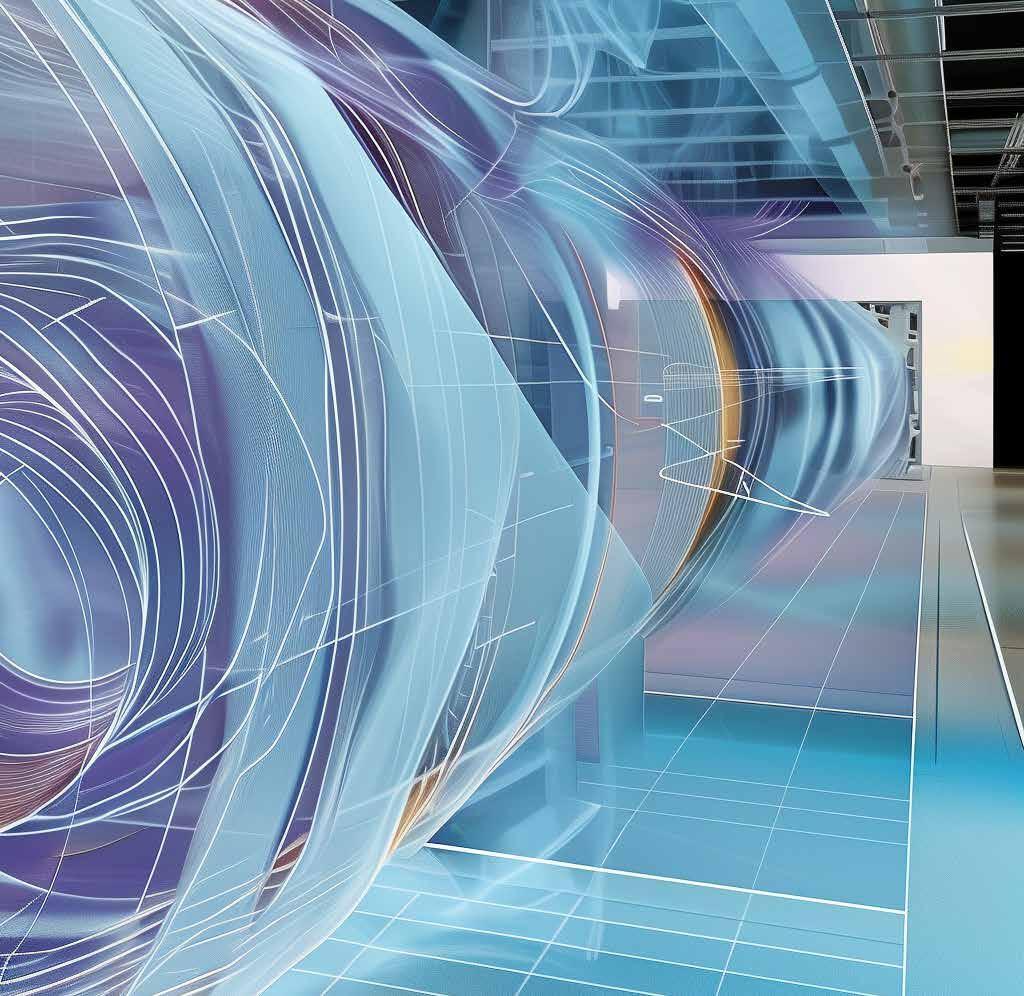
CEED
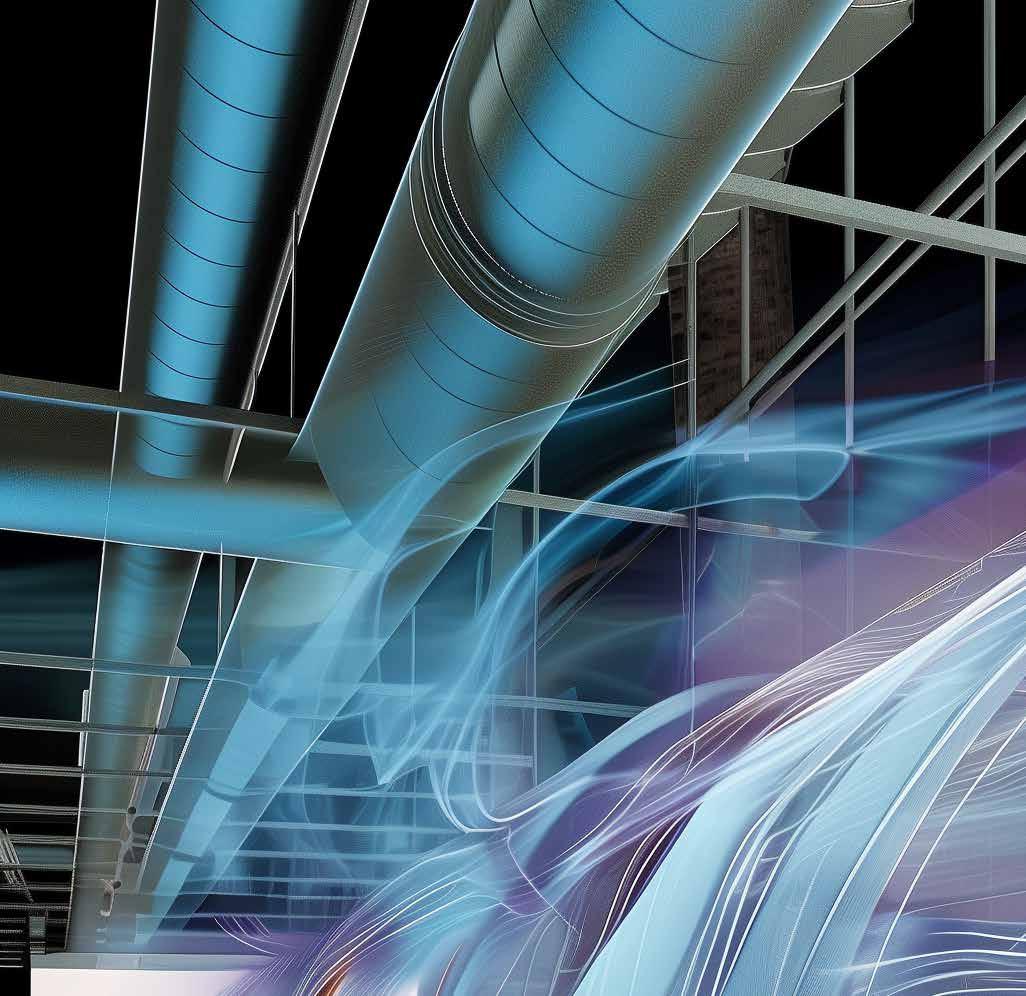
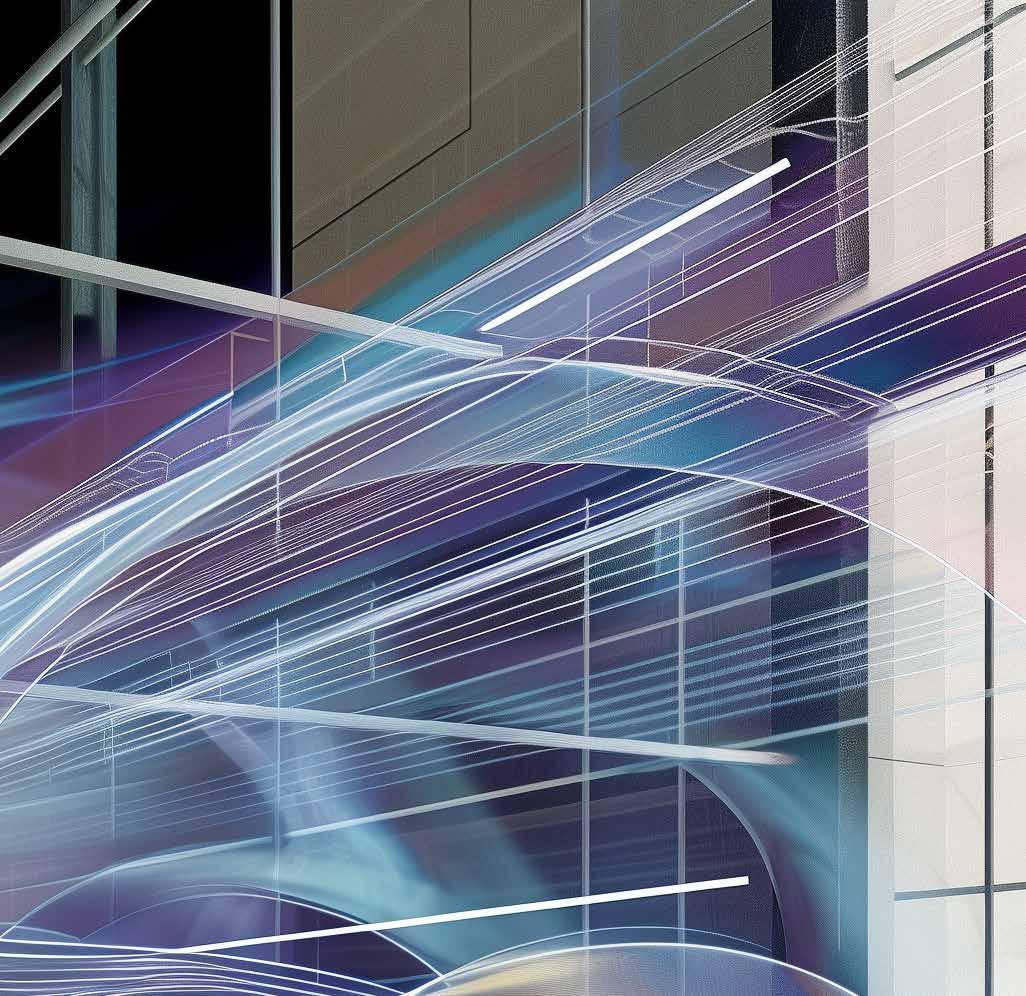
CLIMATE, ENERGY AND ENVIRONMENTAL DESIGN
One of TSPC's most innovative and forward-thinking design and design support groups is the CEED (Comfort, Energy and Environmental Design) division.
It is Hungary's first and market-leading team to introduce the most advanced technologies of complex climate and energy modelling into the architecture, building service and urban design portfolio in order to take the energy efficiency of our buildings to the next level: achieving an indeed zero or even positive energy balance in our designed buildings is no longer an unachievable goal. The team is made up of architects, building services and mechanical engineers, who think and work with both archi-tectural and engineering mind, and thus act as a kind of interdisciplinary interface between the different sectoral planning fields during the design process.
Climate, Energy and Environmental Design (CEED) is an approach to the design of the built environment that creates environmentally sustainable, energy efficient and climate conscious buildings that are comfortable – at the highest possible level.
> Climate and energy optimized planning and design support
> Comfort, energy and aerodynamic simulations
> Environment friendly design and consulting
> Green certification based design and survey
The ultimate goal of the TSPC CEED team is to create high-performance buildings that leave a minimal environmental footprint while providing healthy, comfortable and productive spaces for occupants. This requires integrated design involving architects, engineers, ecologists and other professionals from the earliest stages of the project.
CEED is primarily a design and planning optimization method, which integrates new engineering and scientific fields into the conventional design process of the built environment: building climatology, comfort theory, building aerodynamics, building envelope technologies, building bionics, lighting technology, thermal, lighting and aerodynamic simulations, building energy, sustainable urban planning and climate concept development.
The design method applies climate zone based spatial organization, climate-adaptive building envelope (facade and roof design), aerodynamic shape and structure design, thermal and environmental structure optimization, mechanical and energy system analysis, simulation based ÉKM energy calculation acc. to national-international regulation, detailed services system modelling, and quantifies their effects and results in terms of thermal comfort, visual comfort, annual operational performance and energy demand.
It also models the environmental impact (LCA, LCCA) in form of different ecological footprints. In terms of sustainability and environ-mental adaptation, the high-performance buildings of CEED projects achieve levels of efficiency in terms of operational energy consumption, carbon footprint, comfort and healthy indoor environment, and investment costs that have not been achieved so far.
GEOMETRY
STRUCTURE
AESTHETICS
BUILDING CLIMATE
BUILDING TECHNOLOGY
BUILDING ENERGY
ENVIRONMENTAL CONSCIOUSNESS
BUILDING CLIMATE
> Thermal comfort
> Visual comfort
> Acoustical comfort
> Olfactory comfort
> Haptic comfort
> Local climate
> Local geography
BUILDING TECHNOLOGY
> Building bionics
> Low-tech vernacular tech.
> High-tech
> Building envelope
> lnnovative design methods
BUILDING ENERGY
> Time
> Thermal energies
> Aerodynamics, ventilation tech.
> Thermal masses
> Losses – gains
> Energy performance
> Hydraulics
> Facility management
> Efficiency, pay-back
ENVIRONMENTAL CONSCIOUSNESS
> LCA, LCCA
> Natural materials, structures
> Embodied C0 2
> Ecological footprint
CLIMATE ADAPTION AND SUSTAINABLE ENERGY USE
> A mechanized ship is able to float acc. to the journey at any time and in any weather conditions, at the cost of high fossil fuel consumption and pollution. In contrast, a sailing vessel actively adapts to the prevailing weather and environmental conditions and draws on local energy resources. This also provides a special look: Form Follows Climate, Energy and Environment. The same applies to CEED buildings.
> Designing buildings to minimize energy consumption – heating, cooling and lighting – through thermal and fluid dynamics related space arrangement and shape design, efficient building envelope structures, optimal glazing ratio and orientation of glazed surfaces, passive solar principles and natural ventilation-cooling and lighting.
> Integration of renewable energy sources to reduce dependence on fossil fuels.
> Potable and rainwater-greywater management
> Minimizing the environmental footprint of built structures over their entire life cycle, ensuring minimum embodied energy and carbon emissions.
> Minimize carbon emissions from building operation.
> Reasonable conservation and restoration of existing built fabric and infrastructure.
> Potable water and rainwater-greywater management
> Optimize visual comfort, natural daylighting and illumination through shaping, orientation and transparent building envelope structures.
> Ensuring thermal comfort via healthy and energy efficient structures, materials and services systems.
> Optimizing indoor air quality through combined aerodynamic natural and artificial ventilation.
A primary aspect of a planning project is the analysis of local climate conditions, a so-called climate analysis. The climate analysis is the basis for achieving sustainability objectives by carrying out a climate-adaptive planning process. This requires the collection of local climate measurement data (where available) and the generation of data from other high-resolution meteorological databases, such as: cloud cover, diffuse and direct solar radiation, outdoor air temperature and relative humidity, and annual wind speed and wind direction, respectively.
Rooms with similar internal comfort requirements are not only designed in a functional sense, but are arranged into so-called climate zone groups, taking into account the needs for efficient daylighting and natural ventilation as well as the timely distribution of the local solar path. The climate zone groupings are organized horizontally, vertically as well as per level to form a unified building in an orientation-dependent manner, considering heat losses and gains. The result is a building shape that minimizes heat loss and protects itself from overheating in summer by self-shading or by shading from neighbor buildings (loss-minimizing strategy). Thermal and photo-metric-lighting buffer zones, spaces behind glazed surfaces exploit solar heat gains (gain-maximizing strategy).
The building envelope is the interface structure between the effects of external climate influences and the effects of functional and comfort requirements within the building. This building-energy interface is intended to manage the energy balance, the ’energy houshold’ between the internal and the external world. As the external weather conditions change continuously over time, the building envelope should be orientation dependently designed to take account of the different, daylighting conditions, by considering the glazing ratio, the type of glazing, the shading solutions and roof structure.
In addition to comfort and energy, another important pillar of sustainable construction is the use of robust, long-lasting building structures made of renewable or recycled building materials. The grey energy content and CO 2 -equivalent (emissions) of these materials are significantly lower compared to conventional structures (e.g. solid reinforced concrete, brick masonry, or steel frame and aluminum structures). In a new building (e.g. German KfW55 energy rating), the grey energy used for production, transport, conversion, installation, etc. in a building accounts for about 50% of the building's operational energy consumption over its entire life cycle. Depending on the application, the manufacturing energy demand of the regenerative or recycled structures is 20-90% lower than that of conventional structures. In all the regenerative building materials is common that they are typically natural, healthy materials (i.e. without harmful emissions).
The first step in designing a sustainable building is to maximize energy savings by all possible and reasonable passive design solutions. Then, the building services system should cover the remaining comfort and energy needs, using the most efficient components available on the market, preferably with renewable energy.
The heat dissipation system should be selected and designed to suit the type of use (building), the applied building structures and materials, and the specific user needs and comfort or energy requirements. The heat generation system shall consist of a heating and cooling center of the building and shall use renewable energy sources (geothermal energy, solar thermal energy production, solar electricity generation, waste heat recovery from other processes and combined heat and power generation (CHP)) where possible. Considering the local geographical and climate conditions, heating and cooling energy production should be as regenerative as possible (geothermal, solar, biomass, district heating), as well as made of renewable electricity production, renewable raw materials or waste heat from available waste or other processes and technologies.
Air handling unit system completes the heating and cooling mechanical system and complement natural ventilation to ensure the required air quality. Mechanical ventilation is indispensable in certain climate conditions due to the high energy efficiency of the heat recovery unit (HRU) or due to extreme external conditions. In addition, the ventilation system ensures high thermal comfort.
The BMS completes the assembly and matching of building structures and mechanical systems, ensures optimal, fine-tuned operation of the building all year round, and ensures robust-ness against user behavior, interference and weather. Only an intelligent and as simple as possible automated regulation and control system can, for instance, efficiently operate mechanical ventilation and passive window ventilation motorization.
The primary task of climate concepts is to provide a framework for the different climate-energy design contents and to show the complex relationship between these aspects. As a result, the room organization, building body shaping, building envelope, building components and materials are defined and coordinated with each other, and different variants and combinations are tested. During the design of the building envelope, particular attention is paid to the climate adaptability of the facade and roof structure, so that the envelope can 'react' to the constantly changing weather conditions by intelligent design. A further design element is the strategic consideration of thermal mass of the building structures. Natural and mechanical ventilation concepts, as well as passive (use of daylight) and active (artificial) lighting concepts, complete the seasonal building management and the overall climate concept.
CLIMATE ZONES
THERMAL MASS
NATURAL VENTILATION IN TRANSITION SEASONS – OVERALL BUILDING SYSTEM
1ST FLOOR: MECHANICAL VENTILATION – HEATING OPERATION MODE
2ND FLOOR: ACTIVE AND PASSIVE FLOOR COOLING SYSTEM
The solutions, concept versions, building structures, etc. developed in the design phase can only be used with sufficient certainty in the construction stage if they are quantified, compared to each other (which version is better?) and verified (validated) from a building physics point of view, i.e. in terms of building climate, comfort and energy. For this purpose, thermal and photometric (daylighting) building simulations should be used, in particular for the timedependent calculation and evaluation of thermal and visual (daylight quality) comfort, as well as for the modelling of energy demand (heat loss and heat load caused by architecture) and energy consumption (of mechanical systems). Annual distributions of comfort and energy parameters, as well as annual balances of energy parameters, provide indispensable feedback on the efficiency and suitability of concepts and solutions in the design process.
Fluid flow or aerodynamic building simulations provide the highest resolution and accuracy for indoor passive ventilation and cooling. The design of the building shape, and location and size of the intake and exhaust vents, has significant influence on the functionality and efficiency of natural ventilation and thus on indoor air quality and thermal comfort, as well as on the energy use of active cooling. These aerodynamic performances can be modelled and evaluated using computational fluid dynamics (CFD) simulations. Aerodynamic models are particularly important because the flow pattern of natural ventilation0 cannot be calculated using conventional engineering design tools, but nevertheless passive ventilation is responsible for a significant proportion of the operational energy use.
The environmental impact is covered by Life Cycle Assessment (LCA) and Life Cycle Cost Analysis (LCCA), which cover the entire life cycle from resource extraction to reuse or recycling/ recovery. We bear in mind the operational energy, the environmental impact of building services systems and building structures, the recycled content in building structures, as well as potable water management, freshwater consumption. Global warming potential (GWP), ozone depletion potential (ODP), photochemical ozone creation potential (POCP), acidification potential (AP) and eutrophication potential (EP) are quantified.
Taking the whole life cycle into account, each CEED proposal that generates additional cost is subjected to a payback calculation and the cost-effectiveness or cost-benefit ratio, the expected payback period is determined. This is based on the results of complex building physics simulations. Additionally, it is also possible to maximize and optimize the different comfort and energy performances of the building, strictly within a given construction or operation manage-ment budget.
CEED innovations can revolutionize the design of the built environment. These innovations go far beyond improving energy efficiency, as they offer a range of benefits that can transform the way buildings are designed, built and operated.
From a sustainable comfort, energy and environmental efficiency perspective, we can create buildings that have not been possible before. By optimizing passive and active strategies, these innovations can significantly reduce energy consumption, operating costs and environmental impacts, while creating healthier and more comfortable indoor spaces.
Creating an optimal indoor environment that is not only energy-efficient but also comfortable and healthy is a top priority. This is achieved through the exploitation of passive design technologies, thermal and aerodynamic design of form and structure, as well as through the design and operation of space ventilation, daylighting and shading. On the other hand, the choice of an active system that is optimally adapted to the designed building ensures high thermal comfort sensation, air quality and the health and wellbeing of occupants with simultaneously reduced energy requirements: improved mechanical ventilation, air filtering and the use of non-toxic materials, healthy heat dissipation systems and their operation, control and optimization.
Providing adequate natural lighting is an integral part of CEED innovations. By maximizing the intensity of natural illumination under overcast sky conditions and by maintaining the necessary light conditions under clear skies through climate-adaptive shading and glare control, these innovations enhance advanced daylight autonomy and reduce the need for artificial lighting, while increasing thermal comfort.
A healthier indoor environment is achieved through improved ventilation, air filtering, healthy heat transfer and natural lighting conditions, and the use of nontoxic, mainly natural materials.
CEED innovations take a holistic approach to protect the environment, considering the whole life cycle of the building. This includes:
> CEED design can deliver significant operational energy and cost savings of >100% in new buildings and up to ≤100% in refurbishment projects. Energy independence and economic stability are no longer unattainable goals. Through passive, architectural, structural and natural operating optimization, operational energy savings potential of up to 80% can be achieved, with further savings being realized through efficient mechanical systems.
> The reduction of operational CO 2 - and other environmentally harmful emissions is also at the 100% reduction level.
> Reduction of the energy content of production and environmental emissions of materials, structures and mechanical-electrical systems.
> Maximizing investment cost-effectiveness
• Optimization of active mechanical systems (50-70% less building services equipment is sufficient)
• Finding the optimal building and mechanical systems within a given budget
• Finding the optimal trade-off: investment surplus vs. green, energy-efficient solutions
> Buildings don't use energy, only people do... Complex modelling of occupant behavior and intelligent automation of active + passive building operation can deliver additional poten-tial operational energy savings of 20-40%.
> Solving conflicting design goals, customer needs, e.g.:
• Minimum cooling demand
Maximum natural lighting
• Minimum solar heat load in summer
• Minimum heat loss in winter
Maximum solar heat gain in winter
Maximum cooling building envelope surfaces in summer
Complete design solutions: this includes both general lead design and green building certifica-tion (BREEAM, LEED, DGNB, WELL, Active House.
Project optimization: we offer consulting, expertise, project support and sectoral planning. We also provide simulation-aided design to optimize workflow and ensure project success. In the context of design verification, we carry out simulation supported building physics, climate and energy assessment of projects designed by other design teams.
Boost Your Designs: take advantage of our simulation-assisted design support to take your special designs to the next level.
Design verification with help of simulation modelling in structures and engineering systems to save investment costs.
Smart Building Management: We offer comfort and energy monitoring solutions for completed projects to ensure that implemented projects run efficiently.
Optimizing thermal comfort and air quality
> Maximum thermal comfort level (number of persons dissatisfied PPD, predicted mean vote PMV) all year round
> Minimized CO 2 -concentration to improve air hygiene
> Minimum draught effect (DR index)
Optimising visual comfort and daylighting
> Maximum daylight facotr (DF) distribution in overcast sky conditions to reduce artificial lighting
> Maximum daylight autonomy (DA) on an annual basis at clear skies to reduce artificial lighting
Optimization of passive systems
> Climate zone-based space organization and building body shape design for minimum energy demand, passive heating/cooling and optimal natural ventilation and lighting
> Thermal and environmental design and optimization of climate-adaptive building envelope structures
> Optimization of glazed structures in terms of heat gain-heat loss and lighting
> Optimization of shading technology to control light and heat loads in summer and to reduce cooling load
> Determining the optimum glazing ratio from a photometric and energy point of view
> Aerodynamic shape design, fluid dynamic-optimized mass shaping and development-optimization of natural ventilation structures and systems
Optimization of active systems
> Comparative analysis of heat transfer, heat generation and energy supply systems
> Performance and load reduction of heating and cooling systems
> Building energy calculation ÉKM 9/2023 (V.25.) – based on dynamic simulation
> Detailed building services system and automation modelling to create an optimum operation plan for heating, cooling and ventilation, as well as reduction of operational energy consumption
Buildings that give more than they take
Hungar y's first energy-positive facility was completed in 2012. It was developed using the Energy Design planning method that applies building modelling supported by dynamic climate and energy simulations. Already at an early stage of the design process, complex, exact feedback on the consequences of different space configurations, materials and structures is provided in the form of indoor comfort and energy data. A detailed analysis has been carried out to assess the performance and energy use of different concepts and optimization development steps. The optimized model and plan of the project were validated by IDA ICE thermodynamic simulations.
A further specialty is a natural, passive ventilation providing tower-chimney system (passive air conduction system PACS). In order to investigate the design solutions in a comprehensible way, a four-step aerodynamic algorithm was applied in the form of simplified calculations, wind tunnel model experiments, CFD simulations and in situ field data collection. This 'validation platform' not only allows proven claims to be made about the aerodynamic character of the building, but also allows the impact of changes during design and construction to be reliably quantified and evaluated. The aserodynamic optimization improves thermal comfort, air quali-ty and reduces final energy consumption of ventilation and cooling by the mechanical equipment.
WINERY BOUTIQUE
A wine technology facility with highly energy -conscious, sustainable and environmentally friendly features in the center of the famous Villány wine region. The 2-storey basement and ground-floor industrial section is set 1 level into the ground, a solution that is beneficial from both functional and building climate point of view, due to the buffering effect of the large air volume and the heat storage and cooling effect of the walls adjacent to the ground.
The larger 1st floor level is necessarily cantilevered, with cantilevered floor slab/room extensions on one side in an L-shape or on two sides in a T-shape. As such a shape in buildings causes higher heating demand, it seemed to be a logical step to optimize the cantilevered geometry with higher thermal bridges and to close the L-shape of the cantilevers in an as short as possible way, with the smallest possible external boundary surface.
The result was a building shape with an upwardly spreading 'ship' shape. Case studies supported by dynamic building energy and cli-mate simulations showed significant improvements in energy demand. Reductions of 24% in heating, 9% in cooling and 28% in total energy use were achieved. The optimization was carried out using dynamic thermal building simulations (IDA ICE).
Year:
Function: Area:
Location: 2024
Winery, boutique hotel and panorama restaurant
1100 m2
Villány, Hungary
The aerodynamic concept of the building operates a passive ventilation system. Intake and exhaust chimney structures ensure natural air change in the basement rooms. Several versions have been quantified and analyzed by means of computational fluid dynamic simulations (CFD, Ansys Fluent). Acc. to the modern wine-making philosophy and hygiene considerations, the basement rooms (barrel cellar and technology hall) should possess a relatively dry interior air that is to be achieved by natural ventilation. The attic contains the fresh air intake and air exhaust structures of the natural ventilation system. In case of wind, the fresh air is conducted into the basement through the intake vents.
Architecturally, a 'negative pyramid' shaped open shading structure has been created here – in contrast to the 'positive pyramid' geometry of the built-in enclosed space of the restaurant. Here, a ventilation chimney (exhaust air from the basement) has been placed next to the elevator shaft. A draft enhancing wind deflector structure is integrated into the top of the chimney that causes in windy conditions a 'Venturi' effect, accelerating the flow velocity at the top of the chimney, creating a suction effect on the used air in the basement rooms. In calm conditions, only the thermal buoyancy effect of the ventilation chimney acts as a driving force for convective outflow.
This system is designed to create air exchange and a certain reduction in internal relative humidity during transient and mild summer operating periods, and to provide night-time air exchange and passive cooling during the hot summer period. The 'positive pyramid' shape is also essential for draught intensification, as the ventilation chimney needs to be surrounded by wind deflection surfaces at different inclined angles in the aerodynamically optimal case.
Thermal simulation model, hottest day, mean air temperatures [°C]
Heating and cooling load simulations vs. conventional sizing (services systems engineering) – power of the plant (heating-cooling capacities) [kW]
In the framework of the construction design phase support, after optimizing the thermal insulation, opening/window structures (glazing) and the shading technology, we performed an alternative comparative analysis of the heat emitter systems. Based on a cost analysis and return calculation, we identified the preferred heat transfer system. In addition, we conducted heating and cooling load simulations in order to accurately determine the heat-generating system and to significantly reduce the heat demand power compared to conventional building engineering sizing. It was possible to reduce the plant by 30-55% by reducing the peak loads that occur rarely and only for a brief time. After modeling, potential analysis and sizing of the nearsurface geothermal heat utilization system with ground probes, we demonstrated annual operational optimization.
With the help of automated, motorized operation of the door and window structures, or with the CO2 -based control of the ventilation technology, it was possible to achieve a 40% higher thermal comfort level in the office building, and the annual final operating energy consumption of all heating + cooling + ventilation was reduced by nearly 10%.
The most important result of the fluid dynamics simulations was the functionality test and vali-dation of the heating-chilling beam arrangement, and the ventilation system specified by the building services system engineer-designer in a typical meeting room. From the point of view of thermal comfort, we examined the draft effect, i.e. the quantified mathematical description of the comfort experience (draft effect = DR index). The emerging flow patterns and turbulence flow systems give a reassuring picture of the artificial ventilation of the meeting room, the development of comfortable air velocities, – around both tables there is not only a draft effect of less than 10, but less than 5%, therefore it falls into the highest comfort category.
Along the edges of the heating-chilling beam equipment the reheated-recooled fresh air supply (primary air jet) is blown into the room in a tangential manner, in lateral, horizontal direction, and at the same time spreads the incoming air flow. Along the side walls and along the separable central axis of the meeting office space, the air flow avoids direct contact with the bodies of the people sitting at the meeting table, and then along the people and furniture, the rising, mixed internal air cools with a comfortable, average air speed of 0.025 – 0.05 m/s, and refreshes the indoor air quality.
3D flow diagram, so-called streamline representation of a meeting room, where each line symbolizes the path generated from the starting point for the air particles.
MSZ EN 16798 (II class) working period interval (db h)
The “air extraction” takes place along the lower surface of the heatingcooling beam equipment placed on the ceiling. The simulation also proves that the data provided by the distributors and manufacturers is reliable, but its detail and analyzability is far behind CFD simu-lations.
In this industry hall project, we applied ancient, traditional ventilation and cooling principles in a modern context, harnessing natural wind energy and currents, using the right size and number of aerodynamically optimal positioned chimney structures. The passive air conduction system (PACS) consists of several separate chimney structures, some on the ground floor and another group on the upper floor, supplying fresh air to the manipulation space in case of wind. The fresh air introduced and conducted down via the chimneys is then flushed through the interior space and leaves the building through the façade openings as used, exhaust air. Wind pressure induces natural air exchange: wind induction blows fresh air in through openings at the top of the chimneys, and used air is let out from the building through facade integrated windows. The concept is a traditional, ancient technology combined with modern, high-tech automation.
The openings are industrial louvre structures. In the case of calm winds (wind ~ 0 m/s), the internal air flow system reverses its direction (bidirectional flow system) and fresh air can now enter through the facade openings and the warmed, used air exits through the vertical chimneys, through the upper louvre structures, over the roof. The passive ventilation chimney system provides an air flow rate of 170 000 m3/h (ground floor 4.2 1/h) and 200 000 m3/h (1st floor 4.9 1/h). The natural ventilation system operates from March to November at 100% intensity.
Year: Function:
Location: 2021–Industrial park 29 hectares Vác, Hungary
Area:
Compared to the case without natural ventilation (air handling units work all year round), the PACS model achieved a total of 70% final energy and operating cost savings.
A significant part of the building complex is a building under monument and heritage protection the historical city core. In the new educational building wing, the wall to window ratio was optimized in an orientation-dependent manner using thermal simulations (IDA ICE). The glazing ratio of the facades with different orientations is diverse, from 25 to 90%, in order to ensure that the natural lighting conditions, daylight factor and daylight autonomy reach the highest possible values, while thermal comfort does not decrease. At the same time, the best performance could be achieved in terms of final energy demand.
Beside the optimization of the thermal insulation, glazing and shading technology, we also sized the heat demand using thermal building simulations, 30% heating and cooling capacity reduction was possible and hence downsizing of the heat generating center’s power, as well as investment costs savings. On the one hand this is due to the detailed, realistic dynamic, time-dependent, high-resolution simulations, and on the other hand to the ability to reduce peak loads that occur rarely and only for short periods of time.
The project was designed according to the criteria of the targeted BREEAM "very good" rating, and in this framework, we also performed a "climate resilience" test, simulating a global warming scenario 15 years into the future (2038). Based on the thermal modeling and simulation of the climate change scenario, it is visible that the increase of the internal air temperature analyzed at the room-level resolution does not exceed the value of 2 K. In most cases, the degree of warming is in the 0.5-2 K range (max. air temperature 27-29°C), in offices and classrooms.
Thermal simulation model of the existing building parts A and B1, which are under historical monument protection
Building G climate change simulationMaximum air temperatures 2023 vs 2038
Thermal simulation model of the new educational G building section
Building G climate change simulationdeltaT warming rate of indoor spaces
Kiszolgáló funkció Közlekedő funkció Tanterem Közlekedő funkció Közlekedő funkció Multifunkcós terem Kiszolgáló funkció Közlekedő funkció Tanterem Iroda Iroda Iroda Kiszolgáló funkció Szekcióterem Közlekedő funkció Kiszolgáló funkció Közlekedő funkció Kiszolgáló funkció Közlekedő funkció Közlekedő funkció Közlekedő funkció Ruhatár Hall
Kiszolgáló funkció Tanterem Közlekedő funkció Közlekedő funkció Kiszolgáló terem Tanterem Iroda Iroda Kiszolgáló funkció Kiszolgáló funkció Kiszolgáló funkció Ruhatár Közlekedő funkció Kiszolgáló funkció Ruhatár
Year:
Due to the geographical position (Dubai) the Sun position is so high especially during summer, that the building envelope, including facades to all orientations are barely exposed to direct sunlight or exposed only for short periods of the day which results in less dependency on the building materials, window to wall ratio and even on the existence of external shading when targeting direct overheating.
It results in not only in high demand of cooling energy, but also in a high demand of operation of the mechanical ventilation system. Due to the long necessity of operating the mechanical ventilation system, it is highly recommended that this system should be controlled based on the real demand. The most effective controlling considers the CO2 level measured in the actual spaces and the amount of air to be supplied is provided by a VAV system instead of a regular CAV solution. With this on demand method the saving in cooling energy can reach up to 61%.
Besides the on demand operation of mechanical ventialtion there is still a chance for natural ventilation by simple window opening or by naturally ventilated ducts utilizing thermal uplift phenomenon in 4-5 months which should be controlled based both on the internal and the external temperature. With this additional natural ventilation method the saving in cooling energy can reach up to 19% compared to the already optimized CO2 based VAV ventilation system.
Father of eight children, graduated in 1997 (Budapest University of Technology, Faculty of Mechanical Engineering) with a master’s degree in Building Services Engineering. He worked as a product developer during university and as a designer after graduation. He has been a product manager, construction project manager, technical manager, responsible supervisor, technical inspector, EU energy auditor, MEP leader. After international and national prestigious projects, he joined TSPC in March 2021, as Head of Sections and Project Manager, where he is responsible for the professional communication between the sectors, and Deputy Head of the most optimal environmental design and building physics simulation group. He is committed and motivated to develop professionally. His aim is to create a professional-economic harmony and to strengthen the company as a “building engineer”.
english, german, french, hungarian
CEED Building Physics Simulation Specialist
He studied Architecture in Würzburg, Kassel and Clima Desing at the Technische Universität München, Germany. After a professional educational collaboration at Denver Metropolitan University in Colorado, his practical work has resulted in buildings in Hungary: Holcim Hungary industrial and office building, the renovation of the first residential building in Hungary into an Active House, and a climate-adaptive adobe office building and conference center with a movable building envelope, as well as the design of a 118-unit near-zero energy residential complex (under construction). He has been a subcontractor of TSPC since 2017 and joined the team as an internal collaborator in 2022 and then again as a subcontractor in 2025 because of his teaching activities. His main responsibility was the development and management of the TSPC CEED (Climate, Energy and Environmental Design) group, which he initiated, and which is now the strongest building simulation group in the country. The main motivation is to design and renovate buildings, neighborhoods and settlements with the highest possible comfort and lowest possible energy demand, with a minimum life-cycle ecological footprint. The greatest success is achieved when a project is able to achieve energy savings of up to 80% by optimizing the architecture and structures and is able to provide the remaining internal comfort requirements with 40-60% less mechanical systems.
LANGUAGE SKILLS english, german, french, hungarian
TSPC Group provides full-service packages to its clients, accompanying them along their projects, from the first idea to implementation. TSPC is committed to sustainability, green philosophy, and innovation: We provide our solutions using the currently available highest level of expertise and technology, shaping the architecture of the future.
TSPC has organised the experts of architectural and engineering projects in a single group of companies, to provide the complex expertise and efficient work organisation required for the management of the complete project process to its clients. We provide advanced training possibilities and state-of-the-art technological background to our employees.
ARCHITECTURAL DESIGN
General design
Architectural design
Interior design
Landscape design
Urban design, city planning
BOQ (Bill of Quantities)
Feasibilty studies
Detailed construction
drawings
Construction supervision
ENGINEERING DESIGN
Structural design
MEP design (Electric engineering, HVAC design, Building services engineering)
Fire safety design
Road and public utilitiy design
Technical consultancy Geodesy
REAL ESTATE & CONSULTANCY
Feasibilty studies
Turnkey developments
Operation planning
Development site identification
Facility Management
Due diligence
ENGINEERING DESIGN
Climate and energy optimized planning and design supoort
Comfort, energy and aerodynamic simulations
Enviroment friendly design and consulting
Green certification based design and survey
ArchiCAD, Revit, Solibri, Nemetschek and Tekla support in BIM
BIM consultancy
BIM management
BIM modelling and 2D file preparation
Sharepoint, Plan-doc
Point cloud processing
On-site BIM Solutions
PROJECT MANAGEMENT SURVEILLANCE & DIAGNOSTICS
Public procurement
consultancy
Scheduling
Budgeting design & control
Expert Services
& Consultancy
Designer supervision
Technical supervision
Organizational plans
Building survey
Geodesy
Point cloud survey
3D laser scanning
Energy analysis of existing buildings
Building energetics
Structural diagnostics
HUNGARY > Budapest, Győr, Debrecen
GERMANY > Munich
SLOVAKIA > Komárno
CROATIA > Zagreb
HONG KONG
TSPC GmbH
Leopoldstraße 256., 80807, Munich info@tspcgroup.de
TSPC d.o.o.
Črnomerec 63, HR-10120 Zagreb
TSPC Group HONG KONG
TSPC Group
H-9022 Győr, Dunakapu tér 7. info@tspc.hu
+36 96 200 651
+36 1 800 9192
Dunajské nábrežie 4726, 945 01 Komárno info@tspc.hu
TSPC Group
4025 Debrecen, Barna utca 23. info@tspc.hu
TSPC Group
H-1053 Budapest, Magyar u. 36. info@tspc.hu
+36 1 800 9191
+36 1 800 9192