berkeley, released the first comprehensive structural analysis computer program – called “SAP: A General Structural Analysis Program.” A revolutionary product that was destined to change the trajectory of engineering analysis forever!
Despite the availability of this sophisticated technology, it was not widely used by structural engineers initially. Users were mostly limited to government organizations and large companies.
It took a young Berkeley structural engineering graduate, Ashraf Habibullah, to realize that someone needed to deliver the technology to the field.
“All this research was being done on campus, but none of it was reaching the profession. So there was a gap between what the researchers were producing and what the professionals could productively use in practice. I knew I could bridge this gap,” said Ashraf.
Habibullah approached Wilson and, with his help, created a comprehensive course at UC Berkeley Extension on computeraided structural analysis and design geared toward the practicing structural engineer, with a strong focus on earthquake engineering. The 12-lecture series generated an overwhelming response from the profession. Indeed, there was so much interest that the class had to be moved to a larger venue to accommodate the surge of registrants. Soon after, with growing interest in the technology, Habibullah and Wilson made presentations to packed audiences across the country.
In 1975, Habibullah founded Computers & Structures, Inc. (CSI) and positioned the company as a technology transfer
link between researchers and professionals, producing practical products based on Dr. Wilson’s original research and work.
The advent of personal computers in the late 1970s allowed smaller companies and individuals to access CSI’s powerful technology. This fueled the proliferation of the software worldwide, especially in developing nations. In 1979 Habibullah and Wilson produced the first 3-D structural analysis modeling application for personal computers. Its powerful numerical methods for earthquake engineering, integrated with new graphics and animation, permanently changed the course of the structural engineering profession.
Over the years, CSI has pioneered many developments in algorithms for structural engineering and Finite Element Analysis technology that have addressed challenges that engineers have struggled with for decades. Using the latest and most advanced technology, CSI develops powerful software that directly addresses the needs of the structural engineering community. CSI products are the preferred teaching and research tools in thousands of universities and research organizations, including UC Berkeley, Stanford, Princeton, and MIT. In addition, CSI provides free software to academic institutions in many countries – including Indonesia, Peru, Turkey, and Pakistan –to aid developing countries, specifically in earthquake-prone regions, in producing seismically-resistant structures.
Thousands of engineering firms use software from CSI in over 160 countries to design significant projects, including the Taipei 101 Tower in Taiwan, One World Trade Center in New York, the 2008 Olympics Birds Nest Stadium in Beijing, and the cablestayed Centenario Bridge over the Panama Canal.
CSI produces five primary software packages: SAP2000, CSiBridge, ETABS, SAFE, CSiPlant , and PERFORM-3D
RISA Technologies
Phone: 949-951-5815
Email: info@risa.com
Web: risa.com
Product:RISA-3D
Description: RISA offers everything you need for concrete design. For concrete floors, including beams and two way slabs, nothing beats RISAFloor ES for ease of use and versatility. The design of columns and shear walls with RISA-3D offers total flexibility. Integration between RISA-3D and RISAFloor ES provides a complete building design.
CTS Cement Manufacturing Corporation
Phone: 800-9293030
Email: jong@ctscement.com
Web: www.ctscement.com/
Product: Rapid Set® Cement
Description: Rapid Set® Calcium Sulfoaluminate (CSA) Cement is used in a full line of professionalgrade cement products engineered for high performance, rapid strength gain, versatility, low shrinkage and durability. Rapid Set Cement achieves structural strength in one to two hours. Save time, money, and achieve durable, long-lasting results with Rapid Set.
Altair
Phone: 604-273-7737
Email: manishaw@altair.com
Web: https://www.altair.com/s-concrete/ Product: Altair S-CONCRETE
Description: S-CONCRETE quickly and accurately designs reinforced concrete column, beam, wall sections, and continuous beams to regional code requirements. Versatile and easyto-use for any project workflow, users save time by automatically checking thousands of concrete designs at once to produce comprehensive design reports.
RISA Technologies
Phone: 949-951-5815
Email: info@risa.com
Web: risa.com
Product: ADAPT-Builder
Description: ADAPT-Builder is powerful and easy-to-use 3D finite element software for multistory reinforced concrete and post-tensioned buildings and structures. Builder delivers comprehensive workflows for complete analysis and design. Combine gravity, lateral and post-tensioning actions for efficient, complete, and accurate design. Integrate with various BIM software for seamless project deliverables.
STRUCTURAL ENGINEERING Resource Guide
ASDIP Structural Software
Phone: 407-284-9202
Email: support@asdipsoft.com
Web: https://www.asdipsoft.com
Product: ASDIP CONCRETE
Description: Software for the design of multi-span continues beams, biaxial slender columns, concrete/ masonry bearing walls, shear walls and one-way slabs, per the latest design codes. ASDIP Concrete easily integrates with ETABS and other software to effortlessly maximize your designs with a few clicks.
DYWIDAG
Phone: 630-739-1100
Email: sales.us@dywidag.com
Web: www.dywidag.com
Product: DYWIDAG High-Strength
Reinforcement THREADBAR Systems
Description: DYWIDAG THREADBARS for significant savings versus conventional reinforcement. Realize less congestion and stronger reinforcement. Reduce the number of bars, enable easier concrete placement and smaller columns resulting in more usable/sellable floor space. Threaded bars and accessories for fast/easy construction. Use GR100 for up to 65% weight and 35% labor reduction.
Enhance Project Design and Delivery with More Sustainable Cements
CTS produces two lower-carbon alternatives to portland cement for concrete construction and repair: Rapid Set® Cement and Komponent® expansive cement additive.
Both brands are a type of calcium sulfoaluminate (CSA) cement, which emits 27% less CO2-eq during manufacture than portland cement. A Type III environmental product declaration (EPD) is available for each brand to help project teams meet the increasing demand for low-embodied-carbon (LEC) construction materials.
The core ingredient in dozens of cements, mortars, and grouts, Rapid Set® cement qualifies as very rapid hardening (VRH) per ASTM C1600 (Standard Specification for Rapid Hardening Hydraulic Cement). Unlike other CSA cements, Rapid Set® doesn’t require blending with portland cement or accelerators to achieve structural strength in 1 hour.
Mixed, placed, and finished similarly to portland cement products, Rapid Set® products shorten construction time and lessen maintenance requirements. They’re more resistant to deterioration caused by chlorides, alkali-silica reaction (ASR), sulfate attack, and shrinkage cracking. Project teams benefit by quickly putting buildings and infrastructure back into service and delivering durable, long-lasting results.
Komponent® is blended with local portland cement or portland-limestone cement (PLC) and aggregate to make Type K shrinkage-compensating concrete, low-shrinkage concrete, and non-shrink grout. In addition to lowering concrete’s global warming potential (GWP), the additive simplifies design and construction by maximizing placement sizes and minimizing or eliminating control joints, waterstops, and pour strips.
Our products have been used to build and repair landmarks like the Hoover Dam Bypass, Pentagon, Lincoln Tunnel, and San Francisco-Oakland Bay Bridge. Research by public agencies nationwide confirm the durability of decades-old placements on airport runways, highways, and other infrastructure.
Our material scientists, engineers, technical experts, and field representatives will work with you every step of the way, from design through construction, to ensure your success. Contact us for the Rapid Set® and Komponent® Type III EPDs or for assistance with product selection, specifications, samples, and mix designs.
800-929-3030 | info@ctscement.com | www.CTScement.com
Revolutionizing Construction With Cutting-Edge Solutions
With decades of experience collaborating with structural engineers, Simpson Strong-Tie has honed a deep understanding of the construction industry’s needs. Our technical expertise allows us to develop advanced products for structural reinforcement, designed to help fabricators, erectors, engineers, and contractors enhance efficiency and resilience during the building process.
Introducing Quick-Lock® and Quick-Lock HD® Pier Wheels & Boots
The Quick-Lock® and Quick-Lock HD® Pier Wheels & Boots are engineered to ensure consistent concrete cover and alignment for deep foundations. These innovative products provide stability and support for drilled shafts and other applications where rebar alignment is crucial.
Key Features and Benefits
• Precision Engineering: Our rebar spacers ensure precise spacing and alignment, guaranteeing consistent concrete cover.
• Ease of Installation: Designed for quick and straightforward installation, these spacers significantly reduce labor time and costs.
• Durability: Constructed from high-quality, durable materials, these spacers are built to stay in place.
• Secure Placement: They securely hold rebar in place during concrete pouring, preventing any movement.
• Versatility: Capable of accommodating various rebar sizes and configurations, these spacers offer a reliable and economical solution for maintaining proper rebar cover.
• High Precision: Ensures consistent and accurate rebar placement, contributing to the overall safety and integrity of the structure.
• Compatibility: Ideal for use with large diameter rebar in heavy-duty construction projects.
Unmatched Service and Support
Simpson Strong-Tie’s reinforcement alignment products are readily available through our nationwide distribution network and are backed by industry-leading technical and field support. As a trusted partner in structural reinforcement, we provide builders with innovative products, technology, and services to maximize efficiency and resiliency at every stage of the engineering and construction process.
To learn more about Simpson Strong-Tie Reinforcement Alignment Products, visit strongtie.com/ra.
For inquiries, call 800-999-5099.
Quikspray Inc.
Phone: 419-732-2611
Email: sales@quikspray.com
Web: https://quikspray.com
Product: Quikspray Inc. 1.5-Inch Grout Plant
Description: Quikspray®’s Carrousel Pumps, U-Blend mixers, and Aggregate (AG) Broadcasters have you covered when it comes to pumping and spraying high solids epoxy and cement formulas. Quikspray® equipment is involved in new and restorative structural projects like rehabilitating bridges and buildings, offshore structures, and even building amusement park rides.
Nucor Vulcraft
Phone: 402-844-2400
Email: marketing@vulcraft.com
Web: https://vulcraft.com
Product: Flush Frame Connections
Description: Vulcraft’s Flush Frame Connections can enhance and modernize your steel floor joist systems, providing reduced floor vibrations, faster deck and MEP installation, elimination of blocking between joists, and lower floor-to-floor heights.
Technidea Corporation
Phone: 760-480-4740
Email: info@technideacorp.com
Web: www.ziplevel.com
Product: ZIPLEVEL EZDepth for Excavators
Description: ZIPLEVEL EZDepth is an inexpensive alternative to costly cab-based digital depth instruments at 1/10 conventional costs. Now you can enjoy the cost and labor savings, work quality and safety benefits of instant, Easy Depth readings from the cab without a laser and rodman. For more information, visit www.ziplevel.com/ezdepth.
Strong Tie
Phone: 800-999-5099
Web: https://www.strongtie.com
Product: HTT Heavy-Tension Ties
Description: Simpson Strong-Tie has added the HTTH6.5 to its line of HTT heavy-tension ties for post-pour, wood-to-concrete connections. The industry’s first nailed-down holdown to achieve a 6,500-lb. allowable load, the HTTH6.5 bridges the gap between the company’s HTT5 heavy-tension tie and HDU8 holdown, providing an economical choice when heavier loads are required.
EWilliams Form
Engineering Corp.
Phone: 616-866-0815
Email: williams@williamsform.com
Web: www.williamsform.com
Product: Anchor Systems
Description: Williams Form Engineering Corporation has been providing threaded steel bars and accessories for rock anchors, soil anchors, high capacity concrete anchors, micropiles, tie rods, tiebacks, strand anchors, hollow bar anchors, post tensioning systems, and concrete forming hardware systems in the construction industry for over 100 years.
Hohmann & Barnard
Phone: 800-645-0616
Web: www.h-b.com
Product: Thermal Wing Nut Anchor
Description: Hohmann & Barnard’s 2-SEALTM
Thermal Wing Nut Anchor is an innovative, single screw veneer tie for metal stud construction. It features a dual-diameter barrel with factory-installed EPDM washers to seal both the face of the insulation and the air/vapor barrier, and unique Thermal Wings designed to decrease thermal transfer through rigid insulation.
liminate pour strips, wall restraint, and expansion joints – and the extra costs, construction delays, and safety issues they bring – with the PS=Ø® Mechanical Reinforcement Splice System.
The PS=Ø® Mechanical Reinforcement Splice System eliminates pour strips, wall restraint, and expansion joints while allowing for volume change in the slab and providing structural integrity. Using proven coupler technologies recognized worldwide, the PS=Ø® system features a thread on one end and a grout-filled sleeve on the other. The system is an ACI-permitted Type 1 and Type 2 mechanical splice, ICC-approved, and made in the USA.
Pouring back pour strips is the most expensive concrete poured on a job. Formwork, shoring, and backshoring must stay in place for weeks, and crews must reassemble for small pours. The PS=Ø® system eliminates this costly and time-consuming step.
Pour strips delay construction schedules. They not only require pouring back the leave-out, but also restrict worker access. By eliminating pour strips, the PS=Ø® system cuts months off construction schedules.
An open leave-out in a floor is a major safety hazard. Pour strips are particularly hazardous because they run the entire width of the slab and are impossible to avoid. The PS=Ø® system replaces dangerous leave-outs with a narrow, grouted joint.
Without needing an open pour strip leave-out, engineers can now achieve higher quality concrete by specifying longer leave-out times, releasing stiff elements without causing delays or increasing cost.
Since 1933, Hohmann & Barnard, Inc. has been at the forefront of delivering innovative solutions for building facades. As a global leader in manufacturing construction materials for the building envelope, we specialize in anchors and reinforcement, moisture protection, restoration anchors, thermally efficient systems, air/vapor barrier systems, rainscreen support systems, and custom-engineered solutions tailored to the needs of masonry contractors.
Our product lineup includes industry-leading thermal performance solutions designed to enhance the energy efficiency of your building projects:
• Thermal Brick Support (TBS) System: Designed to minimize thermal bridging in shelf angles, the TBS System improves energy efficiency by allowing for continuous insulation behind the shelf angle. This innovative bracket system is job-specific, engineered to move the shelf angle away from the wall, reducing labor costs and maximizing energy efficiency.
• HB RS EZclad Rainscreen Support System: Fully engineered to project specifications by our expert team, this system utilizes stainless steel structural components to reduce conductivity and enhance thermal efficiency. Key benefits include:
o Inward and outward adjustability
o Uninterrupted continuous insulation
o Optional thermal shims for additional thermal efficiency
o Customizable coating or anodizing for aesthetics
o Comprehensive package including structural connection, rail, engineering calculations, and shop drawings
• HB RS FLEXbracket Rainscreen Support Bracket: A versatile stainless-steel bracket designed to accommodate various insulation depths, the FLEXbracket works with multiple aluminum rail systems for both vertical and horizontal applications. Features include:
o Optional thermal shims for enhanced energy efficiency
o Reduced thermal transfer
o Dual friction fingers for easy installation
o Adjustable, engineered system for precise alignment
• 2-SEAL™ Thermal Wing Nut Anchor: This single-screw veneer tie is available for metal studs, concrete, CMU, and wood. It features thermally isolated wings made from steel encapsulated in flame-resistant plastic, creating a thermal break. The anchor’s EPDM washers provide a dual seal at the insulation and air/vapor barrier, and the anchor is designed to easily orient pintles/hooks parallel to masonry joints.
In addition to our thermal solutions, we also offer innovative structural systems to enhance the aesthetics and functionality of your projects:
• Concealed Lintel System: Elevate the aesthetic appeal of your project with our Concealed Lintel System, available in Surface Mounted, Flat Spine, or Arch Spine configurations. We provide a complete set of drawings and calculations, along with all necessary components and expert support from our Engineering Team, ensuring a seamless, maintenance-free installation.
At Hohmann & Barnard, we are committed to helping you engineer complete wall systems that ensure the full integrity and longevity of your building façade/masonry projects
Phone: 800-424-2252
Email: info@enercalc.com
Web: https://enercalc.com
Product: ENERCALC SEL/ENERCALC 3D
Description: ENERCALC automatically incorporates seismic loads in load combinations, including the vertical component, redundancy & system overstrength factors, as applicable. ENERCALC supports ASCE 7’s Base Shear, Demands on Non-Structural Components & Wall Anchorage. ENERCALC also includes earth retention wall modules - including substantial segmental wall improvements, & ENERCALC 3D FEM.
Bentley Systems
Web: www.bentley.com
Product: Structural Work Suite
Description: Reduce design time by 30% with Bentley Systems’ Structural WorkSuite, a single solution set for concrete and steel. See how this costsaving bundle of eight industry-leading structural engineering solutions can improve your workflows. Get your free e-book.
Nucor Vulcraft Verco
Phone: 402-844-2400
Email: marketing@vulcraft.com
Web: https://vulcraft.com/
Product: PunchlokII® System
Description: Our steel roof deck and PunchLok II® System are valuable options if seismic or highwind challenges are important considerations for your next project. Our team of experts can help you evaluate sustainable solutions offered by Vulcraft and Verco to ensure the right system is in place.
RISA Technologies
Phone: 949-951-5815
Email: info@risa.com
Web: risa.com
Product:RISA-3D
Description: Feeling overwhelmed with seismic design procedures? RISA-3D has you covered with seismic detailing features including full AISC-341/358 code checks. Whether you’re using RISA-3D’s automated seismic load generator, or using the builtin dynamic response spectra & time history analysis/ design capabilities, you’ll get designs and reports that meet all your needs.
SAFI Structural Engineering Software
Phone: 800-810-9454
Email: sales@safi.com
Web: www.safi.com
Product: SAFI GSE Software
Description: Discover GSE – the ultimate multimaterial analysis and design software for structural engineers. With GSE, you can optimize your workflow by designing in multiple materials—steel, concrete, wood, and aluminum—simultaneously. Supported by 38 years of continuous development, GSE stands as a leader in innovation for the construction industry.
STRUCTURAL ENGINEERING Resource Guide
F2025 Resource Guide forms (monthly and annual) are now available on our website.
or steel moment connections, DuraFuse Frames has your back! Whether design is controlled by wind or seismic demands, a DuraFuse project will have the lowest steel tonnage, simple fabrication, and a rapid, safe erection schedule (fully field-bolted). DuraFuse engineers support your team and provide an optimized design that minimizes overall frame construction cost resulting in a sustainable steel solution for any project. Our prequalified seismic connection (AISC 358-22 and OSHPD/HCAI PCS-0004) adds resilience through repairability and eliminates multiple onerous seismic detailing requirements, saving materials and time on the site. Our connections also work for retrofit designs that are
economical and can be tailored to challenging existing nonductile connections. Ensure your next project is economical, sustainable, and resilient.
DuraFuse engineers are constantly improving the modeling, analysis, and design process to ensure efficient, high performance, and resilient design solutions with quick response times. We are happy to provide a complimentary design alternative using DuraFuse Frames based on your specifications. Distinguish your next steel moment frame design by improving economy, constructability, and repairability and adding value for your clients. You can have the best of all worlds with DuraFuse Frames.
Record-Setting Mass Timber Building Stands Tall After Dramatic Shake Table Quake
Simpson Strong-Tie’s longstanding partnerships with leading universities and industry collaborators pays off with the success of the NSF-funded NHERI TallWood Project.
With decades of experience in structural engineering, Simpson Strong-Tie has honed a deep understanding of the construction industry’s needs.
Simpson Strong-Tie, the leader in engineered structural connectors and building solutions, announced in May, 2023, the successful completion of a series of seismic tests on the tallest full-scale building ever tested on an earthquake simulation shake table. Tests on the 10-story mass timber structure were conducted as part of the Natural Hazards Engineering Research Infrastructure (NHERI) TallWood Project, a research project funded by the National Science Foundation and building industry partners to prove the strength and seismic resiliency of mass timber as a low-carbon structural building material.
Seismic tests simulating both the 1994 magnitude 6.7 Northridge earthquake and the 1999 magnitude 7.7 Jiji earthquake were conducted at the Englekirk Structural Engineering Center at the University of California San Diego (UCSD), home to North America’s largest outdoor shake table and one of the two biggest earthquake simulators in the world, with a capacity of carrying and shaking structures weighing up to 2,000 metric tons across six axes of movement.
In addition to UCSD, a consortium of universities collaborated on the NHERI TallWood project, including the Colorado School of Mines; the University of Nevada, Reno; Colorado State University; the University of Washington; Washington State University; Oregon
State University; and Lehigh University. The project also received support from the U.S. Forest Service and the USDA Forest Products Laboratory.
Innovation was a key component of the project. The ten-story structure features a new rocking wall lateral system designed for resilient performance, meaning the building will have minimal damage from design-level earthquakes and be quickly repairable after rare earthquakes.
To connect gravity systems and lateral systems, Simpson Strong-Tie developed a new class of beam-to-column connections and column-to-foundation connections that were as resilient as the rocking timber wall structure. For construction and testing, Simpson Strong-Tie donated mass timber fasteners, angled washers, diaphragm spline straps, cold-formed steel connectors and anchor tiedown systems, then supervised the proper installation of those products.
In 2017, Simpson Strong-Tie collaborated with the project team to test a two-story mass timber building by simulating the Northridge earthquake. In addition to demonstrating that mass timber building systems can be seismically resilient, those tests helped the research team develop the design and analysis methods that were used for the 10-story building. Simpson Strong-Tie also conducts seismic research on earthquake simulators located at the company’s Tyrell Gilb research facility in Stockton, California.
During the tests, an array of sensors measured the impact of seismic forces across a variety of building systems. In addition to the rocking wall lateral system, the building features four exterior façade assemblies, a number of interior walls, and a 10-story stair tower.
The full findings of the construction and testing of the building are expected to support continued adoption of mass timber as a strong and versatile building material for residential and commercial structures in areas prone to seismic activity.
To learn more about mass timber solutions from Simpson Strong-Tie, download our Connectors and Fasteners for Mass Timber Construction catalog at go.strongtie.com/masstimber.
ASDIP Structural Software
Phone: 407-284-9202
Email: support@asdipsoft.com
Web: https://www.asdipsoft.com
Product: ASDIP RETAIN
Description: Software for quick and efficient design of cantilever, restrained, counterfort, and sheet pile retaining walls. See immediate results with calculations and reports of load combinations per the latest IBC/ASCE 7 and AASHTO. Includes 4 intuitive modules to help you design and verify structural members in no time.
Phone: 559-349-0138
Email: nheri-simcenter@berkeley.edu
Web: https://simcenter.designsafe-ci.org/
Product: SimCenter Software
Description: The NHERI SimCenter offers advanced software tools for engineers to quantify uncertainties within their simulations on the performance of buildings and infrastructure under natural hazards. Our applications facilitate a range of capabilities from CFD, to risk evaluation, and performance-based engineering to mitigate damage from earthquakes, hurricanes, and other extreme events.
Nucor Vulcraft Verco
Phone: 402-844-2400
Email: marketing@vulcraft.com
Web: https://vulcraft.com/
Product: Online Design Tools
Description: Our comprehensive suite of online design tools puts the power in your hands when specifying products, leading to improved performance and reduced costs. These tools assist in selecting the most cost-effective Vulcraft and Verco steel products for your project, including joists, roof deck, floor deck, composite deck, and Dovetail deck.
Phone: 267-702-2815
Email: info-us@dlubal.com
Web: www.dlubal.com
Product: RFEM 6
Description: Discover RFEM 6, the cutting-edge structural analysis and design software! With advanced technology, effortlessly create mass timber, concrete, steel, aluminum, cold-formed steel, steel connections, cable, tensile membrane, and CFD wind analysis structures according to USA and international standards. Get your free 90-day trial at www.dlubal.com now!
FRISA Technologies
Phone: 949-951-5815
Email: info@risa.com
Web: risa.com
Product: RISA-3D
Description: With RISA-3D’s versatile modeling environment and intuitive graphic interface, you can model any structure from bridges to buildings in minutes. Get the most out of your model with advanced features such as moving loads, dynamic analysis, and over 40 design codes. With RISA, structural design has never been so thorough or easy.
LLC
Phone: 800-424-2252
Email: info@enercalc.com
Web: https://enercalc.com
Product: ENERCALC SEL / ENERCALC 3D
Description: ENERCALC SEL is: Diverse calculation types and materials. Easy tabular navigation. Rich responsive graphics. Simple intuitive inputs. Detailed calculations. Project based organization. 41 different component type designers. Current/historical Section libraries. Section/Geometry optimization. Applies preset and/or custom load combinations. FEM driven design. Powerful Revit integration. Simple, easy to use, reasonably priced.
or structural engineers, the ability to design and analyze complex trusses with precision and efficiency is critical. Yet, many existing tools are limited by rigid frameworks and compatibility issues, restricting the potential for innovation. Paragon, the latest advancement in truss design software, is here to break those limitations and empower engineers with a flexible, powerful
Paragon’s core strength lies in its adaptability. Unlike traditional software, Paragon allows engineers to work with plates from multiple manufacturers on any truss design from any device with a modern web browser. This flexibility enables engineers to customize and optimize their designs without being constrained by the software. Whether you’re working on a small project or a largescale development, Paragon’s open platform provides the tools to get the job done. Integration is another key feature that sets
Paragon apart. Our software works with industry-standard tools like AutoCAD and Revit, allowing for the incorporation of truss analysis into your existing workflows. This compatibility ensures that you can maintain the continuity of your projects while enhancing them with Paragon’s advanced capabilities.
Collaboration is also at the heart of Paragon’s design. The software offers collaboration features that enable engineers, architects, and builders to work together seamlessly, no matter where they are. This capability not only streamlines communication but also reduces the risk of errors and improves overall project efficiency. With everyone on the same page, projects can move forward more smoothly and confidently.
But Paragon isn’t just about solving immediate challenges—it’s about empowering engineers to explore new possibilities. With its intuitive interface and cutting-edge technology, Paragon is designed to support the evolving needs of today’s structural engineers. Whether you’re optimizing or analyzing existing designs, testing for feasibility, or pushing the boundaries of what’s possible, Paragon gives you the tools to lead the way in truss design innovation.
In a rapidly changing industry, having the right tools can make all the difference. Paragon is more than just a software solution—it’s a partner in innovation, helping you stay ahead of the curve and achieve your design goals with greater efficiency and creativity.
Structural Engineering Software For Engineers, By Engineers
Powerful, Easy-To-Use Structural Engineering Software For Low-To-Mid Rise Structures
To gain perspective on how ENERCALC software can help you, consider your last flight. Before landing, a downtown cluster of skyscrapers, stadiums, and malls appeared. Surrounding them for many square miles: parking garages, manufacturing facilities, warehouses, retail, hotels, medical complexes, residential & commercial buildings.
These low-to-mid rise structures make up 90% of structural engineering work.
ENERCALC calculation software specializes in this work, offering consistent workflow, simple data entry, and fast recalculation.
Today’s building codes are complex, with many load combinations and specific design details. Result: Hand calculations are seldom productive. ENERCALC includes 41 classic structural calculation modules - including earth-retention structures & 3D FEM.
New in 2024 Load Linking
We launched Load Linking, starting with the Steel Beam module. Reactions from one Steel Beam calculation can be automatically referenced as applied loads in another Steel Beam calculation.
The ENERCALC project manager constantly monitors for changes. Visual alerts appear in the Project Manager, which make it simple to manage & monitor dependencies.
You can view the list of beams in a project file in their order of dependence, review the status of linked calculations, and update the necessary calculations with the click of a button.
We introduced full path analysis support for steel beam, steel column, and general footing. EFR now allows you to perform analysis and design tasks from beam to girder to column to footing.
We released ASCE 7-22 Loads & Forces modules. You can now add these modules to your project from the Add Modules menu - like other calculation modules. You can have as many Loads & Forces calculations as you need, name them as you wish, and move them where you want them.
Some states have adopted IBC 2021 with a modification to apply ASCE 7-22. To account for this mix-and-match of building codes and design standards, we’ve separated the ASCE 7 edition selection from the IBC edition selection. Printed reports clearly reference the building code and the design standard(s) selected.
ENERCALC is a small, close-knit team of structural engineers and software developers. Since 1982, we’ve focused on simplifying the work of structural and civil engineers. Our goal: Help you become more productive so you can get home for dinner on time.
Steel Tube Institute
Phone: 847-461-1701
Email: hssinfo@steeltubeinstitute.org
Web: steeltubeinstitute.org
Product: HSS Connex
Description: The Steel Tube Institute’s HSS Connex is a free web-based tool that offers designers a simple way to check HSS wall thicknesses to aid in efficient HSS connection design. These local limit state checks are helpful to ensure HSS wall thicknesses are adequate at connections.
Qnect
Phone: 413-387-4375
Email: pearl@qnect.com
Web: qnect.com
Product: QuickQnect
Description: QuickQnect software is used by EORs to automate the creation of early connected models. Key features include detecting and resolving issues early and rapidly responding to design changes. To learn more about latest capabilities you can watch this recent webinar on our YouTube channel: https://www.youtube.com/ watch?v=HSRkWzzEnKM
RedBuilt
Phone: 866-859-6757
Email: info@redbuilt.com
Web: https://www.redbuilt.com/
Product: RedSpec™
Description: A convenient, user-friendly design program that lets you quickly and efficiently create floor and roof design specifications using Red-I™ Joists, RedBuilt™ Open-Web Trusses, RedLam™ LVL, Glulam Beams, and dimensional lumber. RedSpec™ is provided free of charge to registered users. For support, contact us at RedSpec@RedBuilt.com
Trimble
Phone: 678-737-7379
Email: jodi.hendrixson@trimble.com
Web: www.tekla.com/us
Product: Tekla Structural Designer
Description: With Tekla Structural Designer, engineers have the power to analyze and design multi-material buildings efficiently and cost effectively. Fully automated and packed with unique features for optimized concrete and steel design, Tekla Structural Designer helps engineering businesses win more projects and maximize profits.
STRUCTURAL ENGINEERING Resource Guide
ITrimble
Phone: 678-737-7379
Email: jodi.hendrixson@trimble.com
Web: www.tekla.com/us
Product: Tekla Structures
Description: Create and transfer constructible models throughout the design lifestyle, from concept to completion. With Tekla Structures, accurate and information-rich models reduce RFIs, leverage models for drawing production, material take offs and collaboration with architects, consultants, fabricators and contractors.
Simpson Strong Tie
Phone: 800-999-5099
Web: https://www.strongtie.com/solutions/ software/lotspec
Product: LotSpec
Description: LotSpec is a proven option management solution that works with both Autodesk Revit® and AutoCAD® Architecture. It is an add-in that supports structural options using intuitive rules, smart tools, and flexible workflows. Simpson Strong-Tie is committed to helping customers succeed by providing quality products, software technology, and exceptional customer service
DEA StatiCa is the world-leading solution for the structural design of steel connections, concrete details and critical members. Our applications are desktop based and enable structural engineers in more the 110 countries worldwide to accurately design structural components.
Our flagship product is IDEA StatiCa Steel. It includes our Connection design app and Member, an app for buckling analysis of critical members and assemblies.
Connection design is getting more complex as projects get more complicated. Engineers are finding many situations where the current limit states equations are difficult to apply and thus need an additional tool such as IDEA StatiCa which allows you to easily model any connection geometry or loading and run a finite element analysis on it and compare the real behavior to the allowable material properties.
Member app allows you to run buckling analysis of members and assemblies. The difference is that it allows you to model the actual end
conditions, not just a pin or fixed support. The app uses the actual stiffness of the connections in the member buckling analysis, giving you realistic results and an understanding of the behavior of your structure.
IDEA StatiCa is an independent software developer. This means that we work with a variety of other structural software that you use every day. How many other structural solutions allow you to use the software that you want to use and share your global design information to design connections? Earlier this year, we released a free version of our Checkbot app to help facilitate the sharing of information between engineers and detailers and even connection designers for those that delegate connection design. This means that the EOR can import their analysis model into Checkbot, which will include the geometry of the structure and the load reactions on members. That file can then be used to design the connections. Once the connection design is completed, an IFC file can be exported and then used by the detailer to model the connections in their detailing model. Again, we work with a variety of detailing packages, so you can use the software that you want to use without have to markup PDFs or sketches to send back and forth among the project team. If you work on steel projects, you should look into IDEA StatiCa to help you provide safe and accurate designs in the least amount of time.
RISA has been delivering a suite of leading edge structural design and optimization software for over 30 years. Our products are used around the world for buildings, stadiums, bridges and everything in between. The seamless integration of RISAFloor, RISA-3D, and ADAPT-Builder creates a powerful structural design environment, ready to tackle your next design challenge.
RISA is the engineer’s companion at every stage of their journey. At every stage of their career—from entry-level to experienced professional—engineers need reliable tools and resources. RISA stands as a trusted companion on this journey, offering comprehensive support that spans the entire professional lifecycle. Central to this support is the RISA Learning Center, a resource designed to empower engineers at every step.
The RISA Learning Center
For new engineers, the path to becoming proficient can be daunting. They must quickly grasp complex concepts, navigate sophisticated software, and apply theoretical knowledge to real-world scenarios. The RISA Learning Center serves as an essential foundation, providing the resources needed to build skills and confidence. With its userfriendly interface, the Learning Center offers easy access to a wealth of educational materials, ensuring that even those new to the field can quickly get up to speed.
The importance of a strong start cannot be overstated. By leveraging the resources available through the RISA Learning Center, new engineers can develop a deep understanding of core principles and software tools, laying the groundwork for a successful career. The Center’s offerings include live sessions where engineers can interact with experts in real-time, as well as on-demand content that allows for flexible, self-paced learning. This combination of learning opportunities ensures that every engineer, regardless of their preferred style, has the chance to excel.
As engineers progress in their careers, continuous learning becomes critical. The structural engineering industry is marked by constant innovation, with new materials, design standards, and technologies emerging regularly. To stay competitive, engineers must keep their skills sharp and up to date. The RISA Learning Center is an invaluable resource for ongoing professional development, offering a comprehensive library of training materials that cover both fundamental and advanced topics.
The adaptability of the RISA Learning Center to industry changes is one of its key strengths. Engineers can access video tutorials, model
files, and detailed documentation that help them stay current with the latest trends and best practices. This continuous learning enhances their technical expertise and positions them to take on more complex and challenging projects, driving both personal and professional growth. The impact of RISA’s training programs on engineers’ careers is significant. Many professionals have shared success stories, crediting the Learning Center with helping them achieve new milestones in their work. One engineer noted, “This training is going to help us expand our ability to support larger building projects.” Another engineer, reflecting on their experience with the RISA training resources, remarked, “Combined with the PDF tutorial for RISAFloor that you sent me already, these resources create an excellent in-house introductory training for our new employees. You all do an excellent job of providing all types of training opportunities.” These testimonials underscore the importance of ongoing education in achieving long-term success in structural engineering.
For experienced engineers tackling advanced projects, the challenges are both technical and strategic. Complex designs require not only a deep understanding of engineering principles but also the ability to apply these principles in innovative ways. The RISA Learning Center offers advanced training that equips engineers with the expertise needed to navigate these complexities. Whether it’s mastering intricate software features or exploring new design methodologies, the Learning Center provides the expert guidance engineers need to excel in their most challenging projects.
The Learning Center’s focus on practical application is especially valuable for experienced engineers. The training materials go beyond theory, offering real-world examples and case studies that demonstrate how advanced techniques can be applied to solve complex problems. This hands-on approach ensures that engineers are not only learning new skills but also gaining the confidence to apply them in their daily work.
At every stage of an engineer’s career, RISA stands as a reliable partner, offering the tools and resources needed to succeed. From the first steps of learning and skill development to the challenges of advanced project execution, RISA’s support is comprehensive and unwavering. The RISA Learning Center, in particular, embodies this commitment by providing a platform for continuous growth and professional excellence with new online training resources added continuously.
Engineers are encouraged to take full advantage of RISA’s offerings, from exploring the extensive training opportunities in the Learning Center to engaging with RISA’s technical engineers for support or software tours.
949-951-5815 | info@risa.com | risa.com
ASDIP Structural Software
Phone: 407-284-9202
Email: support@asdipsoft.com
Web: https://www.asdipsoft.com
Product: ASDIP STEEL
Description: Software for the design of steel members and connections, such as composite/ non-composite beams, steel columns, base plates, anchoring to concrete, shear connections, moment connections, and web openings, per the latest design codes.
ENERCALC, LLC
Phone: 800-424-2252
Email: info@enercalc.com
Web: https://enercalc.com
Nucor Vulcraft Verco
Phone: 402-844-2400
Email: marketing@vulcraft.com
Web: https://vulcraft.com/
Product: Joist, Deck, and Bar Grating
Description: For over 70 years, Vulcraft and Verco have been leading manufacturers of high-quality steel joists, deck, and bar grating products in North America. We do more than supply steel - we partner with you to customize solutions that fit the demands of your project.
Product: ENERCALC SEL / ENERCALC 3D
Description: Save hours on every steel design with ENERCALC—now with FEM capabilities. Beams, columns, two dimensional frames, force distribution in bolt groups and more. The clear, simple user interface makes it fast & easy to setup, confirm & “what-if” your designs. Member optimization improves your efficiency and saves time!
Hohmann & Barnard
Phone: 800-645-0616
Web: www.h-b.com
RISA Technologies
Phone: 949-951-5815
Email: info@risa.com
Web: risa.com
Product:RISA-3D
Description: RISA-3D is the best choice for the design of all things steel. Whether designing custom hot rolled steel shapes or cold-formed steel wall panels, RISA-3D has you covered. With steel databases and design codes from all over the world, you’ll never have to look anywhere else.
Product: Rainscreen Support System
Description: After years of R&D, Hohmann & Barnard introduces an innovative stainless-steel clip and rail system for the rainscreen industry. This system meets critical U-Value requirements for thermal efficiency while employing non-combustible materials. For more information on how this new stainless-steel rainscreen system can benefit your projects, visit www.h-b.com.
STRUCTURAL ENGINEERING Resource Guide
Innovators of engineered steel roof & floor deck solutions
Serving the Western United States since the 1970s, ASC Steel Deck is a leading manufacturer of high-quality structural steel deck solutions for the building and construction industry. Based in Kalama, WA, ASC Steel Deck continues to advance the steel deck industry through innovation and an unmatched commitment to customer success.
ASC Steel Deck can supply products with a range of metal thicknesses, galvanized coating weights, paint finishes, and perforation options to meet all project requirements, including pre-primed to save installation labor costs. ASC Steel Deck’s product line covers the scope of shallow to deep rib configurations and standard to high-strength offerings. These products are featured in high-profile projects, including stadiums, warehouses, office buildings, and airports.
One of ASC Steel Deck’s most unique offerings is its propriety cellular deck option, such as the Smooth Series™ product line. Smooth Series features a clean beam-to-pan rivet-attached flat pan underside, eliminating unsightly burn marks created by traditional welded attachments. This creates an aesthetically pleasing, blemish-free appearance while
allowing for composite design. Smooth Series is ideal for exposed applications where the underside of the deck will be visible.
In addition to manufacturing steel roof and floor deck products, ASC Steel Deck seeks to improve the installation process to help keep costs low through innovations. Key innovations include the DeltaGrip® DG4™ Tool, a mechanical side-seam attachment system that reduces the installation costs of high-shear diaphragms by eliminating costly top seam welds. This revolutionary structural sidelap connection system for steel roof deck applications builds upon the original DeltaGrip System by introducing the added benefits of higher connection strength, faster cycle times, and increased punch durability.
With a focus on making project design easier for customers, ASC Steel Deck provides BIM tools, design software libraries, and product samples to support project teams. By continuously advancing steel deck technology, ASC Steel Deck enables architects, engineers, and contractors to maximize value and performance. Their full range of steel roof and floor deck products offers economical, high-performance options for any building project.
800-726-2727 | info@ascsd.com | www.ascsd.com
Vulcraft and Verco are leading manufacturers of high-quality steel products. With over seven decades in the industry, we offer a comprehensive range of steel joists, deck, and bar grating products. But we do more than supply products - we partner with you to customize solutions that fit the demands of your project. Our goal is to provide efficient and sustainable solutions that ensure the success of your project.
VULCRAFT
Over the past 70 years, Vulcraft has expanded to become one of the largest manufacturers of steel joists, deck, and bar grating in North America. As part of the Nucor family, we remain committed to leading the industry in steel solutions.
VERCO DECKING, INC.
Verco Decking, Inc., serves the Western U.S. with high-quality steel deck products and innovative construction solutions. Our California and Arizona facilities collaborate closely with Western Vulcraft divisions to ensure seamless project completion across the region.
Steel Joists: Strength Meets Flexibility
We offer a complete range of efficient steel joists that enable architects and engineers to create open, functional spaces in single- and multi-story structures without compromising safety or durability.
From composite floor systems to open web joists, our products are built to handle heavy loads and deliver exceptional performance while reducing construction time across projects.
Our steel roof and floor deck products are engineered to offer unmatched performance. Whether you need composite deck for multi-story buildings or roof deck for large commercial spaces, our deck products deliver durability, easy installation, and flexibility. We provide deck solutions that are designed for structural efficiency, allowing for greater spans and higher load capacities. These products are ideal for a variety of structures, from industrial plants to high-rise buildings, and include additional features such as acoustical options and excellent fire ratings for added safety and comfort.
Bar Grating: Lightweight and Adaptable
Our bar grating products provide strength and adaptability for industrial platforms, catwalks, benches, shelving, and more. They
allow for optimal water and airflow, making them ideal for facilities from industrial warehouses to airfields.
Available in Standard, Heavy Duty, and Stair Tread types, our bar grating can be custom fabricated with various bar sizes, surfaces, finishes, and fastener styles to meet specific project requirements.
We offer a robust suite of online design tools to streamline your design process. These tools enable engineers and architects to accurately model and analyze project components, reducing design time and ensuring accurate integration of our joists, decking, and bar grating products.
Flush Frame Connections: Seamless Integration, Reliable Performance
Our Flush Frame Connections are designed to integrate smoothly with our steel joists. They provide a reliable and efficient load path throughout your structure while mitigating floor vibration, reducing floor-to-floor heights, and simplifying MEP installation, making buildings safer and more comfortable.
Our connection systems are rigorously tested to meet high safety standards and ensure long-lasting performance, even in extreme conditions like seismic zones and hurricane-prone regions.
Sustainability: Build a Greener Future With Steel
The Vulcraft and Verco commitment to sustainability is rooted in Nucor’s circular steelmaking process, which minimizes waste and energy use. Steel can be endlessly recycled and reused without any loss in quality, offering a significant opportunity to reduce our environmental footprint.
By choosing to partner with us, you’re selecting a company that is committed to environmental responsibility. Our experts will help you meet your sustainability goals by working with you to create customized plans for the joists, deck, and bar grating in your project. Seismic and Wind Solutions: Built to Withstand Nature’s Forces
In an era of climate challenges, we provide structural solutions designed to withstand seismic activity and high winds. Our steel joists and decking are engineered to meet the rigorous demands of seismic and wind codes, ensuring stability in the most demanding conditions, protecting both investments and occupants.
The Punchlok® II System strengthens steel roof deck connections, improving shear diaphragm strength and load transfer to better resist seismic and wind forces. Its secure design ensures that structures remain stable and intact during extreme conditions. Powerful Partnerships. Powerful Results.
We are dedicated to being your trusted partner in construction, offering a wide range of steel solutions customized to meet the needs of your project. From steel joists, decking, and bar grating to cuttingedge online design tools and customized joist connection options, our comprehensive solutions are designed to help you address the challenges of modern construction while contributing to a greener future. Choose Vulcraft Verco Group for innovative solutions that empower you to build with confidence.
Vulcraft.com | Vercodeck.com
ASC Steel Deck
Phone: 800-726-2727
Email: info@ascsd.com
Web: www.ascsd.com
Product: Steel Deck
Description: ASC Steel Deck is a leading steel deck manufacturer serving the Western U.S., offering an extensive product range for complex structural demands. With innovative solutions like DeltaGrip® DG4™ and Smooth Series, we provide engineers and architects with the products, software, and resources to confidently design. Explore more at www.ascsd.com.
New Millennium
Phone: 260-321-8080
Web: www.newmill.com
Product: Flush-Frame Design Guide
Description: Be sure you specify the use of New Millennium flush-frame end connections for your upcoming projects. It’s way better for weigh less. That’s right, steel joists with flush-frame connections offer significant weight savings compared to wideflange beams while providing equivalent stiffness and vibration performance. For help with specification, download the new Flush-Frame Design Guide now.
Atlas Tube
Phone: 800-733-5683
Web: www.atlastube.com
Product: HSS
Description: Engineers relied on Atlas Tube to provide 100% domestic, world-class HSS for Las Vegas’ revolutionary MSG Sphere. Atlas invested in specialized tooling for a first-ever run custom size and supported fabrication and logistics. Partner with Atlas on your next project.
Chicago Clamp Company is a leading innovator in the construction industry, specializing in providing safe, efficient, and cost-effective solutions for roof opening and joist loading projects. With a rich history of engineering expertise, the company has developed a patented line of clamp systems that have transformed traditional construction methods.
For decades, the construction industry relied on time-consuming and potentially hazardous welding techniques for framing roof openings. Recognizing the need for an easier installation alternative for those painful and costly jobs that require welding onto steel joists, Chicago Clamp Company introduced its revolutionary clamp systems. These pre-engineered solutions eliminate the need for welding, significantly reducing labor costs and improving job site safety.
The company’s clamp systems are meticulously engineered and rigorously tested to ensure structural integrity and reliability. They offer versatile applications, accommodating a wide range of project requirements. Whether it’s supporting HVAC equipment, skylights, or other roof-mounted installations, Chicago Clamp Company has a solution. Beyond its innovative products, the company is committed to providing exceptional customer service and technical support. Their team of experts works closely with clients to understand their specific needs and recommend the optimal clamp system for each project. By combining cutting-edge technology with a deep understanding of the construction industry, Chicago Clamp Company continues to set new standards for efficiency, safety, and cost-effectiveness.
www.ChicagoClampCompany.com
New Millennium is your collaborative partner for the design, engineering, and supply of structural steel joist and roof and floor deck solutions for commercial steel construction. A wholly owned subsidiary of Steel Dynamics Inc. with seven manufacturing facilities across North America and local sales teams near you, we stand ready to meet your project needs.
We assist you right from the start in specifying and engineering the steel joists and steel deck best suited for your design and application. We engineer and manufacture solutions that control costs, enhance performance and ensure project success.
Steel joists and joist girders are key components of the most costeffective, performance-optimized structural steel solutions. All our steel joists and joist girders are engineered and manufactured in accordance with the specifications of the Steel Joist Institute.
• Standard steel joists and joist girders: Primary and secondary framing members, steel joists and joist girders support concentrated loads for roof or floor systems.
• Special profile joists: Profiles include gable, bowstring, arched, scissor and double-pitched joists. Over 40,000 special profile steel joist design possibilities.
• Composite joists: Enable the longest open floor spans possible. Flush-frame connections are a versatile and efficient method for connecting open-web steel joists to wide flange girders.
• WN-Series™ wood nailer joists: For stronger, moreefficient and better-performing wood-on-steel roof systems, our unique glued finger joint splices provide structural continuity the full length of the joist.
• Flush Frame Connections: Steel joists with flush-frame end connections offer significant weight savings compared to wide-flange beams while providing equivalent stiffness and vibration performance.
New Millennium manufactures a range of steel roof and floor deck profiles to address your structural and architectural needs. Our new IMPRESSIONS™ steel deck combines wood’s natural beauty with the strength of steel. A structural roof deck using our Versa-Dek® profile, IMPRESSIONS features digitally printed wood-grain finishes so realistic you won’t believe it’s steel. Steel roof can be installed with exposed structural frames to create striking, spacious interiors and dramatic, high-performing exteriors. Optional acoustical treatments absorb sound energy. Applications readily integrate lighting, electrical, plumbing and
fire suppression systems. For multi-story construction, longspan composite floor deck addresses multiple factors, including total floor depth; loads; and fire, vibration and acoustic ratings. Composite floor systems optimize the cost and performance of your mid-rise or high-rise project and can weigh up to 40% less than comparably utilized cast-in-place concrete floors. Long-span deck can be specified to minimize floor depth, optimize floor-to-floor height, reduce project costs and accelerate construction timelines. Composite deck used in combination with composite joists can achieve clear spans up to 60 feet uninterrupted by support columns.
Together with you, our team will help you find the ideal steel deck and deck finish for your project.
For new construction and rehabilitation of concrete or steel bridge structures, we offer two steel stay-in-place bridge deck forming systems.
• Bridge-Dek®: High-strength galvanized steel with factoryclosed ends that speed installation, creates a safe working platform, reduces costs 20% to 25% compared to wood forms, and integrates with steel or concrete girders.
• Rhino-Dek®: Polymer laminated for corrosive environments—available on one or both sides of the deck pan—protects exposed steel from salt corrosion. Provides a service life of 124 years.
To streamline the specification of steel joists and deck, we offer a range of informational and educational resources.
• Load tables: Our downloadable load tables catalogs for steel joists and steel deck enable you to work quicker, not harder.
• Online design tools: Interactive steel joist and steel deck design tools transform your design process, helping increase project performance and reduce project costs.
• Credit-hour courses: Our continuing education courses are offered in three calendar-friendly formats: live remote, on-site and on-demand. Many courses award AIA and PDH credit. Courses can be customized or tailored to your interests and needs. You’ll learn from experienced steel specialists and advance your knowledge of structural steel.
Solve engineering challenges. Realize project goals. Control costs. Your success begins with the selection of the right steel joists and deck—and the right supplier—for your application. At New Millennium, we will help you determine the optimal structural steel solution for your application. Together, let’s build it better. newmill.com
New Millennium
Phone: 260-321-8080
Email: sales@newmill.com
Web: https://www.newmill.com/
Product: Steel Joists and Deck
Description: New Millennium joist and deck are made with steel, the most recycled material in the world. See our Sustainability page for more information on our commitment to sustainability including EPD’s, recycled content and regional materials. https://www. newmill.com/resources/leed.html
Nucor Vulcraft Verco
Phone: 402-844-2400
Email: marketing@vulcraft.com
Web: https://vulcraft.com/
Product: Steel Joists, Deck, and Bar Grating
Description: Vulcraft and Verco are is committed to sustainable design, offering steel solutions that prioritize environmental responsibility. Through circular steel-making processes and strategic partnerships, we work closely with our customers to ensure that their projects efficiently meet today’s rigorous environmental standards while contributing to a more sustainable future.
CTS Cement Manufacturing Corp.
Phone: 800-929-3030
Email: sfoster@ctscement.com
Web: www.ctscement.com/komponent
Product: Komponent
Description: Komponent® is an expansive cement additive blended with portland cements to create shrinkage-compensating concrete and grouts. It is engineered to prevent negative volume change, eliminate drying shrinkage cracking, and ensure dimensional stability and improved structural performance. Its ultra-low carbon intensity ensures optimized sustainable design and performance with improved constructability.
CTS Cement Manufacturing Corporation
Phone: 800-929-3030
Email: jong@ctscement.com
Web: www.ctscement.com/about/sustainable/ sustainability
Product: Rapid Set® Cement
Description: Rapid Set® Cement produces about 1/3 less carbon dioxide (CO2) than portland cement during manufacturing. Rapid Set’s reduced carbon footprint is due to less limestone, lower processing temperature, and greater durability. Ask us for Rapid Set’s certified Type III (product specific) environmental product declaration (EPD).
STRUCTURAL ENGINEERING Resource Guide
The Green Economy Is Built on Steel
As the top provider of steel joists, deck, and bar grating in North America, Vulcraft and Verco are dedicated to leading the way in sustainable construction solutions. From the materials we use to the processes we follow, we’re committed to building a greener future. We design and manufacture our steel joists, deck, and bar grating to maximize performance while minimizing environmental impact. This results in structures that are not only strong and resilient but also contribute to a greener planet.
Our sustainability efforts extend beyond steel products to our operations. Our commitment is rooted in Nucor’s circular steelmaking process, which minimizes waste and energy use. Steel can be endlessly recycled and reused without any loss in quality, offering a significant opportunity to reduce our environmental footprint.
For over 50 years, Nucor has been an industry leader in the use of electric arc furnace (EAF) steelmaking, which recycles end-of-life scrap into new steel. Nucor’s EAF steel recycling process produces only one-third of the greenhouse gas emissions compared to traditional extractive blast-furnace steelmaking methods.
As the industry’s key player in decarbonization, we are continually dedicated to reducing our environmental impact. We have a plan to lower our emissions by 2030 and are committed to achieving carbon neutrality by 2050.
Collaborating with our clients to create sustainable solutions is central to ensuring that decarbonization goals are met. We have a team of dedicated sustainability experts who will work closely with you to create custom solutions for your project’s steel joists, deck, or bar grating.
Based on your company’s goals, our team can provide detailed information on the embodied carbon content for your project proposals. Our use of Environmental Product Declarations (EPDs) ensures transparency so that you can make informed decisions at every stage of your project. Choosing Vulcraft and Verco products means more than just selecting custom-engineered products. It means joining us as we constantly research, innovate, and develop new ways to reduce environmental impact without compromising on quality or performance.
Reach out to one of our experts and find out how we can help.
Vulcraft.com | Vercodeck.com
Jumbo HSS and Shuriken™ help engineers and architects achieve their vision.
As the construction and development industry evolves to meet the demands of the future, so too must the structural engineers and architects responsible for designing the buildings of tomorrow. Projects continue to increase in size and scale, which means building materials play a far more significant role in ensuring their successful completion. While challenges are an inherent part of the job — budget constraints, labor shortages, fluctuating materials costs, supply chain issues and environmental regulations, to name a few — outside-of-the-box solutions can help architects, engineers, contractors and fabricators overcome them and deliver on the project’s vision.
Supporting the design possibilities. Atlas Tube is a domestic manufacturer helping structural engineers meet the challenges of today by innovating new solutions, expanding existing product offerings and delivering on-time for the most demanding project schedules and budgets.
Nothing exemplifies this more than two Atlas Tube products: Jumbo HSS and Shuriken. Each of these products was specifically designed to help engineers, fabricators and erectors overcome common challenges without sacrificing their vision. North America’s largest HSS and Shuriken bolted connections allow engineers to design on a larger scale while at the same time addressing labor and material cost challenges. Build bigger, wider, taller. As the largest domestically manufactured HSS in North America, Jumbo HSS offer the highest strength-to-weight ratio of any structural steel available. That means less steel and less cost for today’s largest applications, like factories, warehouses, hangars, data centers, bridges, high-rises and more. Their superior efficiency and aesthetically appealing design compared to wide-flange sections allow engineers to support the demand for more open, better-utilized spaces. Efficiency is key. The high strength-toweight ratio and size of Jumbo HSS (up to 22” square with 1” wall thickness) creates the potential to use less steel in a project, resulting in increased efficiency — critically important given the labor shortage and fluctuating material costs. And for fabricators, the opportunity to use Jumbo HSS instead of built-up boxes can save valuable shop hours
when labor is tight. Jumbo HSS can also have 50% less surface area than wide-flange sections, further reducing prep and finishing time and costs. Big in size. Small on environmental impact. In addition to their unique size and strength, another appeal of Jumbo HSS is their low environmental footprint. Steel is an endlessly recyclable material, so steel HSS are inherently more environmentally-friendly. Atlas Tube also produces their steel HSS using sustainable manufacturing practices, helping to lower a project’s embodied carbon. Roughly 70% of HSS in North America are produced using electric arc furnace (EAF) steel coils. Atlas Tube exclusively purchases steel from North America, which has among the lowest carbon emissions of the leading steel manufacturing regions. In fact, steel manufacturing in the U.S. produces 60% fewer greenhouse gas emissions than in China, a top steel exporter to the U.S. Atlas Tube’s manufacturing facilities are also continually optimizing energy usage and minimizing wastewater, while ensuring that all steel scrap is recycled.
Connections made easy. Erecting HSS is now even easier thanks to Shuriken, an innovative solution that allows installation of A325 and A490 bolts from one side, reducing or eliminating the need for fieldwelding. Shuriken is a built-in wrench that allows steel fabricators and erectors to quickly and easily field-bolt HSS columns, beams and trusses; connections in tight spaces; and even SpeedCore. By eliminating field-welds, Shuriken speeds erection, reduces cost, and simplifies inspection, making HSS even more costeffective and efficient.
Dream big, design big with Jumbo HSS and Shuriken. Stronger, more innovative, more sustainable building materials like Jumbo HSS and Shuriken make it possible to continue designing and building for the future while facing the challenges of today. To learn more about either product, visit atlastube.com.
A shared vision. Atlas Tube is part of Zekelman Industries — a family of domestic manufacturers striving to help builders meet today’s challenges by delivering new, better ways to build. Their shared vision of operational excellence and best-in-class customer service make them the partners of choice for those with big aspirations. To learn more about Zekelman Industries, visit zekelman.com. atlastube.com
WoodWorks
Email: info@woodworks.org
Web: www.woodworks.org
Product: Free CAD/Revit
Description: Design mass timber, light-frame or hybrid projects more easily. Free details in PDF, DWG, or Revit formats. Mass timber – Connection and assembly details. Filters for class, load, cost, constructability, and fire rating. Light-frame – Structural and architectural details. Fire and acoustic-rated assemblies, floor-to-wall intersections, and more.
ICC-ES
Phone: 800-423-6587
Email: es@icc-es.org
Web: https://icc-es.org
Product: Total Conformity Assessment Solutions
Description: With testing, inspection and certification all under one roof, ICC-ES offers total conformity assessment solutions for the global building market. Our knowledgeable team of experts will guide you through the process. Ask about our Transfer and Save program for new clients!
RISA Technologies
Phone: 949-951-5815
Email: info@risa.com
Web: risa.com
Product:RISAFloor
Description: RISAFloor and RISA-3D form the premiere software package for wood design. Create 3D models of your entire structure and get complete design of wood walls, flexible wood diaphragms, dimensional lumber, glulams, parallams, LVL’s and joists. Custom databases for species, design of strap and hold-downs as well as panel nailing offer total flexibility.
Phone: 800-250-3196
Web: https://ncees.org
Product: NCEES Records
Description: The NCEES Records program is designed for currently licensed engineers and surveyors who are looking for an easier and faster way to complete the licensure process in multiple jurisdictions. An established NCEES Record will include most—if not all—of the materials you need to apply for comity licensure in additional states and territories.
NASDIP Structural Software
Phone: 407-284-9202
Email: support@asdipsoft.com
Web: https://www.asdipsoft.com
Product: ASDIP WOOD
Description: Software for the design of wood members such as biaxial columns, continuous beams, shear walls and out-of-plane bearing walls based on the latest NDS provisions for ASD and LRFD design methods. Easily select Sawn lumber, Glued Laminated, or Composite Lumber sections.
CEES is a national non-profit organization with a mission to advance licensure for engineers and surveyors in order to safeguard the health, safety, and welfare of the public. This mission is supported through its member boards, board of directors, staff, board administrators, and volunteers by:
• Providing outstanding nationally normed examinations for engineers and surveyors
• Providing uniform model laws and model rules for adoption by the member boards
• Promoting professional ethics among all engineers and surveyors
• Coordinating with domestic and international organizations to advance licensure of all engineers and surveyors
Through education, experience, and exams, professional engineering and surveying licensure establishes an important verification of expertise that is critical in safeguarding the public. NCEES offers more than exams for engineers and surveyors. Through the Records Program, Credentials Evaluations, and CPC Tracking, NCEES is here to help engineers and surveyors
become licensed in multiple states, track continuing professional competency requirements, and to help international professionals become licensed in the United States. Visit www.ncees.org to learn more.
800-250-3196 | www.ncees.org
Design wood structures effectively, economically and with ease!
Established decades ago, WoodWorks® Sizer, Shearwalls, and Connections programs are positioned as high-value, low-cost software with several thousand loyal customers in the U.S. and Canada. WoodWorks® is widely recognized as highly reputable since development of both the Canadian and U.S. versions are led by the Canadian Wood Council (CWC) and endorsed by American Wood Council (AWC) (U.S. version). For the U.S. version, we work closely with AWC to ensure consistency in technical interpretations of design provisions in the IBC, NDS, SDPWS, and ASCE 7. Both AWC and CWC participate in codes and standards development, and therefore have the necessary background to ensure the software operates as the codes and standards intend. WoodWorks® software programs provide designers with a quick and accurate way to design and optimize wood structural members, light-frame shear walls, and connections.
WoodWorks® Sizer
The WoodWorks® Sizer is our most used software program. It enables designers to design beams, joists, columns, wall studs and CLT panels used in light-frame as well as heavy and mass-timber construction. Sawn lumber, glulam, structural composite lumber, I-joists and CLT panels are available. The program analyzes all load combinations from ASD or LRFD as per IBC and ASCE 7 and designs for structural and fire performance following AWC’s National Design Specification (NDS).
The WoodWorks® Shearwalls is our most time saving program as it allows engineers to model the lateral force-resisting system of a light-frame wood building up to 7 stories either using an AutoCAD exported file as a template or by drawing from scratch. The software will automatically generate wind and seismic forces based on ASCE 7, distribute the forces to each level, within each level to each shearline based on flexible and rigid diaphragm analyses, and within each shearline to
each shear wall segment based on wall stiffness or capacity. It models proprietary shear walls and designs full-height, forcetransfer and perforated shear walls, and optimizes for sheathing and nailing by iterating and automatically selecting the most economical sheathing and nailing patterns. The program also checks the inter-storey drift limits and implements provisions for structural irregularities from ASCE 7.
WoodWorks® Connections
The WoodWorks® Connections program enables designers to design connections consisting of bolts, lag screws, nails, wood screws, shear plates, rivets, and heavy steel hangers. The program includes different types of connections such as wood-to-wood, steel-to-wood, and wood-to-concrete. The results are displayed as fully dimensioned CAD quality drawings.
Visit our website at woodworks-software.com to download a free trial, watch our training videos, or to access our stepby-step tutorials.
| sales@woodworks-software.com | www.woodworks-software.com
Phone: 276-645-8000
Email: info@strongwell.com
Web: https://www.strongwell.com/
Product: EXTREN
Description: Fiberglass structural shapes and plate that replace traditional materials for applications needing corrosion resistance, durability, light weight, ease to field fabricate, low thermal and electrical conductivity, and EMI/RFI transparency. The EXTREN® line consists of more than 100 different shapes, each with a very specific, proprietary composite design.
OneDek
Phone: 888-970-2947
Email: sales@awipanels.com
Web: www.powerofonedek.com
Product: Insulated Roof Deck
Description: As a transformative, high-performance alternative to traditional low-slope roofing assemblies, OneDek features include:
• Tapered insulation, parapet wall scuppers and internal drains
• Fewer total roof assembly components, which result in fewer labor hours
• Full assembly, industry-exclusive weathertight warranty
The Masonry Society
Phone: 303-939-9700
Email: info@masonrysociety.org
Web: www.masonrysociety.org
Product: Masonry Standards Online
Description: Masonry Standards Online provides easy access to TMS standards from anywhere with enhanced commentary, additional features, and multi-user access. Features include copying, printing, highlighting, annotating, internal linking, links to references and other TMS resources, helpful notations and definition tool tips, and advanced search options.
RISA Technologies
Phone: 949-951-5815
Email: info@risa.com
Web: risa.com
Product: RISA-3D
Description: RISA-3D designs and optimizes steel, concrete, masonry, wood, cold-formed steel and aluminum with a fast, intuitive interface. State of the art solvers, customizable reporting options and robust integration with other products such as RISAFloor, RISAFoundation and Revit make RISA-3D the premier choice for general purpose structural analysis and design.
listed? 2025 Resource Guide forms (monthly and annual) are now available on our website.
STRUCTURAL ENGINEERING Resource Guide
Strongwell is the recognized world leader in the manufacture of fiber reinforced polymer (FRP) composites utilizing the pultrusion process – a continuous production process which creates structural composite parts with a constant cross-section.
Since 1956, Strongwell has developed hundreds of FRP structural shapes, plate, gratings, planking, railing, fencing, structural building panels, and much more, made in one of Strongwell’s three U.S. ISO 9001 certified facilities.
FRP has a higher strength-to-weight ratio than most other materials, weighing approximately 75% less than steel and 30% less than aluminum. Unlike metals, FRP will not permanently deform under load.
FRP is corrosion resistant – it won’t rust or rot, virtually eliminating routine maintenance. This significantly reduces life cycle costs. The polymer resin infused in the parts can also be optimized to accommodate extreme environments.
FRP is low in thermal and electrical conductivity, making it a safer choice for applications near power transmission or distribution, or for areas where heat transfer is a concern, such as thermal breaks. Strongwell’s FRP is transparent to radio waves, microwaves, and other electromagnetic frequencies, making it a frequent choice for
cellular applications such as rooftop screening, enclosures, and support structures.
FRP products offer excellent fire properties. Most Strongwell FRP is slow to ignite and selfextinguishing once the flame source is removed.
FRP products will not leach any undesirable contaminants into water, soil, or air, making it suitable for water, ground, and animal contact without any special treatments or coatings.
EXTREN® structural shapes and plate replace steel, aluminum, and wood in a variety of structural applications, including stair structures, platforms, rooftop screening, and pedestrian bridges.
COMPOSOLITE® structural building panels are suitable for load bearing structural applications including buildings, bridge enclosures, pedestrian bridge decks, tank covers, platforms, walkways, enclosures, and secondary containments.
STRONGIRT® continuous insulation (CI) cladding attachment support system is an all-FRP rainscreen support solution designed for superior flame performance, screw pull out strength, thermal efficiency, and the ability to work with any insulation ranging from 1.5” to 8” thick.
276-645-8000 | info@strongwell.com | www.strongwell.com