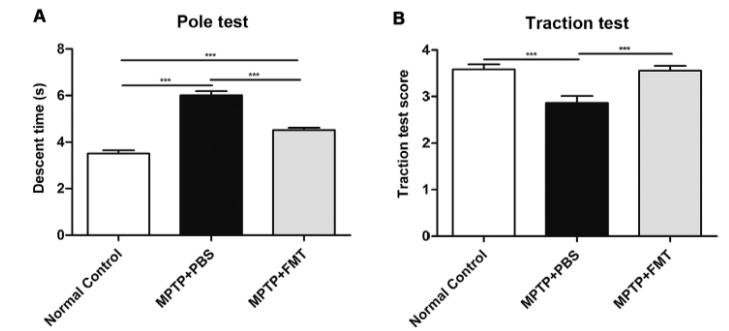
15 minute read
Fecal microbiota transplantation as a defence against MPTP induced Parkinson’s disease mice
Fecal microbiota transplantation as a defence against MPTPinduced Parkinson’s disease mice
Farzad Ahmadi
Advertisement
Parkinson’s disease (PD) patients show consistent dysbiosis of the gut microbiota providing evidence supporting a gut-brain axis. However, the mechanisms by which the gut microbiome influences the pathogenesis of PD is still unknown. Further, current therapies only treat symptoms, and none stop or slow the progression of PD due to a limited understanding of PD’s major molecular markers. The paper by Sun et. Al. (2018) investigates the involvement of the gut microbiota in PD progression and the neuroprotective effects of fecal microbiota transplantation (FMT) within PD mice. FMT is an effective method whereby the feces of one murine is infused into the GI tract of another, essentially causing a complete intervention of the microbial. FMT from α-Syn overexpressing mice into germ-free mice induced motor dysfunction and reduced neurotransmitter release within the germ-free mice showing the ability of the microbiome to induce PD. To test the neuroprotective effects of FMT, the gut microbiota from phosphate-buffered saline (PBS) treated mice were infused into MPTPinduced PD mice. FMT reduced microbiota dysbiosis, increased serotonin and dopamine release within the striatum, decreased fecal short-chain fatty acids (SCFAs) and rescued motor impairments within the PD mice. Microglia and astrocyte activation were also reduced within the substantia nigra showing a decrease in neuroinflammatory precursors. Since gut microbial dysbiosis shows consistent involvement in PD, future studies should aim at understanding the mechanism underlying this association and other neurological disorders, and targeting the microbiota as a possibly therapy for PD.
Background and Introduction
Parkinson’s disease (PD) is a very common neurodegenerative disorder resulting primarily from the loss of dopaminergic neurons in the substantia nigra (Dauer and Przedborski 2003). The risk of PD increases with age affecting approximately 1% of people over 60 (Jackson et al. 2019). The misfolding and aggregation of the protein, alpha-synuclein (α-Syn), into Lewy Bodies within these dopaminergic neurons along with mitochondrial dysfunction is theorized to cause apoptosis through a variety of mechanisms (Haikal, Chen, and Li 2019; Dauer and Przedborski 2003). The characteristic motor symptoms such as bradykinesia and resting tremor are commonly preceded by dysfunction of the GI system such as constipation and weight loss, provoking researchers to investigate the gut-microbiota-brain axis (Goldstein, Sewell, and Sharabi 2011). PD has been established as a multifactorial disorder since studies show that the monogenic mutations account, only, for approximately 5-10% of PD cases leaving the remainder to be caused by complex combination between genetic and environmental factors (Lill 2016).The first autosomal dominant mutation to cause PD was discovered to be in the SNCA gene responsible for the production and expression of α-Syn, however advances have been made and many mutations have been identified (Lill 2016). Many studies have found evidence supporting the involvement of the gut microbiome in neuronal function and disease (Unger et al. 2016; Jackson et al. 2019). Furthermore, a high-fiber Mediterranean diet has been shown to decrease PD risk as a result of changes in the concertration of relative short-chain-fatty acid (SCFA) producing and lipopolysaccharide (LPS) containing bacteria as opposed to a high-fat, high-sugar Western diet (Jackson et al. 2019; Cani et al. 2008). This delicate balance within the gut is theorized to contribute to PD pathogenesis through the complex innervations between the vagus nerve, the enteric nervous system (ENS) and the GI tract (Braak et al. 2003). It has been proposed that a substance with prion-like properties, possibly αSyn, that moves from the GI tract to the CNS through these pathways and induce cell death (Vendrik et al. 2020). Recent studies have shown that chronic stress is a major contributor to the progression of PD through the dysfunction of the intestinal barrier leading to hyper-permeability and pro-inflammatory environment due to a decrease in anti-inflammatory bacteria (Lactobacillus) within the microbiota (Dodiya et al. 2020; Forsyth et al. 2011). Healthy intestinal epithelial cells produce low levels of TLR4; thereby, hyperpermeability will induce the production of pro-inflammatory substances such as TNF-α through increases in TLR4 which will not only cause peripheral immune activation leading to gut inflammation but can cross the blood brain barrier to cause neuroinflammation via microglia activation (Qin et al. 2007; Ransohoff 2016). Fecal microbiota transplantation (FMT) is an effective technique used to transplant the fecal matter from a healthy donor to the GI tract of a patient. FMT is investigated as a treatment for a wide variety of disorders including neurological diseases such as PD, autism, multiple sclerosis and many others (Vendrik et al. 2020). These innovative discoveries show that the dysbiosis of flammation of the intestinal epithelial cells are key factors in the pathogenesis of PD which led Dr. Sun’s group to investigate the neuroprotective effects of FMT.
Major Results
A group of 8-week-old wild-type C57BL/6 mice were exposed to MPTP injections for 5 consecutive days to induce PD-like symptoms while the other group served as a control. The MPTPinduced PD mice were then introduced to FMT, from a healthy donor (wild-type mice), for 7 consecutive days via gavage or were given phosphate-buffered-saline (PBS) for 7 consecutive days to act as the PD control for FMT. FMT alleviates motor impairments in PD mice Physical impairments such as bradykinesia, postural defects, and resting tremor are hallmarks of PD. These impairments were measured through a pole test, measuring the time it takes to descent from the tip of the pole to the base, and a traction test, scoring the mice based on their ability to attach onto the platform with their hind limbs. The MPTP + PBS mice showed significant motor dysfunction such as longer descent times and an inability to use their hind limbs to attach resembling a lower traction score. Remarkably, the MPTP + FMT mice showed a significant increase in motor function such as decreases in descent time and higher traction scores. This data provides evidence supporting FMT as a viable method to rescue motor impairments in PD mice. Figure 1. FMT improves motor function within PD mice. A) The
MPTP-induced PD mice which have undergone FMT resemble the normal controls and have significantly lower descent times. B) The MPTP + FMT mice show significantly higher traction scores like those of the normal control group (Sun et al. 2018) Rescued dopamine and serotonin in PD mice In order to examine the neuroprotective effects of FMT within the brain, the concentration of neurotransmitters present along with their metabolites were examined within the striatum. Fluorescence detection was used after high performance liquid chrothe gut microbiome along with the hyper-permeability and in
matography was performed. FMT rescued neurotransmitter release within the striatum as observed by their relative concentrations within figure 2. This shows that FMT not only alleviates the symptoms of PD but slows and influences the progression of PD by rescuing the neurotransmitter levels that are normally decreased within the PD brains.
Figure 3. The microbiome is responsible for the concentrations of SCFAs present and in turn these SCFAs can activate microglia and induce neuroinflammation and pathology. Figure 4. The relative number of activated microglia and astrocytes within the substantia nigra (SN) are reduced significantly by FMT treatment as opposed to
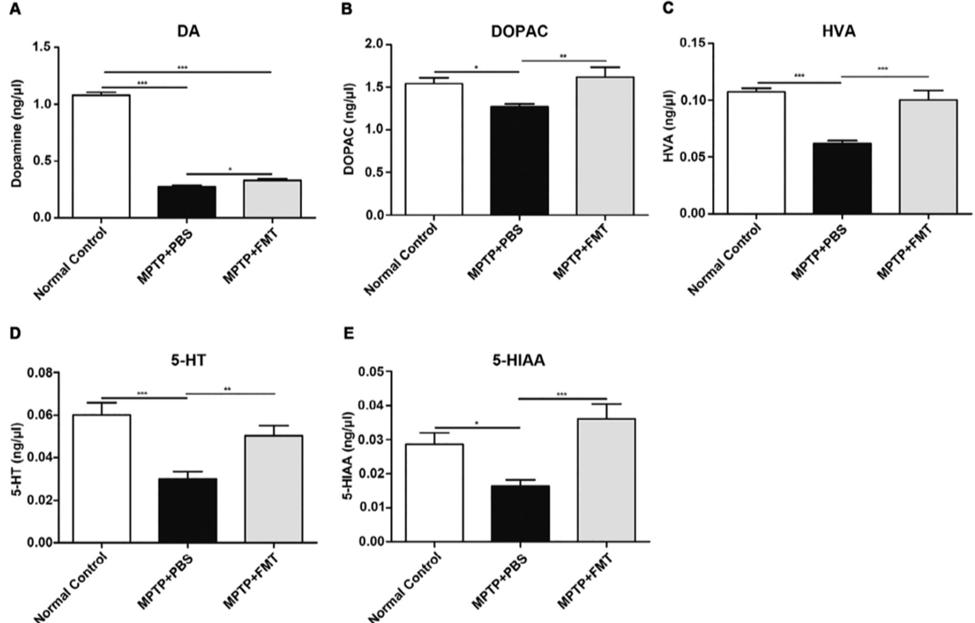
Figure 2. FMT rescues the loss of striatal neurotransmitters mediated by MPTP. An overall increase in neurotransmitter and metabolite levels are measured within the MPTP+FMT group
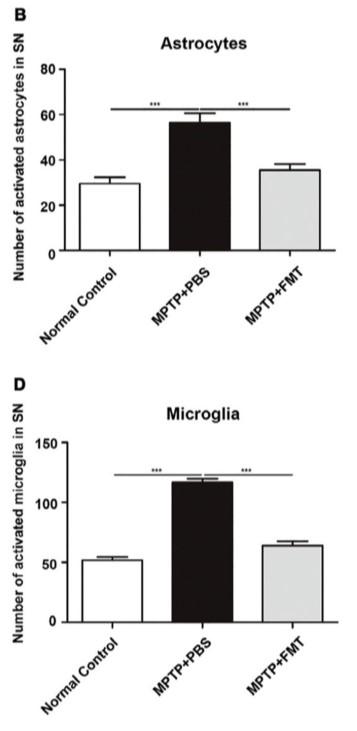
through fluorescence detection. (Sun et al. 2018) MPTP+PBS mice. (Sun et al. 2018)
FMT reduces microglial activation, neuroinflammation, and restore normal SCFAs MPTP induced PD causes dysbiosis of the microbiome resulting in alterations in the concentrations of SCFAs responsible for increased activation of microglia and inflammation. FMT is shown to decrease all SCFAs (acetic acid, propionic acid, butyric acid, and n-valeric acid) concentrations as opposed to MPTP+PBS group resembling the control group. Further, IF staining was used to examine the interactions between microglia, astrocytes and dopaminergic neurons. FMT significantly reduced the activation of astrocytes and microglia within the substantia nigra. This data suggests that through a restoration of normal SCFA concentrations, microglia and astrocyte activation were significantly reduced and thereby, so was the neuroinflammation
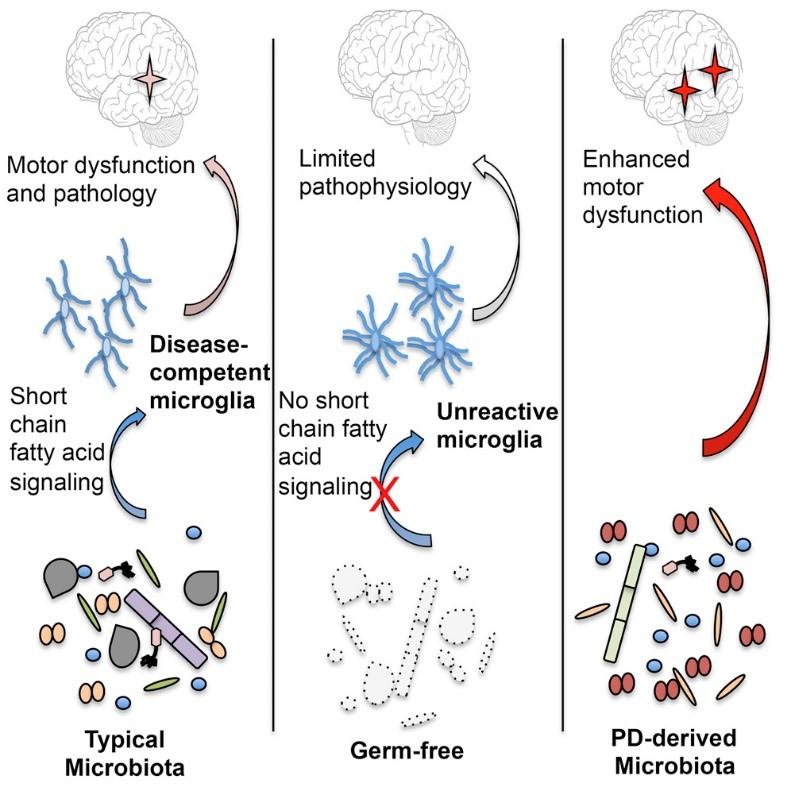
Discussions
This paper investigates the neuroprotective effects of FMT on
MPTP-induced PD mice. They have shown that FMT rescues striatal neurotransmitter levels and restores dopaminergic neurons within the substantia nigra. Furthermore, they have shown that FMT increases motor function and improves bradykinesia, muscle weakness, and postural instability. FMT reduces gut microbial dysbiosis and restores SCFA concentrations which contribute to reduced activation of microglia and astrocytes resulting in less neuroinflammation. The authors shown that the gut microbial dysbiosis within PD mice is consistent with dysbiosis observed in human PD patients suggesting that this
PD model can be used in further research to investigate a possible therapy and provide improved understanding of PD pathology.
These results are relevant as they provide evidence for the neuroprotective effects of a healthy gut microbiome and FMT in PD mice. They build on evidence provided previously about the gut -brain axis and the influence of the gut microbiome on neurological health and disease (Sampson et al. 2016; Haikal, Chen, and Li 2019; Hill‐Burns et al. 2017; Unger et al. 2016). It has been known that there is bidirectional communication between the CNS and the gut microbiome through the vagus nerve and
ENS. Interestingly, this study shows that FMT can reverse, not only the symptoms of PD, but restore the neurotransmitter levels within the striatum. Furthermore, since GI symptoms precede other symptoms, it is suggested that the pathogen that eventually causes PD moves into the GI tract first then into the
CNS (Dauer and Przedborski 2003). This pathogen alongside 15
v crobial dysbiosis. of both controls as TLR4 is not present to initiate the TLR4/TNF
other possible factors such as chronic stress, promote a hyperpermeability of the intestinal epithelial barrier and promote a pro-inflammatory environment. The authors suggest this is due to the TLR4/TNF-α pathway as the damaged epithelial cells increase TLR4 activation which causes neuroinflammation through upregulation of other inflammatory factors such as TNF- α and cytokine secretion. They showed that FMT decreased the expression of this pathway and thereby, reduced gut and neuroinflammation. However, the authors acknowledge that this is only a possibility and there are many other pathways by which signalling can occur. Essentially, the gut microbiome is a very complex system that has many implications on the CNS and neurological disorders. The ability of FMT to rescue almost all PD symptoms and restore near normal brain status is remarkable and must be further studied.
Critical Analysis
This paper provides a strong foundation for future studies examining the role of the gut microbiota in neurological diseases. Since this study, along with many others, have shown that microbial dysbiosis leads to neuroinflammation and neurological disorders, future studies should investigate the methods by which the microbial balance becomes disturbed and the effects of diet and other environmental factors on this. The known genetic mutations only account for 10% of the PD cases therefore there must be a multitude of environmental factors that must account for the other 90% (Lill 2016). Therefore, future studies must investigate the effects of diet, stress, environmental conditions, viral effects as causes of microbial dysbiosis and PD pathology. Also, future studies must investigate more accurate molecular markers that can be used in tandem with microbial dysbiosis as an effective and accurate diagnostic tool for PD. The experiments and controls used in this study are well established. The neurotoxin MPTP is used to induce PD and they included controls to test for the possible effects of PBS. They found consistent evidence with other papers regarding the effects of the gut microbiome on PD (Dauer and Przedborski 2003; Sampson et al. 2016). There have been no contradictory studies published showing negative neurological effects following FMT in PD mice. Therefore, there is a well-established link between the gut microbiome and the brain and dysbiosis and neurological disorders. However, the mechanisms by which microbial dysbiosis influences PD pathology remains unclear and must be investigated further. More research must be conducted on the reasons why dysbiosis occurs (e.g. external pathogen, stress, diet, etc.) and how this dysbiosis contributes to αSyn aggregation within the CNS. The TLR4/TNF-α pathway proposed by the authors based on previous papers and this study, must be further investigated to examine if it has a direct role in PD pathogenesis. Interestingly, dysbiosis of the gut microbiome and alterations of SCFA concentrations are subjective as many papers provide a variety of findings, and this makes the search
Future Directions
A future study should investigate the TLR4/TNF- α pathway as the primary pathway regulating neuroinflammation and microglial activation. This pathway consists of an overexpression of TLR4 which through a descending cascade of protein activation induces TNF- α activation which leads to cytokine release and inflammation via transcriptional activators. This study provided evidence that FMT reduces gut and neuroinflammation possibly through a reduction of this pathway, but the evidence is unclear. The TLR4 are commonly found in low concentrations on intestinal epithelial cells. Therefore, an artificial method of causing damage to the epithelial cells (e.g. MPTP) must be used in tandem with a TLR4-knock out (KO) mice strain to observe if the TLR4/TNF- α pathway is the main mechanism responsible for the neuroinflammation and PD pathogenesis following miThe researcher must include two groups, one consisting of TLR4 -KO mice and the other must be wild-type C57BL/6 mice. Each of the mice strains must be divided into two groups and one will undergo i.p. injection of MPTP for 5 consecutive days in order to produce MPTP-induced PD mice. After the injection has occurred, behavioural tests such as the pole descent test and traction test must be done followed by tests measuring for neuroinflammatory molecular markers such as TNF- α, Iba-1 (microglial marker), and GFAP (astrocyte marker). If this pathway plays an important role in mediating neuroinflammation and PD pathogenesis, the following results should be expected. The TLR4-KO + MPTP mice must show short descent times and high traction scores similar to the TLR4-KO and the wild-type C57BL/6 mice. The only mice strain showing PD-like bradykinesia and movement impairment should be the C57BL/6 mice. Furthermore, once testing for neuroinflammatory markers, the levels within the TLR4-KO + MPTP mice must be similar to those for possibly therapies and mechanisms more difficult.
α pathway and cause neuroinflammation. However, there should be gut inflammation present and hyper-permeability of the intestinal epithelial cells possibly through sigmoidoscopy. Furthermore, if this pathway is not the main pathway responsible for the neuroinflammation following microbial dysbiosis, then the TLR4-KO + MPTP mice test results should mimic those of the C56BL/6 + MPTP mice as TLR4 would have no significant effects on PD pathogenesis. If this pathway is determined to be the major pathway contributing to neuroinflammation and PD pathogenesis, then further studies must be conducted investigating possible methods of suppressing the pathway and therapies targeting this pathway.
10.
11.
12.
13.
14.
15.
16. Braak, H., U. Rüb, W. P. Gai, and K. Del Tredici. 2003. “Idiopathic Parkinson’s Disease: Possible Routes by Which Vulnerable Neuronal Types May Be Subject to Neuroinvasion by an Unknown Pathogen.” Journal of Neural Transmission 110 (5): 517–36. https:// doi.org/10.1007/s00702-002-0808-2. Cani, Patrice D., Rodrigo Bibiloni, Claude Knauf, Aurélie Waget, Audrey M. Neyrinck, Nathalie M. Delzenne, and Rémy Burcelin. 2008. “Changes in Gut Microbiota Control Metabolic Endotoxemia-Induced Inflammation in High-Fat Diet–Induced Obesity and Diabetes in Mice.” Diabetes 57 (6): 1470–81. https://doi.org/10.2337/db07-1403. Dauer, William, and Serge Przedborski. 2003. “Parkinson’s Disease: Mechanisms and Models.” Neuron 39 (6): 889–909. https:// doi.org/10.1016/S0896-6273(03)00568-3. Dodiya, Hemraj B., Christopher B. Forsyth, Robin M. Voigt, Phillip A. Engen, Jinal Patel, Maliha Shaikh, Stefan J. Green, et al. 2020. “Chronic Stress-Induced Gut Dysfunction Exacerbates Parkinson’s Disease Phenotype and Pathology in a Rotenone-Induced Mouse Model of Parkinson’s Disease.” Neurobiology of Disease 135 (Complete). https://doi.org/10.1016/j.nbd.2018.12.012. Forsyth, Christopher B., Kathleen M. Shannon, Jeffrey H. Kordower, Robin M. Voigt, Maliha Shaikh, Jean A. Jaglin, Jacob D. Estes, Hemraj B. Dodiya, and Ali Keshavarzian. 2011. “Increased Intestinal Permeability Correlates with Sigmoid Mucosa Alpha-Synuclein Staining and Endotoxin Exposure Markers in Early Parkinson’s Disease.” PLOS ONE 6 (12): e28032. https://doi.org/10.1371/journal.pone.0028032. Goldstein, David S., LaToya Sewell, and Yehonatan Sharabi. 2011. “Autonomic Dysfunction in PD: A Window to Early Detection?” Journal of the Neurological Sciences, Seventh Congress of Mental and other Non-motor Dysfunctions in Parkinson’s Disease, 310 (1): 118–22. https://doi.org/10.1016/j.jns.2011.04.011. Haikal, Caroline, Qian-Qian Chen, and Jia-Yi Li. 2019. “Microbiome Changes: An Indicator of Parkinson’s Disease?” Translational Neurodegeneration 8. https://doi.org/10.1186/s40035-019-0175-7. Hill‐Burns, Erin M., Justine W. Debelius, James T. Morton, William T. Wissemann, Matthew R. Lewis, Zachary D. Wallen, Shyamal D. Peddada, et al. 2017. “Parkinson’s Disease and Parkinson’s Disease Medications Have Distinct Signatures of the Gut Microbiome.” Movement Disorders 32 (5): 739–49. https://doi.org/10.1002/mds.26942. Jackson, Aeja, Christopher B. Forsyth, Maliha Shaikh, Robin M. Voigt, Phillip A. Engen, Vivian Ramirez, and Ali Keshavarzian. 2019. “Diet in Parkinson’s Disease: Critical Role for the Microbiome.” Frontiers in Neurology 10. https://doi.org/10.3389/fneur.2019.01245. Lill, Christina M. 2016. “Genetics of Parkinson’s Disease.” Molecular and Cellular Probes, Genetics of multifactorial diseases, 30 (6): 386–96. https://doi.org/10.1016/j.mcp.2016.11.001. Qin, Liya, Xuefei Wu, Michelle L. Block, Yuxin Liu, George R. Breese, Jau-Shyong Hong, Darin J. Knapp, and Fulton T. Crews. 2007. “Systemic LPS Causes Chronic Neuroinflammation and Progressive Neurodegeneration.” Glia 55 (5): 453–62. https://doi.org/10.1002/glia.20467. Ransohoff, Richard M. 2016. “How Neuroinflammation Contributes to Neurodegeneration.” Science 353 (6301): 777–83. https:// doi.org/10.1126/science.aag2590. Sampson, Timothy R., Justine W. Debelius, Taren Thron, Stefan Janssen, Gauri G. Shastri, Zehra Esra Ilhan, Collin Challis, et al. 2016. “Gut Microbiota Regulate Motor Deficits and Neuroinflammation in a Model of Parkinson’s Disease.” Cell 167 (6): 1469-1480.e12. https:// doi.org/10.1016/j.cell.2016.11.018. Sun, Meng-Fei, Ying-Li Zhu, Zhi-Lan Zhou, Xue-Bing Jia, Yi-Da Xu, Qin Yang, Chun Cui, and Yan-Qin Shen. 2018. “Neuroprotective Effects of Fecal Microbiota Transplantation on MPTP-Induced Parkinson’s Disease Mice: Gut Microbiota, Glial Reaction and TLR4/TNF-α Signaling Pathway.” Brain, Behavior, and Immunity 70 (May): 48–60. https://doi.org/10.1016/j.bbi.2018.02.005. Unger, Marcus M., Jörg Spiegel, Klaus-Ulrich Dillmann, David Grundmann, Hannah Philippeit, Jan Bürmann, Klaus Faßbender, Andreas Schwiertz, and Karl-Herbert Schäfer. 2016. “Short Chain Fatty Acids and Gut Microbiota Differ between Patients with Parkinson’s Disease and Age-Matched Controls.” Parkinsonism & Related Disorders 32 (November): 66–72. https://doi.org/10.1016/j.parkreldis.2016.08.019. Vendrik, Karuna E. W., Rogier E. Ooijevaar, Pieter R. C. de Jong, Jon D. Laman, Bob W. van Oosten, Jacobus J. van Hilten, Quinten R. Ducarmon, Josbert J. Keller, Eduard J. Kuijper, and Maria Fiorella Contarino. 2020. “Fecal Microbiota Transplantation in Neurological Disorders.” Frontiers in Cellular and Infection Microbiology 10. https://doi.org/10.3389/fcimb.2020.00098.