
19 minute read
Effect of germ free mice on monoamine neurotransmitter gene expression and anxiety like behavior
Effect of germ-free mice on monoamine neurotransmitter gene expression and anxiety-like behavior; further evidence of the gut-brain connection.
Alexander Geiger
Advertisement
Studies have demonstrated gut microbiota are important for cognitive health, as revealed when gut microbiome balance is disrupted. Neurological, behavioral, and mental health issues can result. Questions arise as to what specific effects an abnormal microbiome have on the brain and, importantly, whether those effects can be reversed. The current study, represented by the visual abstract below, examined hippocampal monoamine neurotransmitter gene expression in mice with absent gut microbiota, and observed behavioral aspects, compared to mice with more normal gut microbiota (Pan et al. 2019). The researchers chose monoamine neurotransmitters as a promising biological indicator due to several previous studies linking this system to neuropsychiatric disorders. They compared Germ Free (GF) mice to Specific-Pathogen-Free (SPF) mice and found that 19 monoamine genes were expressed at different levels in GF mice. Additionally, GF mice portrayed much less anxiety than SPF mice, as measured using the open field test (OFT) and the novelty suppressed feeding test (NSF). The GF mice displayed higher locomotor activity and reduced latency for feeding compared to SPF mice. Finally, the researchers attempted to re-colonize gut microbiota in a set of GF mice (CGF) to reverse the effects of differential gene expression. However, CGF mice continued to show less anxiety compared with SPF mice, and only 50% of the monoamine neurotransmitter genes were successfully expressed to the same levels as SPF mice. Keywords: gut microbiota, gut-brain axis, monoamine neurotransmitters, GF/SPF mice, hippocampus
Background and Introduction
Studies on gut microbiota and their connection to the brain include such areas as mental illness (Misiak et al. 2020), obesity and inflammation (Soto et al. 2018), and neural-related behavior (Huo et al. 2017). Understanding the neurological and
Monoamine Gene Expression
Hippocampal slices were analyzed, and bioinformatics software was used to research genes. Overall, 19 genes in the GF mice were found to be up- or down-regulated differently when compared to the SPF group’s monoamine levels. Upregulated genes with significant differences included 15 genes as seen in Figure 1. The genes that were downregulated included: Fos, Grk6, and Nr4a1. Bioinformatics analysis revealed the most categorized into four types as seen in Figure 1. These included
behavioral impact of biological processes is a key goal, making these studies truly relevant to neuroscience. The gut microbiome can affect the brain commensally, but also may be the culprit behind neurological disorders. Misiak et al. (2020) have accumulated a body of evidence from studies that link dysregulation of the hypothalamic-pituitary-adrenal axis (HPA), stemming from abnormal gut microbiota, to mood disorders and more severe mental illness. Additionally, the gut microbiota and HPA were linked to anxiety and stress disorders (Frankiensztajn, 2020). The reciprocal path has also been studied related to how neuronal systems affect the gut. In one study, it was shown that altered serotonin levels affected gut microbes which in turn can affect homeostasis, leading to many symptoms including depression (Reigstad, 2015). As complex & metabolism genes, where no CGF genes successfully re
connections are discovered, there remain questions about the impact gut microbes have on specific areas within the brain what accounts for differences in their behavioral and cognitive health effects. An additional important question is whether the effects can be reversed. In the current study, Pan et al. (2019) were able to expand upon the gut-brain link and directly correlate hippocampal monoamine neurotransmitter gene expression levels with a lack of a microbiota. They included a neuro-behavioral link by measuring anxiety-like behavior as well. Briefly, the method and materials involved three types of mice split into three groups each. All were tested at around 9 weeks, which kept in strictly controlled, sterile environments with sterilized water and food. SPF mice were purchased and kept in the same feeding conditions. CGF mice were created from adolescent male GF mice. The recolonization procedure was accomplished by placing them with SPF bedding and fecal matter. Each of the groups were randomly assigned to one of three separate tests. One group underwent behavioral activity testing in the OFT and NSF tests in a quiet environment, another group was used for western blotting and the third underwent PCR array analysis. This was done in separate groups so that the behavioral testing itself would not affect gene expression testing.
probable function and pathways involving the genes and were dopamine and serotonin receptor, signal transduction, transporters and metabolism and downstream signaling genes. The most effected genes were related to dopaminergic and serotonergic synapses.
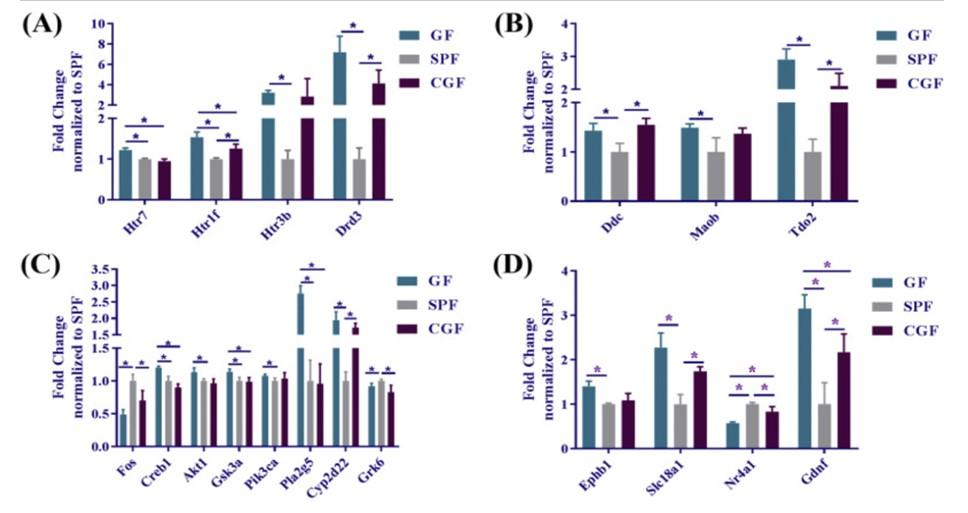
Figure 1. Gene expression results of Pan et al. study (2019). Represents the levels of hippocampal monoamine neurotransmitter genes across the three groups of mice. Graph (A) represents dopamine and serotonin receptor genes. Recolonization was successful only in Htr7 in this category, (B) is transporters were considered to be adults. GF mice were generated and
colonized; Graph (C) represents the monoamine neurotransmitters related to signal transduction. Several genes were expressed to the same level as SPF. Graph (D) represents the downstream signaling gene targets.
Behavioral Test Results
Locomotor activity and latency in feeding are two measures of anxiety in mice studies. Groups of mice; GF (n=22), and SPF (n=25) were given standard anxiety-index tests in quiet, low stress environment. OFT measures the movement and provides the total distance traveled, as well as time spent in the center cage. For the NSF test, mice were unfed for 24 hours to induce hunger and placed in an open-field novel arena with a single pellet of food. The time it took to leave the edges and eat the pellet was measured. It was found that the GF mice were less anxious, as indicated by more locomotor activity and reduced latency in feeding compared to the SPF mice. These results coincide with another study that compared GF and SPF motor activity under non-stressed and stressed conditions; the
CGF Results
In experiments designed to examine reversibility of GF effects, adolescent GF mice were recolonized (CGF mice) by placing them with SPF fecal matter. Ten of the 19 genes were expressed at the same level as SPF mice. The OFT and NSF tests were administered, and results showed that the CGF mice retained lower anxiety-like behaviors compared to SPF mice, simirate of noradrenaline, dopamine, or serotonin, but did find differential gene expression in the hippocampus consistent
lar to levels of GF mice, as can be seen in Figure 2. These results seem to conflict with a study by Soto et al. (2017), where expressions were able to be visualized and correlated with behavioral effects. This is a complex connection and important area to study given all the potential detrimental effects on mental and general health. Additionally, by looking at behavioral impact and utilizing bioinformatics, a great deal of information was gathered that can be used in future studies. Results showed that anxiety-like behaviors were reduced in both CGF and GF mice, but in CGF mice, the NSF test showed al results. The authors could add the additional condition of
effects associated with changes in BDNF and GABA related to high fat diet mice were successfully transferred to GF mice, however, they used fecal transplant as a method to transfer gut microbiota.
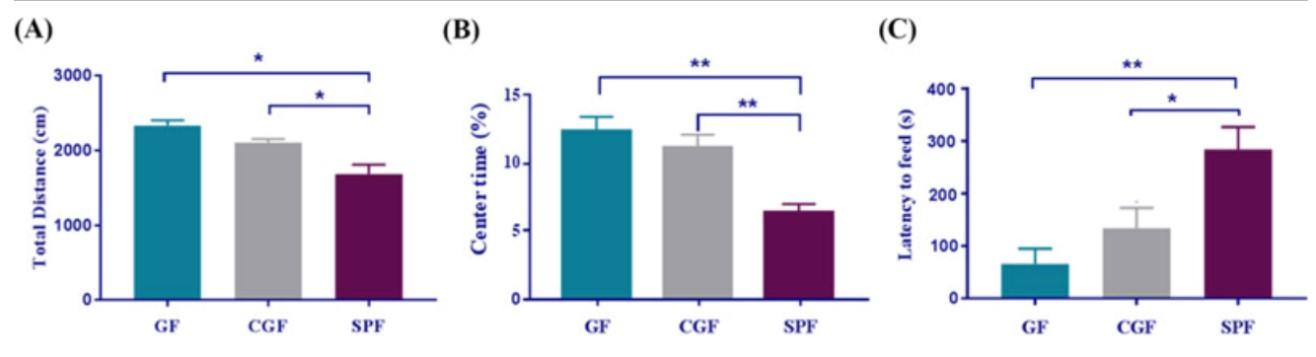
Figure 2: This figure represents results from the behavioral tests for anxiety. Graph (A) and (B) show the results of OFT, distance, and time spent in the center, respectively. Graph C represents the latency to feed time during the NSF test. CGF mice did not achieve SPF anxiety-like results.
Conclusion/Discussions Section
Pan et al. (2019) concluded with the two main findings. First, a lack of gut microbiota did distinctly affect the dopaminergic and serotoninergic pathways in the hippocampi of mice as evidenced by differential gene expression. Additionally, the absence of a microbiome resulted in behavioral changes, as were indicated by less anxiety-like activities of GF mice. The researchers were not able to reverse these effects in adult mice but pointed out that at least 50% of genes were brought to normalized levels using their method of recolonization. The study performed is important because it adds expanded evidence that the gut microbiota effect the brain and provided new insight on specific hippocampal impact of altered Fecal transplant is an example alternate method.
gut microbiome. The authors combined the behavioral aspect by including some standard anxiety tests that allowed them to show an association between the affected hippocampal monoamine neurotransmitter system and behavior. They concluded that their results suggest the absence of early life microbiota can disrupt the entire monoamine neurotransmitter system, which can cause neuropsychiatric effects. ments, one of which was by Diaz Heijtz et al. (2011). These researchers looked at neurochemistry changes in the hippocampus, frontal cortex, and striatum in GF and SPF mice as well as gene expression in five brain regions including the hippocampus. They found similar anxiety-like behavioral effects in GF mice, no significant difference in the hippocampus turnover with the current study. The current study expanded upon the previous one by looking at the reversibility of these differences. In another study that looked at the bidirectional impact on intestinal microbes, hormonal levels and behavior in stressed and un-stressed GF and SPF mice, they found similar results in behavioral tests (Huo et al. 2017). Pan et al. (2019) expanded upon this study by looking at the hippocampal connection.
Critical Analysis
The methodologies utilized by Pan et al. (2019) allowed the researchers to target and analyze specific gene activity in the hippocampus. Results of varied monoamine gene Though in the current study they found that the recolonization procedure did not result in fully reversing the effects of GF, attempting to understand additional aspects helps to advance research in this area. Improvement areas lie first in the behavioral analysis. increased latency in feeding over GF. The authors should perform an additional behavioral test such as dark-light or elevated zero-maze testing that could be used to further validate that differences between mice groups are related to anxiety. Additionally, in this experiment, behavioral tests were conducted in a quiet, stress free environment. The authors concluded that the GF mice were less anxious, but this was found to be not significant when GF and SPF mice were put in stress-induced environments (Geng et al. 2020). Stress situations activate the HPA axis which may negate differences in GF and SPF behaviorcontrolled stress to further determine if GF mice are less anxious.
There are some additional experiments that the authors discuss should be completed. The bioinformatics analysis revealed expected pathways and effects. Metabolomic studies need to be completed to validate those pathways are correct. One other factor the authors could review, as mentioned, is the method used to recolonize mice, as it might be insufficient to conclude that the effects of GF are not reversible in adult mice. The results of this study supported earlier experi
Future Directions Section
This study still leaves some unanswered questions, such as how the absence of gut microbiota changes the brain and whether a specific developmental stage of life most adversely
effects the ability to colonize gut microbiomes. Pan et al. (2019) studied a single age-group, adult mice, but it has been shown that in humans, microbiota composition changes in stages from birth until a shift to a more adult-like colonization at about 3-5 years (Koenig, 2010). There may be a more susceptible period to recolonization (Rodriguez, 2015). The future experiment should expand to multiple ages and stages of development to determine whether recolonization would be successful at alternate ages and, if so, whether there is an optimal time to reverse the effects of altered microbiota. The study would include GF, CGF, and SPF mice, each with a neonatal group, breast-fed group, and weaning stage group. PCR array analysis and western blotting could be used for analyzing hippocampal gene expression levels along with metabolomic studies as suggested by the authors to validate pathways predicted based on bioinformatics programs. Additionally, different behavioral measures would need to be administered to better suit the age of the mice, such as in vocalization and movement comparisons. Expected results should show that the effects are reversible at all the earlier stages. Therefore, recolonizing at such a young age should result in normalized behaviors and gene expression levels. The importance of knowing developmental stage-related impact would be beneficial if eventually would lead to testing, intervention, and prevention of neuropsychological effects of abnormal gut microbiomes.
1. 2.
3. 4.
5. 6.
7. 8.
9. 10.
11. 12.
13. 14.
15. 16.
17. 18.
19. Diaz Heijtz, Rochellys, Shugui Wang, Farhana Anuar, Yu Qian, Britta Björkholm, Annika Samuelsson, Martin L. Hibberd, Hans Forssberg, and Sven Pettersson. 2011. “Normal Gut Microbiota Modulates Brain Development and Behavior.” Proceedings of the National Academy of Sciences of the United States of America 108 (7): 3047–52. https://doi.org/10.1073/pnas.1010529108. Frankiensztajn, Linoy Mia, Evan Elliott, and Omry Koren. “The Microbiota and the Hypothalamus-Pituitary-Adrenocortical (HPA) Axis, Implications for Anxiety and Stress Disorders.” Current Opinion in Neurobiology 62 (June 2020): 76–82. https://doi.org/10.1016/j.conb.2019.12.003. Geng, Shaohui, Liping Yang, Feng Cheng, Zhumou Zhang, Jiangbo Li, Wenbo Liu, Yujie Li, et al. 2020. “Gut Microbiota Are Associated With Psychological Stress-Induced Defections in Intestinal and Blood–Brain Barriers.” Frontiers in Microbiology 10 (January): 3067. https://doi.org/10.3389/fmicb.2019.03067. Huo, Ran, Benhua Zeng, Li Zeng, Ke Cheng, Bo Li, Yuanyuan Luo, Haiyang Wang, et al. 2017. “Microbiota Modulate Anxiety-Like Behavior and Endocrine Abnormalities in Hypothalamic-Pituitary-Adrenal Axis.” Frontiers in Cellular and Infection Microbiology 7 (November): 489. https://doi.org/10.3389/fcimb.2017.00489. Koenig, Jeremy E., Aymé Spor, Nicholas Scalfone, Ashwana D. Fricker, Jesse Stombaugh, Rob Knight, Largus T. Angenent, and Ruth E. Ley. “Succession of Microbial Consortia in the Developing Infant Gut Microbiome.” Proceedings of the National Academy of Sciences of the United States of America 108 Suppl 1 (March 15, 2011): 4578–85. https://doi.org/10.1073/pnas.1000081107 Misiak, Błażej, Igor Łoniewski, Wojciech Marlicz, Dorota Frydecka, Agata Szulc, Leszek Rudzki, and Jerzy Samochowiec. “The HPA Axis Dysregulation in Severe Mental Illness: Can We Shift the Blame to Gut Microbiota?” Progress in Neuro-Psychopharmacology and Biological Psychiatry 102 (August 2020): 109951. https:// doi.org/10.1016/j.pnpbp.2020.109951. Pan, Jun-Xi, Feng-Li Deng, Ben-Hua Zeng, Peng Zheng, Wei-Wei Liang, Bang-Min Yin, Jing Wu, et al. “Absence of Gut Microbiota during Early Life Affects Anxiolytic Behaviors and Monoamine Neurotransmitters System in the Hippocampal of Mice.” Journal of the Neurological Sciences 400 (May 2019): 160–68. https:// doi.org/10.1016/j.jns.2019.03.027. Reigstad, Christopher S., Charles E. Salmonson, John F. Rainey Iii, Joseph H. Szurszewski, David R. Linden, Justin L. Sonnenburg, Gianrico Farrugia, and Purna C. Kashyap. “Gut Microbes Promote Colonic Serotonin Production through an Effect of Short‐chain Fatty Acids on Enterochromaffin Cells.” The FASEB Journal 29, no. 4 (April 2015): 1395–1403. https://doi.org/10.1096/fj.14-259598. Rodríguez, Juan Miguel, Kiera Murphy, Catherine Stanton, R. Paul Ross, Olivia I. Kober, Nathalie Juge, Ekaterina Avershina, et al. “The Composition of the Gut Microbiota throughout Life, with an Emphasis on Early Life.” Microbial Ecology in Health and Disease 26 (2015): 26050. https://doi.org/10.3402/mehd.v26.26050. Soto, M., Herzog, C., Pacheco, J.A. et al. Gut microbiota modulate neurobehavior through changes in brain insulin sensitivity and metabolism. Mol Psychiatry 23, 2287–2301 (2018). https://doi.org/10.1038/s41380-018-0086-5
Arianna Gholami
For many years, the public has been aware of the detrimental effects of smoking, but until recently, knowledge about how the main active component of cigarettes, nicotine, affects the brain has remained somewhat elusive. This literature review seeks to explore the results of a paper studying the effects of perinatal nicotine exposure on the genetic profile of VTA dopaminergic neurons and whether it can disrupt various molecular pathways. The authors conducted this study by treating isolated ventral tegmental areas (VTA) of rat pups with either nicotine or saline during the perinatal period of development. They then conducted experiments such as FACS, mRNA & miRNA expression microarrays, and other analysis techniques to gain more information as to the effect that nicotine can have specifically on the dopaminergic neurons of the VTA. It is important to dedicate some time to this topic because it is important to gain new information as to how smoking affects the fetal brain and what effects these are. This will allow the public to become better educated, hopefully leading to a decline in the incidence of smoking, especially in expecting mothers.
Once the authors isolated the VTA of both the nicotine treated and control rat pups, they then sorted the samples of VTA neurons into those that expressed both NeuN and TH. This was done using FACS, to measure the differences in expression of these neurons. When analyzing the results, no statistically significant difference was found compared to control (p>0.5). The dopaminergic neurons were then collected, and a microarray miRNA and mRNA expression analysis was conducted to determine the differential expression profiles of the dopaminergic neurons due to perinatal nicotine exposure. MiRNAs stance abuse within the mesocorticolimbic dopaminergic pathway. This result was validated by a previous study conducted by
(microRNAs) are non-coding, short RNA sequences with the ability to regulate genes in a post-transcriptional manner by targeting the 3’-UTR of other mRNA sequences. In order to elucidate the points of regulation as well as enriched pathways caused by nicotine exposure, the authors used the transcriptome and miRNome of the VTA dopaminergic neurons. When searching for changes in mRNA exposure following perinatal nicotine exposure, it was found that there was a statistically significant difference in differential expression when compared to control, with 862 upregulated and 1,774 down regulated differentially expressed genes (DEGs). These results support the theory that perinatal nicotine exposure is able to cause differential gene expression, and furthermore, that the majority of genes affected are downregulated. In addition to this finding, the authors then conducted an miRNA expression analysis and found that of the 74 differentially expressed miRNAs, 58 were upregulated and 16 were downregulated in response to perinatal nicotine exposure. This is consistent with the data found for mRNA differential expression, due to the fact that the majority of mRNAs were downregulated while the majority of miRNAs were upregulated, and miRNAs are known to regulate mRNA expression. This was validated when using MultimiR, showing that the majority of the DEmiRNAs (differentially expressed miRNAs) that were upregulated were paired with an mRNA DEG that was downregulated.
After gaining information as the differential expression of both mRNAs and miRNAs, the authors investigated the different pathways that were enriched in response to perinatal nicotine exposure. It was found that the downregulation of the DEG mRNAs caused enrichment in pathways such as neuroacnaling, long term potentiation. These pathways are all known to be involved in drug addiction and are known as the Kyoto Encyclopedia Genes and Genomes (KEGG) pathways. In addition to the enrichment of the KEGG pathways, it was also found that additional signaling and synapse pathways were also affected in the same manner. This enrichment was found to be particularly significant in the dopaminergic synapse pathway, a key interest to the authors studying the effects of perinatal nicotine exposure. However, the glutamatergic and serotonergic synapse pathways were less affected than the dopaminergic pathway. Four genes in particular were found to be significantly downregulated in response to perinatal nicotine exposure. The first two genes, Gabrd and Gabrg2, are subunits of the GABA(a) receptor, and have been shown to be involved in the response of dopaminergic neurons and dopaminergic neurotransmission in response to drugs. The second two genes are subunits of the glutamate receptor, Grin2d and Gria3, and are known to be involved in the nucleus accumbens, leading to an increase in dopamine release. These are significant findings because these four genes are involved in modulating the function of the dopaminergic system, leading to a possible vulnerability to drug addiction in individuals exposed to nicotine at a young age.
In addition to the above findings, the authors were able to identify specific miRNAs as well as mRNAs that resulted in a significant alteration within the dopaminergic system. For example, the authors found that there was a reduction in nAChR subunits such as the Beta4 mRNA subunit, Chrnb4, found to be expressed in dopaminergic neurons. This receptor subunit functions in the neuroactive ligand-receptor interaction, and the cholinergic synapse pathways; pathways that were shown to be enriched due to DEG downregulation. Additionally, the authors validated the results of the miRNA and mRNA microarray conducted previously, using RT-qPCR. This validation was conducted using the miRNAs and mRNAs that were deemed significant; Cck and Gabrg2 for the mRNA microarray validation, and Scn1a, Ntrk2, and Ablim3 for the miRNA microarray validation. It was found that the microarray was consistent with previous results from the microarray. This allowed for the researchers to implicate nicotine in drug addiction by showing a correlation between addiction and altered activity of miRNA located in the dopaminergic system, known to be involved in addiction. In terms of nicotine addiction specifically, the authors were able to show that in response to perinatal nicotine exposure, miR-140-3p and miR-140-5p were both differentially expressed. This was a significant finding due to the fact that these two miRNAs have been shown to be directly involved in nicotine addiction by increasing the reinforcing effects of subtive ligand-receptor interactions, calcium signaling, cAMP sig
Bosch et. al., using methamphetamine self-administration rats. In this study, miR-125a-5p in the VTA was shown to play a significant role in the reinforcement of the addictive behaviors displayed by the rats.
As the authors predicted, perinatal nicotine exposure was able to change the differential expression of many miRNAs as well as mRNAs. Additionally, they concluded that this exposure not only led to genetic alterations within these neurons, but also to post-transcriptional gene regulation via genemiRNA target interactions. Given their results, the authors were able to further confirm that these genetic alterations can lead to the reinforcement of addictive behaviors in the mesocorticolimbic pathway of the dopaminergic system. The authors did however recommend further studies into how gender may play a role in how dopaminergic neurons in the VTA are affected by perinatal nicotine exposure, and urged further investigation to help elucidate the effect of miRNAs on other biological pathways. This study is important to acknowledge because it shows a clear link between the effects of nicotine and the brain. Many
people may not take into consideration the lasting consequences that nicotine can have on their brain, and instead only focus on issues such as cancer. However, given this study and studies that have been conducted previously, it has now become clear that more data must be collected to determine how else nicotine may affect our brains.
Keller, Renee F., et al. “Investigating the Genetic Profile of Dopaminergic Neurons in the VTA in Response to Perinatal Nicotine Exposure Using MRNA-MiRNA Analyses.” Scientific Reports, vol. 8, no. 1, 2018, doi:10.1038/ s41598-018-31882-9. Bosch, P J et al. “mRNA and microRNA analysis reveals modulation of biochemical pathways related to addiction in the ventral tegmental area of methamphetamine self-administering rats.” BMC neuroscience vol. 16 43. 19 Jul. 2015, doi:10.1186/s12868-015-0186-y