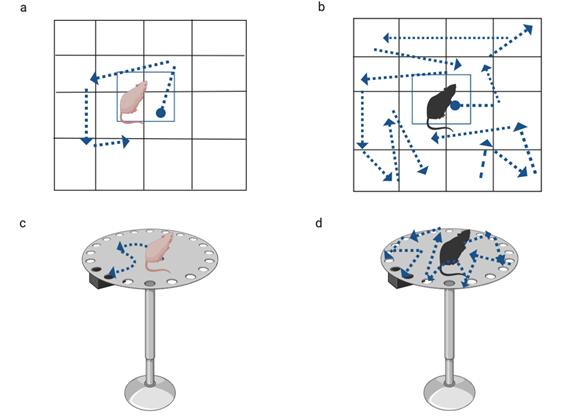
25 minute read
Kynurenine Pathway and Gut Microbiota: Treatment Applications for Schizophrenia
Ali Abdolizadeh
Previous studies reported increased pro-inflammatory markers in schizophrenia (SCZ) patients (Müller 2018). In addition, altered tryptophan (Trp) metabolism and dysregulation of its metabolites have been observed in inflammation-associated diseases (Dantzer 2017). The gut microbiota modulates different pathways of Trp metabolism and influences brain function (Gao et al. 2020). Significant contributions of dysregulated gut microbiota to neuropsychiatric disorders through the gut-brain axis have been highlighted in the past decade (Petra et al. 2015). However, it is still unclear whether microbiota alteration itself leads to neuropsychiatric diseases, or in turn, microbiota dysbiosis is a result of mental disorders. The original paper sought to further investigate the causation of this process (Zhu et al. 2019). They performed fecal microbiota transplantation (FMT) from antipsychotic-free individuals with schizophrenia (SZC) and healthy controls (CS) into specific pathogen-free (SPF) mice. Following FMT, behavioural tests revealed impaired spatial learning and memory, increased exploratory activity, and enhanced rearing behaviour in SPF mice treated with fecal-derived microbiota from SCZ individuals. Moreover, kynurenine (Kyn) and kynurenic acid (Kyna) levels of Trp metabolites were elevated in both peripheral tissues and the central nervous system of SCZ mice. Nonetheless, the exact mechanism by which the gut microbiota contributes to schizophrenia pathogenesis remains unclear. The current paper aims to review the role of the gut microbiota in brain functions, focusing on the Trp metabolism and Kyn pathway. This review further elucidates the link between Kyn metabolites and schizophrenia for potential therapeutic targets.
Advertisement
Key words: schizophrenia (SZC), tryptophan (Trp) metabolism, microbiota, brain-gut axis, impaired learning and memory, kynurenine (Kyn), Kynurenic acid (Kyna), fecal microbiota transplantation (FMT).
Introduction
The gut microbiota is the community of all microorganisms, mainly bacteria, that live in our intestine (Turnbaugh et al. 2007). Accumulating evidence indicates that environmental factors, such as epigenetics, the mode of delivery, infant feeding, diet, antibiotics, may change the composition and diversity of the gut microbiota (Wen and Duffy 2017). Studies in mice and humans have revealed that the gut microbiota regulates signalling between the brain and the gut, contributing to the healthy development of the brain (Dinan and Cryan 2017; Carlson et al. 2018; Sharon et al. 2016). An earlier study showed that microbiota has a significant role in mediating the stress response through the hypothalamic-pituitary-adrenal (HPA) axis (Sudo et al. 2004). The primary microbiota metabolites, short-chain-fatty acids (SCFA), are also essential for the normal function of the nervous system (Silva, Bernardi, and Frozza 2020). Moreover, gut microbiota plays an important role in the regulation of proteins involved in synaptic transmissions, such as synaptophysin (Heijtz et al. 2011). Further studies indicated that the gut microbiota is required for microglial cells’ function, and dysregulated microbiota may lead to neuroinflammation and mental disorders (Erny et al. 2015). Vagus nerve is another pathway by which microbiota influences the gut-brain crosstalk (Bravo et al. 2011). Additionally, Trp metabolism, which is known to be important in inflammation and brain function, is regulated by the gut microbiota (Gao et al. 2020). Thus, since our gut microbiota is involved in a broad spectrum of pathways to influence our brain function, it can be plausibly targeted to help us better understand the pathogenesis of psychiatric disorders such as schizophrenia. Schizophrenia (SCZ) is known to be one of the most complex mental disorders, and the symptoms may fall into three subcategories; positive, negative, and cognitive (Kahn et al. 2015). The most common characteristics of positive symptoms are hallucinations and delusions, while anhedonia and lack of motivation are considered as negative symptoms. Individuals with schizophrenia may also experience cognitive symptoms such as cognitive dysfunctions (Kahn et al. 2015). A metaanalysis of 12 studies illustrated the importance of genetic predisposition and environmental factors for the development of schizophrenia (Sullivan, Kendler, and Neale 2003). Therefore, it is conceivable that the gut microbiota, which is highly regulated by environments, to be involved in the SCZ-associated symptoms. In this context, recent studies used 16S rRNA sequencing to find a diagnostic biomarker in the gut microbiota of SCZ patients. They were able to observe significant differences between the gut microbiota of SCZ patients and healthy controls (Shen et al. 2018; Nguyen et al. 2019). Miller et al. (2011) conducted a meta-analysis to investigate changes of proinflammatory cytokines in individuals with SCZ. They found elevated levels of TNF-alpha, IL-6, and IL-1B in SCZ patients, suggesting schizophrenia as a neuroinflammatory disorder. Despite a slight discrepancy found between studies (Erhardt et al. 2001; Szymona et al. 2017), the impacts of inflammation on Trp metabolism and SCZ pathogenesis have been widely accepted (Pedraz-Petrozzi et al. 2020). Previous studies showed that microbiota-derived metabolites are involved in the regulation of inflammations through acting on Trp metabolism (Lamas et al. 2016). Furthermore, the microbiota can mediate conversions of Trp into the downstream molecules, influencing the release of neurotransmitters, such as glutamate, serotonin, and dopamine (Agus, Planchais, and Sokol 2018). In the original paper, Zhu et al. (2019) sought to examine whether fecal-derived microbiota of humans can affect the normal brain function of the mice. Subsequently, they performed FMT from SCZ and healthy individuals into SPF mice. Impaired spatial learning and memory and increased exploratory behaviour, known as SCZ-associated behavioural abnormalities were observed in the mice treated with dysregulated fecalderived microbiota from SCZ patients. Further analysis revealed that the Kyn pathway of Trp metabolism in SCZ mice is upregulated in both peripheral tissues and the central nervous system, leading to neurotransmitter alterations.
Results
SCZ-like behaviors in SPF mice treated with FMT from SCZ patients
To examine the effects of the human gut microbiota on mice, behavioural tests, such as open field test (OPT) and Barnes maze (BM) task, were conducted following transplantation. OPT illustrated that SCZ mice, compared to HC mice, exhibited hyperlocomotor activity since the number of lines crossed in the field and the total distance moved were all increased in the given period (Fig.1 a and b). In addition, the number of rearing events in SCZ mice was elevated, suggesting hyper-exploratory behaviours. The Barnes Maze task was conducted to assess the learning and memory of the mice (Fig. 1 c and d). In the BM task, escape latency measurements indicated that SCZ mice had prolonged latency to find the escape box after trial training. The results indicate spatial learning and memory impairments as another feature found in SCZ mice. In line with these findings, Zheng et al. (2019) showed that the behaviours of mice treated with the microbiota of SCZ patients were associated with SCZ symptoms, such as increased hyperactivity. It is worth mentioning that locomotor activity and exploratory behaviours as positive symptoms and impaired memory as a negative symptom have been previously reported as the behaviours associated with the animal model of SCZ (Winship et al. 2019).
Fig.1) OFT (a and b) and BM task (c and d) were performed to examine mice behaviours after FMT. HC mice and SCZ mice are shown in light pink and black, respectively. OFT showed increased locomotor activity and rearing events in SCZ mice (a) compared to HC (a). In BM task, SCZ mice exhibited impaired spatial learning and memory (d), in comparison to HC mice (c).
Upregulation of the peripheral Kyn-Kyna pathway of Trp metabolism in SCZ mice
Upon dissecting the mice’s peripheral and brain tissues, enzymelinked immunosorbent assay (ELISA), liquid chromatographymass spectrometry (LC/MS), and qPCR were used to measure downstream molecules concentrations and gene expressions of the enzymes present in Trp metabolism. It is noted that 5-hydroxytryptophan (5-HT) and Kynurenine (Kyn) are known as the two pathways of Trp metabolism (Höglund, Øverli, and Winberg 2019). Zhu et al. (2019) found that Trp levels in serum and peripheral tissues, such as intestine, spleen, and liver of the SCZ mice were decreased. Reduced 5-HT levels in serum and intestine of the SCZ mice were also observed. In addition, the expression of tryptophan hydroxylase (TPH-1), which controls the production of 5-HT from Trp, decreased in the peripheral tissues, suggesting that Trp metabolism in the 5-HT pathway is downregulated. On the other hand, they reported increased Kyn levels in serum and peripheral tissues. Consistently, indoleamine 1,2- dioxygenase 1 (IDO-1) enzyme that converts Trp to Kyn were overexpressed (Fig.2 a). Kyn can be further metabolized into two branches; Kynurenic acid (KA) and 3-hydroxykynurenine (3-HK) (Höglund, Øverli, and Winberg 2019). They reported unchanged 3-HK in peripheral tissues, whereas the level of Kyna was higher in serum and peripheral tissues. In agreement with the increased Kyna, mRNA levels of kynurenine aminotransferase (KAT -II), which act as Kyn-Kyna conversion enzyme, were elevated in the liver of the SCZ mice (Fig.2 b). In consistent with the unchanged 3-HK, the Kynurenine-3- monooxygenase (KMO) enzyme that catalyzes Kyn to 3-HK did not change. These results indicated that the peripheral Kyn-Kyna pathway of Trp metabolism is activated in SCZ mice, compared to HC. A recent study also showed that elevated Kyna is associated with cognitive impairments in individuals with SCZ (Huang et al. 2020). Although many studies indicated that elevated immune response and inflammatory cytokines are associated with the pathogenesis of schizophrenia (Müller 2018), Zhu et al. (2019) could not find any significant differences in inflammatory markers between SCZ and HC mice. Thus, they postulated that direct or indirect effects of microbiota induced those changes in Trp metabolism. Fig.2 Expression of IDO and KAT-II in the Kyn pathway of Trp metabolism were measured immediately after and ten days after FMT. IDO mRNA significantly increased in the colon, liver, and spleen of SCZ mice compared to HC mice (a). mRNA levels of KAT-II were elevated only in the liver (b). This figure was pre
sented in the original paper (Zhu et al. 2019).
Increased Kyn-Kyna pathway of Trp metabolism in the brain of SCZ mice
Zhu et al. targeted three brain regions of SCZ mice; prefrontal cortex (PFC), striatum, and hippocampus, to measure metabolites and enzymes involved in the Trp metabolism (Fig.3). The same tools and techniques previously mentioned were used to do the experiment. They similarly observed elevated Kyn-Kyna pathway of Trp metabolism in the brain of the SZC mice. In line with this finding, high brain Kyna in SCZ was also found in previous studies (Schwarcz et al. 2001). Moreover, 3-HK levels were decreased in PFC, striatum, and hippocampus of SCZ mice, while the mRNA levels of KMO remained almost the same, compared to HC. Even though the expression of IDO, which converts Trp to Kyn, did not increase, they found elevated Kyn levels in all three brain regions. Notably, increased IDO expressions were previously reported in the brain of the SCZ individuals (Miller et al. 2004). In addition, as the mRNA levels of KAT-II increased, they found the Kyna level was also elevated in the mentioned brain areas of SCZ mice. TPH-1 expression, which is responsible for Trp/5H-T conversion, increased in the hippocampus of SCZ mice, and subsequently, 5-HT was elevated. The increased 5-HT level was observed in the striatum as well. Furthermore, they reported elevated dopamine levels in the striatum and PFC and increased glutamate in PFC.
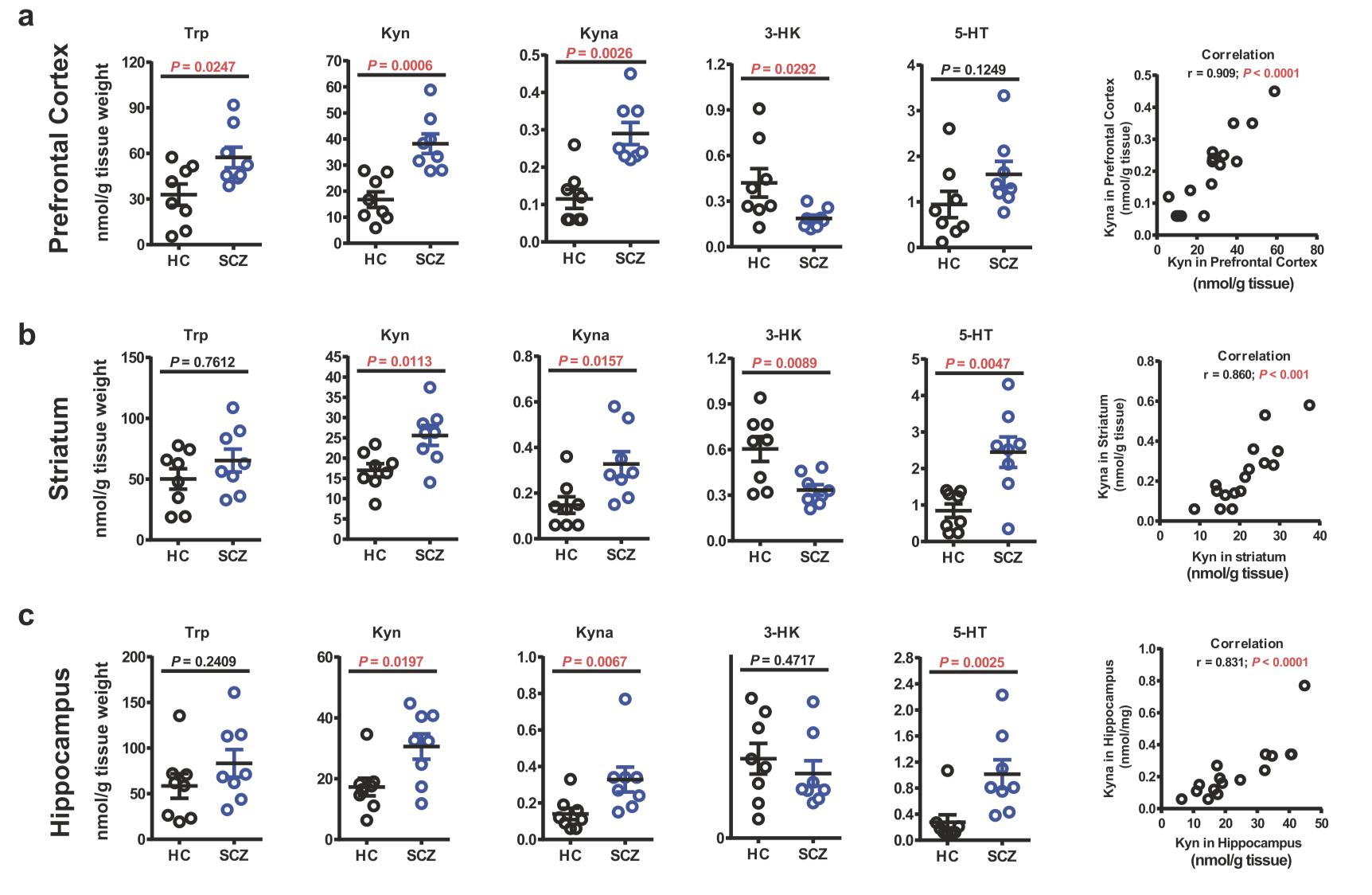
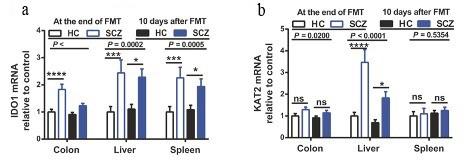
Fig.3 Levels of Trp metabolites in PFC (a), striatum (b), and hippocampus (c) of SCZ and HC mice are illustrated above. Increased levels of Kyn and Kyna are noted in all three brain regions of SCZ mice, whereas 3-HK decreased, showing upregulation of central Kyn-Kyna pathway of Trp metabolism. Increased 5 -HT in striatum and hippocampus may explain positive symptoms in SCZ mice. This figure was presented in the original paper (Zhu et al. 2019).
Discussion
Zhu et al. illustrated that altered gut microbiota from SCZ patients transplanted into SPC mice upregulated both peripheral and central Kyn-Kyna pathway of Trp metabolism. Correspondingly, these changes were associated with SCZ-like symptoms. Indeed, they provided new findings that may have implications for future researches and therapeutic targets. The gut microbiota can regulate Trp metabolisms in three different pathways; indole, 5-HT, and Kyn (Agus, Planchais, and Sokol 2018) that the two later pathways will be discussed in this section. Interestingly, our gut microbiota has regulatory effects on the enzyme TPH (Yano et al. 2015; Reigstad et al. 2015), which converts Trp to 5-HT. It has been reported that microbiota metabolites, such as short-chain fatty acids, are involved in stimulating the expression of TPH (Yano et al. 2015). In line with this observation, a recent study showed 5-HT deficiency in germ-free mice (Reigstad et al. 2015). These findings may explain the original paper’s result indicating decreased peripheral 5-HT in SCZ mice with altered gut microbiota. In the Trp metabolism, IDO is the enzyme that catalyzes Trp to Kyn (Thomas and Stocker 1999). Recent studies have shown that IDO is stimulated by inflammatory cytokines, such as INF-gamma (O’Connor et al. 2009). Zhu et al. (2019) did not report increased inflammatory markers in SCZ mice, while the expression of IDO increased in the periphery. Therefore, they suggested that the influence of dysregulated microbiota led to such results. In parallel with this postulation, the effects of the gut microbiota on IDO activity were reported in Valladares et al. ‘s paper (2013). It should be noted that Kyn can readily cross the bloodbrain barrier (BBB) using transporters (Fukui et al. 1991). This process is mediated by the microglia cells (Schwarcz and Pellicciari 2002), and interestingly maturation of these microglia is highly dependent on the gut microbiota (Erny et al. 2015). In addition, the ability of Kyn to cross the BBB may account for the increased brain Kyn, while IDO expression remained unchanged in the brain. Further, Kyn is metabolized in two distinct pathways, resulting in Kyna and 3-HK productions by the enzymes KAT-II and KMO, respectively (Guidetti et al. 2007; Kindler et al. 2019). Downstream, 3-HK leads to the formation of neurotoxic quinolinic acid (QA), which is known to be an N-methyl-daspartate (NMDA) receptor agonist. However, Kyna acts as an antagonist of the NMDA receptor (Schwarcz et al. 2012). Even though Kyna can reduce excitotoxicity effects of QA, increased Kyna level has been associated with impaired cognition in SCZ patients (Huang et al. 2020). Researchers suggested that inhibition of Kyna in the brain could be a potential target to restore cognition in SCZ patients (Wonodi and Schwarcz 2010). In this regard, Kozak et al. (2014) showed that KAT-II inhibitors decreased brain Kyna level and improved cognitive functions in mice. It is worth mentioning that Olney and Farber (1995) had proposed that the dysfunction of glutamate receptors is linked with the pathogenesis of SCZ. Indeed, they showed that blockade of glutamate receptors resulted in the loss of inhibitory effects of GABAergic neurons, and further increased the release of glutamate in the brain. These findings may explain increased glutamate levels observed in the brain regions of SCZ mice, which are associated with positive symptoms. Studies have also demonstrated that high Kyna, as an NMDA receptor antagonist, can stimulate dopaminergic neurons in the midbrain of SCZ individuals (Erhardt and Engberg 2002). In line with this observation, Zhu et al. (2019) reported increased dopamine in striatum and PFC of SCZ mice. Reviewing the original paper’s results and the relevance demonstrates that the Kyn pathway of Trp metabolism can be considered an effective therapeutic target for SCZ patients. In addition, once more, the importance of the gut microbiota in psychiatric disorders has been highlighted.
Critical Analysis
A recent study had indicated that germ-free mice exhibited depressive-like symptoms when received microbiota from individuals with major depressive disorder (Zheng et al. 2016). In this context, the original paper supported the result that microbiota can have casual effects in developing psychiatric disorders. Zheng et al. (2019) very recently performed FMT from SCZ individuals to germ-free mice and observed SCZ-associated symptoms. In contrast to the present paper, Zheng et al. (2019) reported reduced anxiety behaviours and increased startle response in SCZ mice. As discussed in the original article, the authors suggested the use of germ-free vs. SPF mice in their experiments may account for these discrepancies. Collecting fecal samples from a small number of individuals and using the same strain and gender of mice for FMT make the results difficult to be generalized. In addition, the authors did not address whether the SCZ individuals that donated their feces were at the early or late stage of illness. This may be important since the gut microbiota may differ throughout the disease and subsequently have different impacts on Trp metabolism, Kyn and Kyna levels. The authors should have divided SCZ mice into two groups and then induced inhibition to the Kyn pathway of Trp metabolism. This would allow them to compare their SCZ-like behaviours and ensure that the effects of altered gut microbiota on the Kyn pathway lead to such results. Furthermore, the authors did only focus on the positive and cognitive symptoms of SCZ mice, while negative symptoms were not examined.
It is worth emphasizing that increased Kyna levels are not observed in all cases of SCZ. For instance, in their clinical research, Szymona et al. (2017), reported significantly reduced Kyna in the periphery and elevated 3-HK in individuals with SCZ. Similarly, when Mynt et al. (2011) looked at the Kyn pathway of Trp metabolism in drug-free SCZ vs. medicated SCZ, they report-
ed that Kyna levels decreased, while 3-HK increased in the plasma of SCZ patients. Thus, it is plausible to consider the imbalanced Kyna/3-HK ratio, increased or decreased in either direction as an implication for SCZ development for future investigations.
Future Directions
For the first time, the original paper postulated the casual effects of altered gut microbiota on the Kyn pathway of Trp metabolism and developing SCZ (Zhu et al. 2019). Therefore, future studies will be conceivable to delineate the exact mechanisms involved in this process, validate the results, and develop effective treatments for SCZ. Future investigations with larger sample sizes and balanced recruitment of both male and female mice are required for fecal microbiota transplantation. Phases of illness, acute vs. residual, or the disease progression must be taken into consideration when choosing SCZ subjects as donors in the experiment. To further confirm that the casual links between altered gut microbiota and SCZ pathogenesis occur through the Kyn pathway, researchers should inject NDMA receptor agonists or KAT-II inhibitors to the mice immediately after treatments with microbiota from SCZ individuals. Inhibition of KAT-II would result in decreased brain Kyna and allow researchers to realize if it can improve mice's SCZ-like behaviours compared to HC. In other words, they would then be able to conclude better if altered gut microbiota disrupting the Kyn pathway is responsible for developing such behaviours. If decreased Kyna, as a result of KAT-II inhibition, did not improve SCZ-associated behaviours in mice, it would suggest that the gut microbiota can induce their effects through different pathways than Trp metabolism. Moreover, the sucrose preference test should be obtained to evaluate the negative symptoms of the mice treated with SCZ microbiota. This would help researchers understand whether the upregulated Kyna induced by altered gut microbiota is only associated with psychotic episodes of SCZ or can also be related to negative symptoms. Additionally, researchers should measure further downstream Kyn metabolites in SCZ mice, such as QA and Xanthurenic acid (XA), which are known to fluctuate in SCZ patients (Fazio et al. 2015). The results would allow them to understand if altered gut microbiota has an impact on SCZ pathogenies by acting on QA or XA metabolite and may introduce a new target for developing treatments for SCZ. Although it is difficult to determine the healthy composition of microbiota in humans, identification of the gut microbiota in SCZ individuals may have implications as a marker for diagnosis, prognosis, and even treatment response for SCZ. It is not apparent if a particular group of gut microbiota alone contributes to neuropsychiatric disorders, or the presence of certain bacteria with the effects of other microbes together leads to such results. Thus, it is plausible to investigate the patterns of gut microbiota and observe their relations with other bacteria in
10.
11.
12.
13.
14.
15.
16.
17. Agus, Allison, Julien Planchais, and Harry Sokol. 2018. “Gut Microbiota Regulation of Tryptophan Metabolism in Health and Disease.” Cell Host & Microbe 23 (6): 716–24. https://doi.org/10.1016/j.chom.2018.05.003. Bravo, Javier A., Paul Forsythe, Marianne V. Chew, Emily Escaravage, Hélène M. Savignac, Timothy G. Dinan, John Bienenstock, and John F. Cryan. 2011. “Ingestion of Lactobacillus Strain Regulates Emotional Behavior and Central GABA Receptor Expression in a Mouse via the Vagus Nerve.” Proceedings of the National Academy of Sciences of the United States of America 108 (38): 16050–55. https:// doi.org/10.1073/pnas.1102999108. Carlson, Alexander L., Kai Xia, M. Andrea Azcarate-Peril, Barbara D. Goldman, Mihye Ahn, Martin A. Styner, Amanda L. Thompson, Xiujuan Geng, John H. Gilmore, and Rebecca C. Knickmeyer. 2018. “Infant Gut Microbiome Associated with Cognitive Development.” Biological Psychiatry 83 (2): 148–59. https://doi.org/10.1016/j.biopsych.2017.06.021.
Dantzer, Robert. 2017. “Role of the Kynurenine Metabolism Pathway in Inflammation-Induced Depression –Preclinical Approaces.” Current Topics in Behavioral Neurosciences 31: 117–38. https://doi.org/10.1007/7854_2016_6.
Dinan, Timothy G., and John F. Cryan. 2017. “Gut Instincts: Microbiota as a Key Regulator of Brain Development, Ageing and Neurodegeneration.” The Journal of Physiology 595 (2): 489–503. https://doi.org/10.1113/JP273106.
Erhardt, S., K. Blennow, C. Nordin, E. Skogh, L. H. Lindström, and G. Engberg. 2001. “Kynurenic Acid Levels Are Elevated in the Cerebrospinal Fluid of Patients with Schizophrenia.” Neuroscience Letters 313 (1–2): 96–98. https://doi.org/10.1016/s0304-3940(01)02242-x. Erhardt, S., and G. Engberg. 2002. “Increased Phasic Activity of Dopaminergic Neurones in the Rat Ventral Tegmental Area Following Pharmacologically Elevated Levels of Endogenous Kynurenic Acid.” Acta Physiologica Scandinavica 175 (1): 45–53. https://doi.org/10.1046/ j.1365-201X.2002.00962.x.
Erny, Daniel, Anna Lena Hrabě de Angelis, Diego Jaitin, Peter Wieghofer, Ori Staszewski, Eyal David, Hadas Keren-Shaul, et al. 2015. “Host Microbiota Constantly Control Maturation and Function of Microglia in the CNS.” Nature Neuroscience 18 (7): 965–77. https:// doi.org/10.1038/nn.4030. Fazio, Francesco, Luana Lionetto, Martina Curto, Luisa Iacovelli, Michele Cavallari, Cristina Zappulla, Martina Ulivieri, et al. 2015. “Xanthurenic Acid Activates MGlu2/3 Metabotropic Glutamate Receptors and Is a Potential Trait Marker for Schizophrenia.” Scientific Reports 5 (December). https://doi.org/10.1038/srep177
Fukui, Shinsuke, Robert Schwarcz, Stanley I. Rapoport, Yoshiaki Takada, and Quentin R. Smith. 1991. “Blood–Brain Barrier Transport of Kynurenines: Implications for Brain Synthesis and Metabolism.” Journal of Neurochemistry 56 (6): 2007–17. https://doi.org/10.1111/ j.1471-4159.1991.tb03460.x.
Gao, Kan, Chun-long Mu, Aitak Farzi, and Wei-yun Zhu. 2020. “Tryptophan Metabolism: A Link Between the Gut Microbiota and Brain.” Advances in Nutrition 11 (3): 709–23. https://doi.org/10.1093/advances/nmz127.
Guidetti, Paolo, Laura Amori, Michael T. Sapko, Etsuo Okuno, and Robert Schwarcz. 2007. “Mitochondrial Aspartate Aminotransferase: A Third Kynurenate-Producing Enzyme in the Mammalian Brain.” Journal of Neurochemistry 102 (1): 103–11. https://doi.org/10.1111/ j.1471-4159.2007.04556.x.
Heijtz, Rochellys Diaz, Shugui Wang, Farhana Anuar, Yu Qian, Britta Björkholm, Annika Samuelsson, Martin L. Hibberd, Hans Forssberg, and Sven Pettersson. 2011. “Normal Gut Microbiota Modulates Brain Development and Behavior.” Proceedings of the National Academy of Sciences of the United States of America 108 (7): 3047–52. https://doi.org/10.1073/pnas.1010529108.
Höglund, Erik, Øyvind Øverli, and Svante Winberg. 2019. “Tryptophan Metabolic Pathways and Brain Serotonergic Activity: A Comparative Review.” Frontiers in Endocrinology 10. https://doi.org/10.3389/fendo.2019.00158. Huang, Xingbing, Wenhua Ding, Fengchun Wu, Sumiao Zhou, Shuhua Deng, and Yuping Ning. 2020. “Increased Plasma Kynurenic Acid Levels Are Associated with Impaired Attention/Vigilance and Social Cognition in Patients with Schizophrenia.” Neuropsychiatric Disease and Treatment 16 (January): 263–71. https://doi.org/10.2147/NDT.S239763.
Kahn, René S., Iris E. Sommer, Robin M. Murray, Andreas Meyer-Lindenberg, Daniel R. Weinberger, Tyrone D. Cannon, Michael O’Donovan, et al. 2015. “Schizophrenia.” Nature Reviews Disease Primers 1 (1): 1–23. https://doi.org/10.1038/nrdp.2015.67. Kindler, Jochen, Chai K. Lim, Cynthia Shannon Weickert, Danny Boerrigter, Cherrie Galletly, Dennis Liu, Kelly R. Jacobs, et al. 2019. “Dysregulation of Kynurenine Metabolism Is Related to Proinflammatory Cytokines, Attention, and Prefrontal Cortex Volume in Schizophrenia.” Molecular Psychiatry, April, 1–13. https://doi.org/10.1038/s41380-019-0401-9.
17.
18.
19.
20.
21.
22.
23.
24.
25.
26.
27.
28.
29.
30.
31.
32. Kozak, Rouba, Brian M. Campbell, Christine A. Strick, Weldon Horner, William E. Hoffmann, Tamas Kiss, Douglas S. Chapin, et al. 2014. “Reduction of Brain Kynurenic Acid Improves Cognitive Function.” The Journal of Neuroscience 34 (32): 10592–602. https:// doi.org/10.1523/JNEUROSCI.1107-14.2014.
Lamas, Bruno, Mathias L. Richard, Valentin Leducq, Hang-Phuong Pham, Marie-Laure Michel, Gregory Da Costa, Chantal Bridonneau, et al. 2016. “CARD9 Impacts Colitis by Altering Gut Microbiota Metabolism of Tryptophan into Aryl Hydrocarbon Receptor Ligands.” Nature Medicine 22 (6): 598–605. https://doi.org/10.1038/nm.4102.
Miller, Christine L, Ida C Llenos, Jeanette R Dulay, Meliza M Barillo, Robert H Yolken, and Serge Weis. 2004. “Expression of the Kynurenine Pathway Enzyme Tryptophan 2,3-Dioxygenase Is Increased in the Frontal Cortex of Individuals with Schizophrenia.” Neurobiology of Disease 15 (3): 618–29. https://doi.org/10.1016/j.nbd.2003.12.015.
Miller, Brian J., Peter Buckley, Wesley Seabolt, Andrew Mellor, and Brian Kirkpatrick. 2011. “Meta-Analysis of Cytokine Alterations in Schizophrenia: Clinical Status and Antipsychotic Effects.” Biological Psychiatry 70 (7): 663–71. https://doi.org/10.1016/ j.biopsych.2011.04.013.
Müller, Norbert. 2018. “Inflammation in Schizophrenia: Pathogenetic Aspects and Therapeutic Considerations.” Schizophrenia Bulletin 44 (5): 973–82. https://doi.org/10.1093/schbul/sby024.
Myint, A. M., M. J. Schwarz, R. Verkerk, H. H. Mueller, J. Zach, S. Scharpé, H. W. M. Steinbusch, B. E. Leonard, and Y. K. Kim. 2011. “Reversal of Imbalance between Kynurenic Acid and 3-Hydroxykynurenine by Antipsychotics in Medication-Naïve and Medication-Free Schizophrenic Patients.” Brain, Behavior, and Immunity 25 (8): 1576–81. https://doi.org/10.1016/j.bbi.2011.05.005. Nguyen, Tanya T., Tomasz Kosciolek, Yadira Maldonado, Rebecca E. Daly, Averria Sirkin Martin, Daniel McDonald, Rob Knight, and Dilip V. Jeste. 2019. “Differences in Gut Microbiome Composition Between Persons with Chronic Schizophrenia and Healthy Comparison Subjects.” Schizophrenia Research 204 (February): 23–29. https://doi.org/10.1016/j.schres.2018.09.014.
O’Connor, Jason C., Caroline André, Yunxia Wang, Marcus A. Lawson, Sandra S. Szegedi, Jacques Lestage, Nathalie Castanon, Keith W. Kelley, and Robert Dantzer. 2009. “Interferon-γ and Tumor Necrosis Factor-α Mediate the Upregulation of Indoleamine 2,3-Dioxygenase and the Induction of Depressive-Like Behavior in Mice in Response to Bacillus Calmette-Guérin.” The Journal of Neuroscience 29 (13): 4200–4209. https://doi.org/10.1523/JNEUROSCI.5032-08.2009.
Olney, J. W., and N. B. Farber. 1995. “Glutamate Receptor Dysfunction and Schizophrenia.” Archives of General Psychiatry 52 (12): 998–1007. https://doi.org/10.1001/archpsyc.1995.03950240016004.
Pedraz-Petrozzi, Bruno, Osama Elyamany, Christoph Rummel, and Christoph Mulert. 2020. “Effects of Inflammation on the Kynurenine Pathway in Schizophrenia — a Systematic Review.” Journal of Neuroinflammation 17 (1): 56. https://doi.org/10.1186/s12974-020-1721-z.
Petra, Anastasia I., Smaro Panagiotidou, Erifili Hatziagelaki, Julia M. Stewart, Pio Conti, and Theoharis C. Theoharides. 2015. “GutMicrobiota-Brain Axis and Its Effect on Neuropsychiatric Disorders With Suspected Immune Dysregulation.” Clinical Therapeutics 37 (5): 984–95. https://doi.org/10.1016/j.clinthera.2015.04.002.
Reigstad, Christopher S., Charles E. Salmonson, John F. Rainey Iii, Joseph H. Szurszewski, David R. Linden, Justin L. Sonnenburg, Gianrico Farrugia, and Purna C. Kashyap. 2015. “Gut Microbes Promote Colonic Serotonin Production through an Effect of Short-Chain Fatty Acids on Enterochromaffin Cells.” The FASEB Journal 29 (4): 1395–1403. https://doi.org/10.1096/fj.14-259598.
Schwarcz, Robert, Arash Rassoulpour, Hui-Qiu Wu, Deborah Medoff, Carol A Tamminga, and Rosalinda C Roberts. 2001. “Increased Cortical Kynurenate Content in Schizophrenia.” Biological Psychiatry 50 (7): 521–30. https://doi.org/10.1016/S0006-3223(01)01078-2. Schwarcz, Robert, and Roberto Pellicciari. 2002. “Manipulation of Brain Kynurenines: Glial Targets, Neuronal Effects, and Clinical Opportunities.” The Journal of Pharmacology and Experimental Therapeutics 303 (1): 110. https://doi.org/10.1124/jpet.102.034439. Schwarcz, Robert, John P. Bruno, Paul J. Muchowski, and Hui-Qiu Wu. 2012. “Kynurenines in the Mammalian Brain: When Physiology Meets Pathology.” Nature Reviews Neuroscience 13 (7): 465–77. https://doi.org/10.1038/nrn3257.
Sharon, Gil, Timothy R. Sampson, Daniel H. Geschwind, and Sarkis K. Mazmanian. 2016. “The Central Nervous System and the Gut Microbiome.” Cell 167 (4): 915–32. https://doi.org/10.1016/j.cell.2016.10.027. Shen, Yang, Jintian Xu, Zhiyong Li, Yichen Huang, Ye Yuan, Jixiang Wang, Meng Zhang, Songnian Hu, and Ying Liang. 2018. “Analysis of Gut Microbiota Diversity and Auxiliary Diagnosis as a Biomarker in Patients with Schizophrenia: A Cross-Sectional Study.” Schizophrenia Research 197 (July): 470–77. https://doi.org/10.1016/j.schres.2018.01.002.
34.
35.
36.
37.
38.
39.
40.
41.
42.
43.
44.
45.
46. Sudo, Nobuyuki, Yoichi Chida, Yuji Aiba, Junko Sonoda, Naomi Oyama, Xiao-Nian Yu, Chiharu Kubo, and Yasuhiro Koga. 2004. “Postnatal Microbial Colonization Programs the Hypothalamic–Pituitary–Adrenal System for Stress Response in Mice.” The Journal of Physiology 558 (Pt 1): 263–75. https://doi.org/10.1113/jphysiol.2004.063388.
Sullivan, Patrick F., Kenneth S. Kendler, and Michael C. Neale. 2003. “Schizophrenia as a Complex Trait: Evidence From a Meta-Analysis of Twin Studies.” Archives of General Psychiatry 60 (12): 1187. https://doi.org/10.1001/archpsyc.60.12.1187.
Szymona, Kinga, Barbara Zdzisińska, Hanna Karakuła-Juchnowicz, Tomasz Kocki, Martyna Kandefer-Szerszeń, Marta Flis, Wojciech Rosa, and Ewa M. Urbańska. 2017. “Correlations of Kynurenic Acid, 3-Hydroxykynurenine, SIL-2R, IFN-α, and IL-4 with Clinical Symptoms During Acute Relapse of Schizophrenia.” Neurotoxicity Research 32 (1): 17–26. https://doi.org/10.1007/s12640-017-9714-0.
Thomas, S. R., and R. Stocker. 1999. “Redox Reactions Related to Indoleamine 2,3-Dioxygenase and Tryptophan Metabolism along the Kynurenine Pathway.” Redox Report 4 (5): 199–220. https://doi.org/10.1179/135100099101534927.
Turnbaugh, Peter J., Ruth E. Ley, Micah Hamady, Claire M. Fraser-Liggett, Rob Knight, and Jeffrey I. Gordon. 2007. “The Human Microbiome Project.” Nature 449 (7164): 804–10. https://doi.org/10.1038/nature06244.
Valladares, Ricardo, Lora Bojilova, Anastasia H. Potts, Evan Cameron, Christopher Gardner, Graciela Lorca, and Claudio F. Gonzalez. 2013. “Lactobacillus Johnsonii Inhibits Indoleamine 2,3-Dioxygenase and Alters Tryptophan Metabolite Levels in BioBreeding Rats.” The FASEB Journal 27 (4): 1711–20. https://doi.org/10.1096/fj.12-223339.
Wen, Li, and Andrew Duffy. 2017. “Factors Influencing the Gut Microbiota, Inflammation, and Type 2 Diabetes.” The Journal of Nutrition 147 (7): 1468S-1475S. https://doi.org/10.3945/jn.116.2407
Winship, Ian R., Serdar M. Dursun, Glen B. Baker, Priscila A. Balista, Ludmyla Kandratavicius, Joao Paulo Maia-de-Oliveira, Jaime Hallak, and John G. Howland. 2019. “An Overview of Animal Models Related to Schizophrenia.” Canadian Journal of Psychiatry. Revue Canadienne de Psychiatrie 64 (1): 5–17. https://doi.org/10.1177/0706743718773728.
Wonodi, Ikwunga, and Robert Schwarcz. 2010. “Cortical Kynurenine Pathway Metabolism: A Novel Target for Cognitive Enhancement in Schizophrenia.” Schizophrenia Bulletin 36 (2): 211–18. https://doi.org/10.1093/schbul/sbq002.
Yano, Jessica M., Kristie Yu, Gregory P. Donaldson, Gauri G. Shastri, Phoebe Ann, Liang Ma, Cathryn R. Nagler, Rustem F. Ismagilov, Sarkis K. Mazmanian, and Elaine Y. Hsiao. 2015. “Indigenous Bacteria from the Gut Microbiota Regulate Host Serotonin Biosynthesis.” Cell 161 (2): 264–76. https://doi.org/10.1016/j.cell.2015.02.047.
Zheng, P., B. Zeng, C. Zhou, M. Liu, Z. Fang, X. Xu, L. Zeng, et al. 2016. “Gut Microbiome Remodeling Induces Depressive-like Behaviors through a Pathway Mediated by the Host’s Metabolism.” Molecular Psychiatry 21 (6): 786–96. https://doi.org/10.1038/mp.2016.44.
Zheng, Peng, Benhua Zeng, Meiling Liu, Jianjun Chen, Junxi Pan, Yu Han, Yiyun Liu, et al. 2019. “The Gut Microbiome from Patients with Schizophrenia Modulates the Glutamate-Glutamine-GABA Cycle and Schizophrenia-Relevant Behaviors in Mice.” Science Advances 5 (2). https://doi.org/10.1126/sciadv.aau8317.
Zhu, Feng, Ruijin Guo, Wei Wang, Yanmei Ju, Qi Wang, Qingyan Ma, Qiang Sun, et al. 2019. “Transplantation of Microbiota from DrugFree Patients with Schizophrenia Causes Schizophrenia-like Abnormal Behaviors and Dysregulated Kynurenine Metabolism in Mice.” Molecular Psychiatry, August, 1–14. https://doi.org/10.1038/s41380-019-0475-4