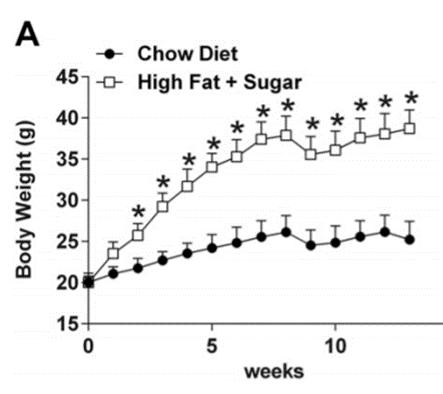
11 minute read
You Are What You Eat : Alzheimer’s and Diabetes Edition. A review of the effect of high fat and sugar diets on cognitive decline
Michelle Ha
Is there a relationship between a high fat diet, insulin resistance within the brain, and cognitive impairment? The aim of a 2017 study sought to determine whether a fatty diet, able to induce insulin sensitivity, has the ability to alter brain signalling pathways impacting cognitive functions. High fat diets have been observed to induce obesity, and obesity is often associated with insulin resistance and dementia. It is likely obesity and insulin resistance have some sort of association since approximately 85% of patients with type 2 diabetes mellitus are also obese (Kang et al., 2016). Furthermore, prior studies have observed that early stage Alzheimer’s patients tend to also show an impairment in insulin signalling (Ferreira et al., 2018).
Advertisement
One of the most prevalent forms of dementia is Alzheimer’s disease, and is characterized by the presence of amyloid plaques and neurofibrillary tangles in the brain. Kang’s study analyzed the effect of insulin induced changes in protein expression relating to cognitive impairment, and whether a high fat diet played a role. It was ultimately concluded that the mice given the high fat and sugar diet showed a statistically significant increase in obesity, lower insulin insulin sensitivity and glucose tolerance, and higher fasting insulin levels compared to the control group. Interestingly, impaired glucose uptake and insulin resistance was also seen in the brain. The researchers concluded that high fat diets cause inflammatory and stress responses which lead to the dysregulation of amyloid-β production and clearance rates, the formation of neurofibrillary tangles, and decreased synaptic plasticity within the brain. Furthermore, insulin resistance is a major pathological feature in patients with Type 2 Diabetes Mellitus, and could also be involved in Alzheimer’s since 80% of patients with Alzheimer’s are also diagnosed with type 2 diabetes, or at the very least, an impaired resting level of glucose (Kothari et al., 2017).
Key words: western diet, obesity, insulin resistance, Alzheimer’s disease.
Background & Introduction:
The global burden of Alzheimer’s is projected to affect over 74.4 million people by 2030, 131.1 million people by 2050, and double every 20 years (Kothari et al., 2017). As such, it’s imperative to fully understand the mechanisms in order to find a cure, or at the very least, decrease the risk of the disease. Evidence suggests that Alzheimer’s is closely linked to metabolic disorders since patients with diabetes are much more likely to develop Alzheimer’s. This risk goes both ways as Alzheimer’s patients also have an increased risk for developing diabetes, and seems to be due to shared mechanisms between metabolic disorders and Alzheimer’s disease (Ferreira et al., 2014). Furthermore, in subjects with and without pre-existing insulin resistance, a diet rich in saturated fats was able to induce systemic insulin resistance (Koska et al., 2016). It has been speculated that Western diets which tend to be higher in saturated fats, may play a role in reducing cognitive function, especially within the hippocampal region (Hansen et al., 2018). Furthermore, animal models chronically exposed to diets high in fats and sugars perform worse in memory tests, suggesting a link to worsening hippocampal memory function. It is likely that systemic changes in the phosphotyrosine signalling are involved in the relationship between fattier diets and cognitive decline. Through tyrosine phosphorylation, changes to enzymes involved in the metabolic pathways are made resulting in the development of insulin resistance, and potentially diabetes (Dittmann et al., 2019). It has also been observed that people who frequently consume high fat or high cholesterol diets often have lipoprotein profiles similar to Alzheimer’s patients. Animal models fed diets high in fat and sugars also show changes in protein markers associated with Alzheimer’s disease (Hansen et al., 2018). Kothari’s team (2017) randomly assigned six week old male C57BL/6NHsd mice into experimental groups. The control group was fed a standard chow diet with 12% of energy coming from lipids. In comparison, the high fat and sugar group was fed a diet where 40% of energy came from lipids. Additionally, while the control group were given pure water to drink, the experimental group had a water source supplemented with 42g of sugar/L. At twelve weeks of age, the mice underwent a glucose tolerance test where blood glucose measured following an intraperitoneal glucose injection. Western blots were used to visualize the expression of proteins in the whole brain lysate. Ultimately it was concluded that the high fat and sugar diet could successfully induce systemic insulin resistance, impair glucose uptake, and cause chronic neuroinflammation. Additionally, insulin resistance was observed in the brain resulting in biochemical changes to the rate of β-amyloid deposition, the formation of neurofibrillary tangles, and decrease in synaptic plasticity often seen in Alzheimer’s pathology. administered at twelve weeks of age also revealed that the mice on a high fat diet also showed a higher fasting insulin level, impaired glucose tolerance, and decreased insulin sensitivity (Figure B and Figure C). Following the glucose tolerance test, not only did the high fat diet mice show a higher concentration of blood glucose, they also required a longer period of time to bring these glucose levels back down. The decrease in glucose tolerance suggests a development in insulin resistance. These changes have previously been observed in similar studies and generally falls in line with the results of other publications. These metabolic changes are seen systemically, and the decrease in insulin sensitivity in the brain, as noted via HOMA-IR testing, (figure D) may be due to the increased degradation of the insulin receptor substrate-1 (IRS-1) protein. Additionally, the western blots of mice fed the fattier diet had a statistically significant decrease in the expression of glucose transporters GLUT1 and GLUT3 within the brain (figure E). These transporters are responsible for glucose uptake, and their decrease has also been noted in both diabetes and Alzheimer’s patients. Researchers also found an increase in the expression of β-secretase which is responsible for cleaving amyloid precursor protein (APP) into β-amyloid. On the other hand, expression of insulin degrading enzyme (IDE) responsible for moderating β-amyloid clearance, was found to have decreased. As a result of this dysregulation, β-amyloid plaque formation is observed. Furthermore, the researchers noted a visible increase in Tau phosphorylation. In its phosphorylated form, Tau encourages the growth of neurofibrillary tangles; this is also a major indicator of Alzheimer’s pathology.
Figure A: A comparison of the body weights between mice given the high fat and sugar diet versus the control group over a span of 13 weeks. (Kothari et al., 2017)
Major Results:
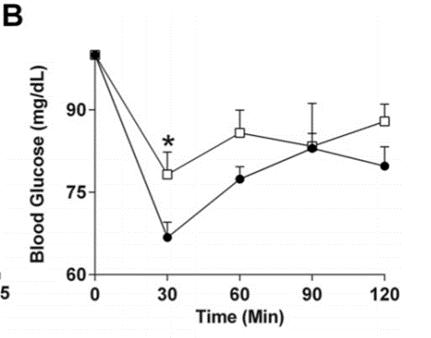
The mice given a diet high in fat and sugar all showed a statistically significant increase in total body mass in comparison to the control group (Figure A). The glucose tolerance test
Figure B: Fasting insulin levels taken at 12 weeks (left), and changes to blood glucose levels following an intraperitoneal injection of glucose (right). (Kothari et al., 2017).
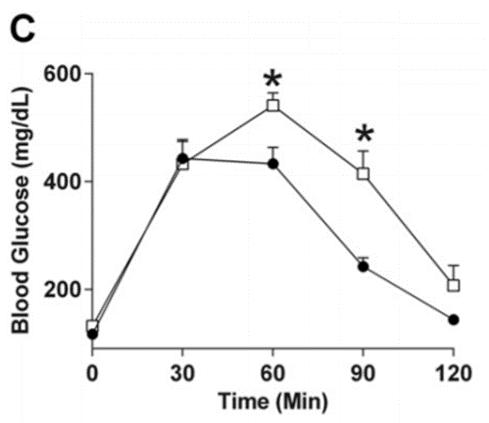
ages yet another pathological hallmark of Alzheimer’s known as neurofibrillary tangles. All together, the combination of changes stimulated by a fattier and sweeter diet are similar to the biological changes in Alzheimer’s patients. Therefore, diet itself could play a vital role in the process of cognitive decline. The data taken from this study shows that a high fat and sugar diet is able to induce insulin resistance within the brain, alter insulin signaling pathways within the brain, affect β-amyloid plaque formation, encourage neurofibrillary tangle formation, and decrease synaptic plasticity.
Figure C: Mice following a diet high in sugar and fats (white boxes) have impaired glucose tolerance due to decreased insulin sensitivity. This is shown by the higher levels of blood glucose (Kothari et al., 2017).
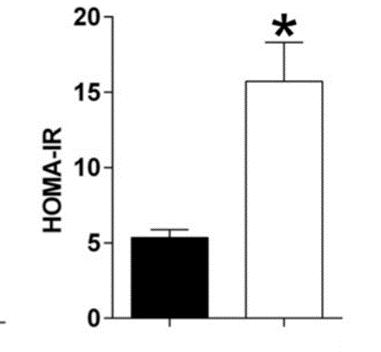
Figure D: HFS mice show higher insulin resistance in the HOMAIR test; measured in (µU/mL) x glucose (mg/dL)/405 (Kothari et al., 2017).
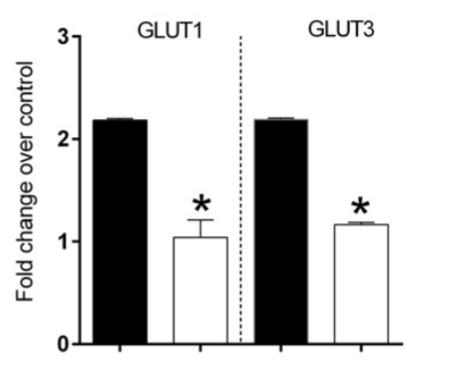
Figure E: Western blot and boxplot of corresponding expression of glucose transporters GLUT1 and GLUT3, which were significantly decreased in the mice given the high fat and sugar diet. Samples were taken from whole brain lysate after 13 weeks. (Kothari et al., 2017)
Critical Analysis Section
The results of this study were fascinating and covered a wide variety of bases. Going forward, it may be beneficial to run a similar study with varying levels of fat and sugar within the diet. Rather than confirming whether diet induced insulin resistance has the ability to cause changes, researchers should now focus on the level at which fats and sugars begin to impact insulin signaling and cognitive dysfunction. To do so, the levels of fat and sugar in the feed should be changed linearly with a control group given regular chow, a low fat high sugar control group, and a high fat low sugar control group. Since this study analyzed the effect of a fatty diet supplemented with sugar water, it may very well be that a combination of these two factors are responsible for the observed changes. By varying the composition of the mice’s feed, it would be possible to note whether a fatty diet, or sugary diet alone is able to induce these changes. Furthermore, Hanses (2018) proposed that obesity caused by high fat diets may not be the mechanism responsible for the difference in neuronal signaling, but rather dyslipidemia. An abnormal amount of lipid in the blood may be behind the changes to neuronal signaling and is thought to reduce cognitive function. Rather than using a higher fat diet to induce obesity, perhaps increasing serum lipid concentration would be sufficient in generating a response. Furthermore, it may be beneficial to have a regulated exercise regime for all mice rather than allow them exercise ad libitum. It was noted in the original experiment that the mice on the higher fat diet had an overall lower level of physical activity in comparison to the control group (Kothari et al., 2017). With mounting evidence that physical activity can ameliorate cognitive decline and the stimulation of glucose uptake via exercise (Brasure et al., 2018) and (Cockroft et al. 2019), it would be worthwhile to moderate their level of activity in order to remove physical activity levels as a confounding variable.
Discussion & Conclusion:
High fat diets potentiate systemic insulin resistance, thus resulting in altered protein expression of key transporters and proteins within the brain. Decreased glucose uptake in addition to the downregulation of insulin degrading enzymes are responsible for changing metabolic pathways. This will tip the balance between β-amyloid production and degradation and encourage the formation of β-amyloid plaques seen in Alzheimer pathology. Furthermore, the increase in Tau phosphorylation encour
1.
2.
3.
4.
5.
6.
7.
8.
9.
10.
11. Brasure, Michelle, Priyanka Desai, Heather Davila, Victoria A. Nelson, Collin Calvert, Eric Jutkowitz, Mary Butler, et al. “Physical Activity Interventions in Preventing Cognitive Decline and Alzheimer-Type Dementia.” Annals of Internal Medicine 168, no. 1 (December 19, 2017): 30–38. https://doi.org/10.7326/M17-1528. Cockcroft, Emma J., Bert Bond, Craig A. Williams, Sam Harris, Sarah R. Jackman, Neil Armstrong, and Alan R. Barker. “The Effects of Two Weeks High-Intensity Interval Training on Fasting Glucose, Glucose Tolerance and Insulin Resistance in Adolescent Boys: A Pilot Study.” BMC Sports Science, Medicine and Rehabilitation 11, no. 1 (December 2019): 29. https:// doi.org/10.1186/s13102-019-0141-9. Dittmann, Antje, Norman J Kennedy, Nina L Soltero, Nader Morshed, Miyeko D Mana, Ömer H Yilmaz, Roger J Davis, and Forest M White. “High-Fat Diet in a Mouse Insulin-Resistant Model Induces Widespread Rewiring of the Phosphotyrosine Signaling Network.” Molecular Systems Biology 15, no. 8 (August 1, 2019): e8849. https://doi.org/10.15252/ msb.20198849. Ferreira, Laís S. S., Caroline S. Fernandes, Marcelo N. N. Vieira, and Fernanda G. De Felice. “Insulin Resistance in Alzheimer’s Disease.” Frontiers in Neuroscience 12 (2018). https://doi.org/10.3389/fnins.2018.00830. Ferreira, Sergio T., Julia R. Clarke, Theresa R. Bomfim, and Fernanda G. De Felice. “Inflammation, Defective Insulin Signaling, and Neuronal Dysfunction in Alzheimer’s Disease.” Alzheimer’s & Dementia 10, no. 1S (February 1, 2014): S76–83. https://doi.org/10.1016/j.jalz.2013.12.010. Grice, Brian A., Kelly J. Barton, Jacob D. Covert, Alec M. Kreilach, Lixuan Tackett, Joseph T. Brozinick, and Jeffrey S. Elmendorf. “Excess Membrane Cholesterol Is an Early Contributing Reversible Aspect of Skeletal Muscle Insulin Resistance in C57BL/6NJ Mice Fed a Western-Style High-Fat Diet.” American Journal of Physiology-Endocrinology and Metabolism 317, no. 2 (June 25, 2019): E362–73. https://doi.org/10.1152/ajpendo.00396.2018. Hansen, Stine Normann, David Højland Ipsen, Anne Marie Schou-Pedersen, Jens Lykkesfeldt, and Pernille Tveden-Nyborg. “Long Term Westernized Diet Leads to Region-Specific Changes in Brain Signaling Mechanisms.” Neuroscience Letters 676 (May 29, 2018): 85–91. https://doi.org/10.1016/j.neulet.2018.04.014. Kang, Young-Ho, Mi-Hyang Cho, Ji-Young Kim, Min-Seo Kwon, Jong-Jin Peak, Sang-Wook Kang, Seung-Yong Yoon, and Youngsup Song. “Impaired Macrophage Autophagy Induces Systemic Insulin Resistance in Obesity.” Oncotarget 7, no. 24 (May 25, 2016): 35577–91. https://doi.org/10.18632/oncotarget.9590. Kasper, J. M., A. J. Milton, A. E. Smith, F. Laezza, G. Taglialatela, J. D. Hommel, and N. Abate. “Cognitive Deficits Associated with a High-Fat Diet and Insulin Resistance Are Potentiated by Overexpression of Ecto-Nucleotide Pyrophosphatase Phosphodiesterase-1.” International Journal of Developmental Neuroscience, Special Issue: Developmental Perspectives on Obesity and Energy Balance, 64 (February 1, 2018): 48–53. https://doi.org/10.1016/j.ijdevneu.2017.03.011. Koska, Juraj, Marlies K. Ozias, James Deer, Julie Kurtz, Arline D. Salbe, S. Mitchell Harman, and Peter D. Reaven. “A Human Model of Dietary Saturated Fatty Acid Induced Insulin Resistance.” Metabolism 65, no. 11 (November 1, 2016): 1621–28. https://doi.org/10.1016/j.metabol.2016.07.015. Kothari, Vishal, Yuwen Luo, Talia Tornabene, Ann Marie O’Neill, Michael W Greene, Thangiah Geetha, and Jeganathan Ramesh Babu. “High Fat Diet Induces Brain Insulin Resistance and Cognitive Impairment in Mice.” Biochimica et Biophysica Acta (BBA) - Molecular Basis of Disease 1863, no. 2 (February 1, 2017): 499–508. https://doi.org/10.1016/ j.bbadis.2016.10.006.