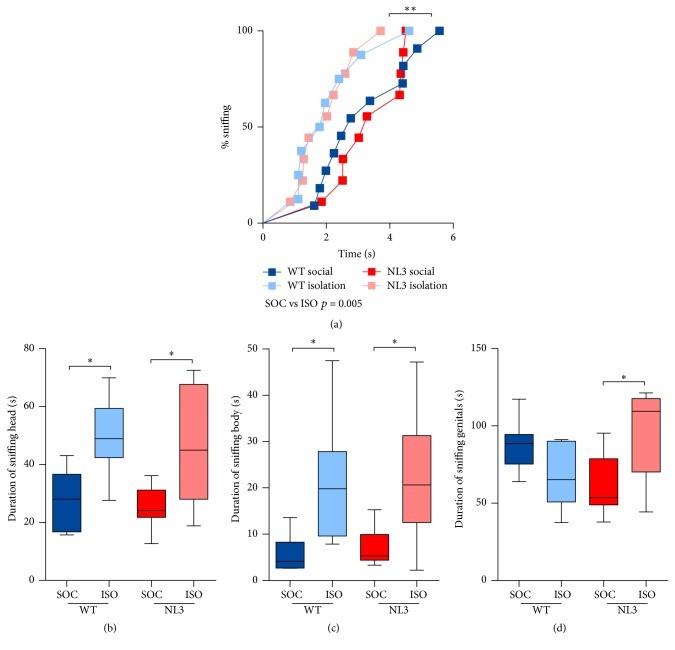
33 minute read
A Neuroligin 3 Mutation Results in Abnormal Behaviour in Mouse Model of Autism Spectrum Disorder
Liang Chen
Repetitive, obsessive, and restricted behaviours are usually seen in individuals with Autism Spectrum Disorder (ASD), along with impaired social interaction. ASD is one of the common neurodevelopmental disorders that has been studied in many mouse models. The development of various regions of the brain is different in imaging tests of autistic individuals. Mutations in genes such as SHANK3, Neuroligin (NLGN), and Neurexin (NRXN) have been discovered and contribute to the cause of ASD. However, genetic mutations are not the only cause of ASD but also environmental factors, too. Individuals with autism experience a range of symptoms; therefore, it is difficult to pinpoint the exact cause of ASD and it still remains unknown. A study conducted by Burrows et al. (2017) examines how the Neuroligin-3 R451C mutation (NL3 R451C ) in mouse model of ASD changes behaviours in social interaction and mating. The R451C mutation in Neuroligin-3 was originally found in a Swedish pair of siblings with ASD and Burrows et al. specifically investigated the mutation and wild type (WT) in male mice. Mice were pseudorandomly assigned to either social housing or isolation housing. The researchers identified that NL3 R451C mice from both housings had increased interest in mating and were more aggressive toward female mice, especially NL3 R451C mice from social housing. Thus, NL3 R451C mice provide useful insights concerning atypical behaviour in ASD.
Advertisement
INTRODUCTION
Many researchers continue to investigate autism spectrum disorder (ASD) despite its complex neurodevelopmental condition. There has been a gradual increase in the prevalence of ASD and there are more males who are affected by the disorder than females (Lai et al., 2014). Autistic individuals typically display repetitive and restricted behaviour and they have difficulty communicating with people (Lai et al., 2014; Lewis et al., 2007). ASD is mainly caused by genetic factors with a relatively high heritability (Abrahams & Geschwind, 2008). Further evidence supported by a study that illustrates the likelihood of inheriting social impairments for ASD is about 61% and the concordance rates for identical twins and fraternal twins are 80% and 13.6%, respectively (Deng et al., 2015). Moreover, many genetic mutations linked with ASD influence proteins that are essential for appropriate synaptic growth and function (Voineagu et al., 2011). Specifically, genetic mutations that encode single-pass transmembrane proteins such as neuroligin and neurexin have been discovered (Cao & Tabuchi, 2017; Jamain et al., 2003). Neurexin and neuroligin interact with each other on both the presynaptic and postsynaptic ends, which they are also called cell-adhesion molecules (Betancur et al., 2009; Cao & Tabuchi, 2017; Jamain et al., 2003). Interruption to these cell-adhesion molecules may uncover novel understanding of the mechanisms of synaptic dysfunction in ASD (Betancur et al., 2009). Besides genetic factors that are, for the most part, responsible for ASD, environmental factors are just as important to be taken into consideration since some could function as risk factors stimulating the development of ASD. Fortunately, environmental factors can be minimized and prevented from increasing the risk of giving birth to an autistic child. Pregnant mothers who have high intake of fatty acids in the first trimester lower the risk of giving birth to autistic newborns by 34% (Karimi et al., 2017). However, other environmental factors such as the MMR vaccine is found to be unassociated with ASD (Karimi et al., 2017; Taylor et al., 2002). ASD is indeed a complicated disorder that is caused not only by one factor but a mixture of numerous environmental and genetic risk factors. mones. This study demonstrated that the genetic mutation of NL3 R451C is involved in abnormal social behaviour that represents characteristics of ASD.
MAJOR RESULTS
Social interaction in WT and NL3 R451C mice
WT and NL3 R451C
mice’s outcomes of different types of social interaction were recorded. The research was conducted over two stages (two exposure with three minute break in between) for two weeks (once a week) (Burrows et al., 2017). There were ten WT; ten NL3 R451C mice in social housing, and nine WT; nine NL3 R451C mice in isolation housing. All mice contacted their female mouse in less than six seconds, and thus no differences in groups were observed in sniffing latency (Figure 1a). WT and NL3 R451C mice from isolation housing exhibited enhanced sociability toward the female mouse where they spent longer duration of sniffing the female’s head and body (Figure 1b & 1c) as well as sniffing the female mouse’s genital region (Figure 1d). The results are in agreement with a previous study that socially isolated mice are more eager to be involved in social interaction (Chabout et al., 2012).
Burrows et al. used WT and NL3 R451C mice that were obtained from pairing NL3 R451C male mice with heterozygous female mice. This produced even number of WT and NL3 R451C male progenies. Mice were pseudorandomly assigned to social housing (consisted of three to four mice in each housing) or isolation housing. All mice from both housing conditions subsequently experienced the Male-Female Social Interaction Test (MFSIT) at the age of 14 to 20 weeks where they interact with a new sexually full-grown female mouse individually. Total of two MFSIT were conducted once a week and each female mouse was paired with one male mouse pseudorandomly for each day. The Female Urine Sniffing Test (FUST) was also conducted. NL3 R451C mice displayed atypical behaviour in terms of aggressiveness toward female mice and increased interest in mating. Socially isolated WT and NL3 R451C mice had increased interest in interacting with female mice as well as NL3 R451C mice from social housing showed a lack of interest in exploring female pheroFigure 1. (a) No differences in groups were observed where all mice contacted female mouse within six seconds. (b)&(c) Longer duration of sniffing female mouse’s head and body in WT and NL3 R451C mice from isolation housing. (d) NL3 R451C mice from isolation housing were more interested in sniffing female mouse’s genital. The results in each graph is an average of four tests from the two stages. Figure derived from Burrows et al. (2017)
Mating behaviour in WT and NL3 R451C mice
There were ten WT; ten NL3 R451C mice in social housing, and nine WT; nine NL3 R451C mice in isolation housing (Burrows et al.,
2017). The research was conducted over two stages for two weeks. No differences were observed in mounting latency between all mice where they were all faster to mount during the first test after they had been separated for a period of time and were slower during the second test a week after (Burrows et al., 2017)(Figure 2a). The number of mounts is greater in NL3 R451C mice than WT mice, especially NL3 R451C mice from social housing (Figure 2b). NL3 R451C mice also spent longer duration of mounting the female mouse than WT mice (Figure 2c). All mice spent longer time mounting the female mouse when the female was the same for both stages (Burrows et al., 2017). Conversely, there was a decrease in mounting duration when a different female mouse was paired in the second stage (Burrows et al., 2017).
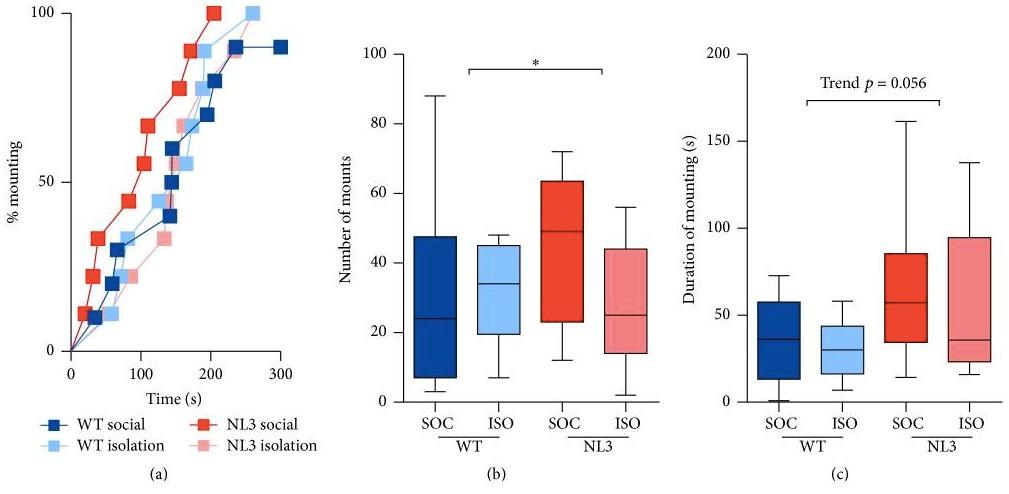
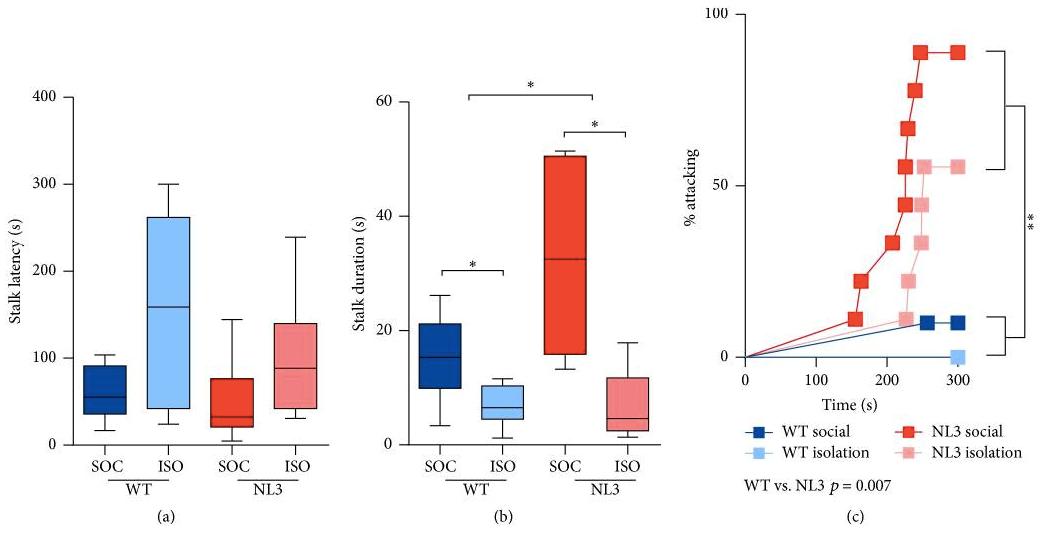
Figure 3. (a) No effect of genotype on latency to stalk was observed. (b) NL3 R451C mice from social housing stalked female mouse for longer time, whereas WT and NL3 R451C mice from isolation housing had shorter stalk duration. (c) NL3 R451C mice had higher percentage of attacking female mice than WT mice, especially NL3 R451C mice from isolation housing. The results in each graph is an average of four tests from the two stages. Figure derived from Burrows et al. (2017) exploring and sniffing female mice’s urine based on FUST (Figure 4), whereas NL3 R451C mice from isolation housing were
Figure 2. (a) All mice were faster to mount during the first test after they had been separated for a period of time and were slower during the second test a week after, and thus no differences were observed. (b) NL3 R451C mice mounted more times than WT mice, especially NL3 R451C mice from social housing. (c) NL3 R451C mice spent longer time mounting female mouse than WT mice. The results in each graph is an average of four tests
NL3 R451C
mice from social housing showed a lack of interest in from the two stages. Figure derived from Burrows et al. (2017)
highly interested in examining female mice’s urine where the levels of duration is similar to WT mice (Figure 4).
Unacceptable behaviour in NL3 R451C Urine Sniffing Test (FUST) mice and the Female
There were ten WT; ten NL3 R451C mice in social housing, and nine WT; nine NL3 R451C mice in isolation housing (Burrows et al., 2017). The research was conducted over two stages for two weeks. No effect of genotype on latency to stalk was observed (Figure 3a) and NL3 R451C mice from social housing stalked the female mouse for longer time (Figure 3b). WT and NL3 R451C mice from isolation housing had shorter stalk duration (Figure 3b). Furthermore, NL3 R451C mice were hostile toward female mice and had higher chances of attacking female mice, especially NL3 R451C mice from social housing (Figure 3c). These results are consistent with a recently study done on NL3 R451C mice that displayed atypical hostile behaviour (Hosie et al., 2018).
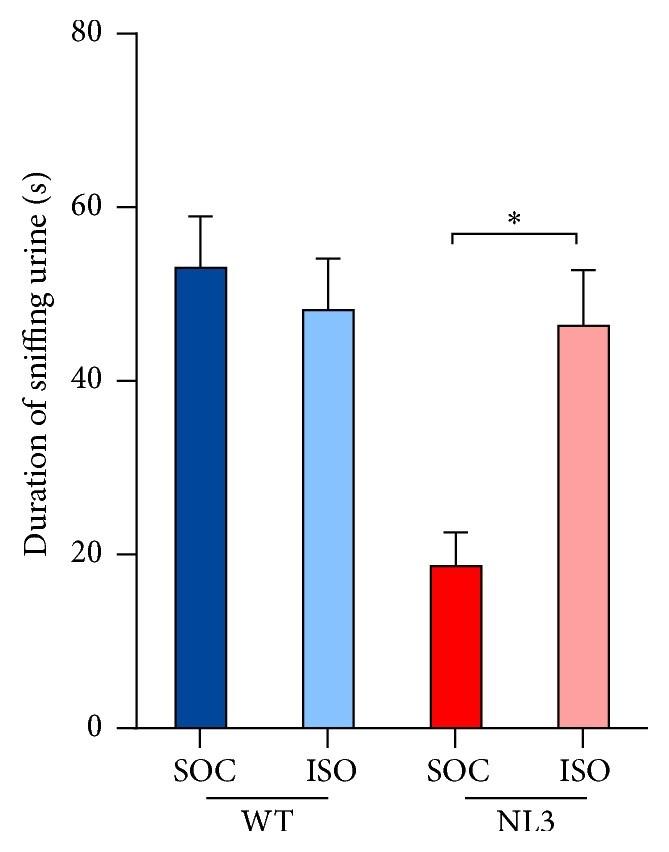
Figure 4. NL3 R451C mice from social housing was uninterested in sniffing female mice’s urine, whereas NL3 R451C mice from isolation housing were interested in sniffing female urine just as much as WT mice. The results in each graph is an average of four tests from the two stages. Figure derived from Burrows et al. (2017)
CONCLUSION/DISCUSSION
Burrows et al. (2017) concluded that the mutation of NL3 R451C plays a role in altering social behaviour in mouse model of ASD where mice behave abnormally in terms of being hostile to-
ward female mice and wanting to mate more. Mice from isolation housing were more motivated to socialize with female mice no matter what the genotype is and NL3 R451C mice from social housing were uninterested in sniffing female mice’s urine.
Aggressive behaviour is common between male mice since it is an intrinsic, social behaviour that is essential to gain social determine mating drive in NL3 R451C mice. By comparing the baseline measure to the measure after being introduced to a
ranking and available resources from the surroundings (Miczek et al., 2001), but exhibiting hostile behaviour toward female iour in the hypothalamus, the researchers could excite or inhibexamined areas in the hypothalamus and olfactory systems are
mice is aberrant. Burrows et al. stated that NL3 R451C mice’s abnormal aggression might not be territorial because they were being aggressive toward female mice as well. In addition, NL3 R451C mice exhibiting atypical hostile behaviour has been reported that it is due to low levels of serotonin in the brain where they do not have sufficient tryptophan hydroxylase 2 in the brain (Beis et al., 2015).
Mice sense pheromones to identify and interact with individuals. NL3 R451C mice that displayed a lack of interest in exploring urine could be the cause to their hostility toward female mice. The lack of interest could suggest that NL3 R451C mice may have olfactory dysfunction. However, a previous study showed that mice had no problem discriminating between different types of odors, including urine (Burrows et al., 2015). Moreover, NL3 R451C mice from isolation housing explored pheromones in urine just as much as WT mice, implying that social housing might be a factor in affecting the levels of interest in exploring female mice’s urine.
NL3 R451C
mice exhibited high mating drive where they mounted female mice more times and for longer duration. This may be due to the concentration differences in blood testosterone bemating drive is influenced by testosterone. One possible experiment is that first, laboratory mice are castrated before conTestosterone is given through injection. The researchers then pair each male mouse to a female mouse in a separate room group and the experimental group. The expected outcomes
fore and after introducing to a female (Burrows et al., 2017).
Collectively, this neuroligin-3 R451C mutation does have an impact on modifying social behaviour in mice, which is also seen in individuals with ASD.
CRITICAL ANALYSIS
The present study conducted by Burrows et al. (2017) obtained several fascinating findings that are in line with other literature in regard to the types of dysfunctional behaviour such as atypical aggression that was detected in NL3 R451C mice (Burrows et al., 2015; Miczek et al., 2001). Nevertheless, experiments remain to be performed are what brain regions are affected by NL3 R451C that brings about abnormal behaviour in NL3 R451C mice. Experiments on brain regions such as hypothalamus and olfactory systems should be performed as well as the levels of neurotransmitters such as serotonin. Besides regulating homeostasis, the hypothalamus is known to release hormones that have are crucial to engage in social interaction and investigation (Hashikawa et al., 2016). Experiments on brain regions could potentially unveil additional insights into atypical behaviour caused by NL3 R451C mutation. Further studies are required in assessing the concentration of testosterone in the blood to female mouse, the level of mating drive can be established based on the concentration difference obtained.
FUTURE DIRECTIONS
Future studies should concentrate on examining specific areas in the hypothalamus and olfactory systems that are responsible for different behavioral outcomes such as aggression in NL3 R451C mice. The technique of optogenetics could be employed to inhibit or excite particular parts in these brain regions. If a specific group of neurons is thought to modulate aggressive behavit those particular neurons and observe the behavioural outcomes of the mouse. Similar procedure applies to olfactory systems. The expected outcomes of the hypothalamus would be if those specific neurons were being excited, the mouse should display hostile behaviour because the area responsible for aggression behaviour is activated. When those neurons are inhibited, the mouse should behave less hostile. The expected outcomes of olfactory systems would be if those specific neurons were inhibited, the mouse should become more aggressive since it could be difficult to identify individuals or could misinterpret other mice as strangers. If no aggressive behaviour is observed in NL3 R451C mice, then it can be concluded that the not responsible for eliciting hostile behaviour.
Future studies should also concentrate on examining whether ducting the study. Mice then are separated into two groups –castrated mice that do not receive testosterone (control) and castrated mice that receive testosterone (experimental group). and see whether there is a difference between the control an effect on sexual and social behaviours, and olfactory systems
would be the experimental group has higher mating drive than the control group. If no difference in mating drive is observed, then it can be concluded that testosterone does not have any effect on mating drive and that other factors might be involved in regulating mating drive instead.
1.
2.
3.
4.
5.
6.
7.
8.
9.
10.
11.
12.
13.
14.
15.
16.
17. Abrahams, B. S., & Geschwind, D. H. (2008). Advances in autism genetics: On the threshold of a new neurobiology. Nature Reviews Genetics, 9(5), 341–355. https://doi.org/10.1038/nrg2346 Beis, D., Holzwarth, K., Flinders, M., Bader, M., Wöhr, M., & Alenina, N. (2015). Brain serotonin deficiency leads to social communication deficits in mice. Biology Letters, 11(3). https://doi.org/10.1098/rsbl.2015.0057 Betancur, C., Sakurai, T., & Buxbaum, J. D. (2009). The emerging role of synaptic cell-adhesion pathways in the pathogenesis of autism spectrum disorders. Trends in Neurosciences, 32(7), 402–412. https://doi.org/10.1016/j.tins.2009.04.003 Burrows, E. L., Eastwood, A. F., May, C., Kolbe, S. C., Hill, T., McLachlan, N. M., Churilov, L., & Hannan, A. J. (2017). Social Isolation Alters Social and Mating Behavior in the R451C Neuroligin Mouse Model of Autism. Neural Plasticity, 2017. https://doi.org/10.1155/2017/8361290 Burrows, Emma L., Laskaris, L., Koyama, L., Churilov, L., Bornstein, J. C., Hill-Yardin, E. L., & Hannan, A. J. (2015). A neuroligin-3 mutation implicated in autism causes abnormal aggression and increases repetitive behavior in mice. Molecular Autism, 6(1), 62. https://doi.org/10.1186/s13229-015-0055-7 Cao, X., & Tabuchi, K. (2017). Functions of synapse adhesion molecules neurexin/neuroligins and neurodevelopmental disorders. Neuroscience Research, 116, 3–9. https://doi.org/10.1016/j.neures.2016.09.005 Chabout, J., Serreau, P., Ey, E., Bellier, L., Aubin, T., Bourgeron, T., & Granon, S. (2012). Adult Male Mice Emit ContextSpecific Ultrasonic Vocalizations That Are Modulated by Prior Isolation or Group Rearing Environment. PLoS ONE, 7(1). https://doi.org/10.1371/journal.pone.0029401 Deng, W., Zou, X., Deng, H., Li, J., Tang, C., Wang, X., & Guo, X. (2015). The Relationship Among Genetic Heritability, Environmental Effects, and Autism Spectrum Disorders: 37 Pairs of Ascertained Twin Study. Journal of Child Neurology, 30(13), 1794–1799. https://doi.org/10.1177/0883073815580645 Hashikawa, K., Hashikawa, Y., Falkner, A., & Lin, D. (2016). The neural circuits of mating and fighting in male mice. Current Opinion in Neurobiology, 38(Complete), 27–37. https://doi.org/10.1016/j.conb.2016.01.006 Hosie, S., Malone, D. T., Liu, S., Glass, M., Adlard, P. A., Hannan, A. J., & Hill-Yardin, E. L. (2018). Altered Amygdala Excitation and CB1 Receptor Modulation of Aggressive Behavior in the Neuroligin-3R451C Mouse Model of Autism. Frontiers in Cellular Neuroscience, 12. https://doi.org/10.3389/fncel.2018.00234 Jamain, S., Quach, H., Betancur, C., Råstam, M., Colineaux, C., Gillberg, I. C., Soderstrom, H., Giros, B., Leboyer, M., Gillberg, C., & Bourgeron, T. (2003). Mutations of the X-linked genes encoding neuroligins NLGN3 and NLGN4 are associated with autism. Nature Genetics, 34(1), 27–29. https://doi.org/10.1038/ng1136 Karimi, P., Kamali, E., Mousavi, S. M., & Karahmadi, M. (2017). Environmental factors influencing the risk of autism. Journal of Research in Medical Sciences : The Official Journal of Isfahan University of Medical Sciences, 22. https:// doi.org/10.4103/1735-1995.200272 Lai, M.-C., Lombardo, M. V., & Baron-Cohen, S. (2014). Autism. The Lancet, 383(9920), 896–910. https://doi.org/10.1016/ S0140-6736(13)61539-1 Lewis, M. H., Tanimura, Y., Lee, L. W., & Bodfish, J. W. (2007). Animal models of restricted repetitive behavior in autism. Behavioural Brain Research, 176(1), 66–74. https://doi.org/10.1016/j.bbr.2006.08.023 Miczek, K. A., Maxson, S. C., Fish, E. W., & Faccidomo, S. (2001). Aggressive behavioral phenotypes in mice. Behavioural Brain Research, 125(1–2), 167–181. https://doi.org/10.1016/S0166-4328(01)00298-4 Taylor, B., Miller, E., Lingam, R., Andrews, N., Simmons, A., & Stowe, J. (2002). Measles, mumps, and rubella vaccination and bowel problems or developmental regression in children with autism: Population study. BMJ : British Medical Journal, 324(7334), 393–396. Voineagu, I., Wang, X., Johnston, P., Lowe, J. K., Tian, Y., Horvath, S., Mill, J., Cantor, R. M., Blencowe, B. J., & Geschwind, D. H. (2011). Transcriptomic analysis of autistic brain reveals convergent molecular pathology. Nature, 474(7351), 380–384. https://doi.org/10.1038/nature10110
Behavioural and synaptic effect from EAAT3 overexpression on obsessive-compulsive disorder
Yi -Chin Chen
Obsessive compulsive disorder (OCD) is a neurodevelopmental disorder that involves in repetitive thoughts and behaviours that are unwanted. Up to 3% of the general population are affected by this disorder and the symptoms can persist chronically if left untreated. Currently, antidepressant and cognitive behavioural therapy are the two main treatment that aids in alleviating OCD symptoms. A cure for this disorder has yet to be found. Delgado-Acevedo et al. (2019) explored the overexpression effect of EAAT3 on OCD and found an increase in anxious and repetitive behaviours along with greater spontaneous recovery of fear. They also found altered corticostriatal synapses from overexpression of EAAT3. These findings provided evidences and connection between the involvement of EAAT3 expression on OCD-related behaviours. Therefore, EAAT3 could be a potential therapeutic target for OCD treatment.
Key words: obsessive-compulsive disorder (OCD), solute carrier family 1 member 1 (SLC1A1), excitatory amino acid transporter 3 (EAAT3), repetitive behaviours, N-methyl-D-aspartate receptor (NMDAR), GluN2B subunit, fear extinction, glutaminergic system, corticostrial synapse
Obsessive compulsive disorder (OCD) is a neurodevelopmental disorder that involves compulsive behaviours and persistent thoughts (Abramowitz et al., 2009). It affects 2-3% of adults in the general population and the symptoms can be chronic if left (Figure 1A). In the light-dark exploration test, EAAT3 glo /CMKII mice spends more time in the dark compartment as oppose to
untreated (Mataix-Cols et al., 2002; S. E. Stewart et al., 2004). Antidepressants, such as serotonin reuptake inhibitors, are often prescribed with or without conjunction of cognitive behavioral therapy to help alleviate OCD symptoms (Abramowitz et al., 2009). However, a cure for OCD has not yet been found.
Studies have implicated that defective glutaminergic system are linked to OCD (Brennan et al., 2013; Chakrabarty et al., 2005). Specifically, the glutaminergic neurons in the cortico-striatalthalamic-cortical (CSTC) circuitry is hyperactive in OCD (Hajcak & Simons, 2002; Johannes et al., 2001). The hyperactivity results from alternation of glutaminergic NMDR receptors that will ultito EAAT3 glo /CMKII mice for one day or 21 days (Figure 1). This result supports literature evidences on antidepressant as a treat
mately promote synaptic plasticity (Pittenger, 2015). Hence, the reoccurrence of the intrusive thoughts leading to repetitive behaviours can be seen in OCD.
Although there are environmental contributors to this disorder, studies on twins suggested genetic factors can held up to 65% liability for the observed OCD symptoms (Grootheest et al., 2005). In particular, solute carrier family 1 member 1 (SLC1A1) has been identified as a candidate gene for OCD that expresses excitatory amino acid transporter 3 (EAAT3), a glutamate transporter (Pittenger, 2015; Ting & Feng, 2008). EAAT3 can regulate synaptic level of glutamate and N-methyl-D-aspartate receptor (NMDAR) function (Bjørn-Yoshimoto & Underhill, 2016; Escobar et al., 2019). Previous studies has shown that EAAT3 knockout mice shows unaltered baseline anxious and repetitive behaviours (González et al., 2017; Peghini et al., 1997; Quinlan et al., 1999; Zike et al., 2017). Several SLC1A1 variants were found to promote transcription of SLC1A1 in OCD brain (Liang et al., 2008; Shugart et al., 2009; S. Evelyn Stewart et al., 2007). Therefore,
Overexpression of EAAT3 increases anxious and repetitive behaviours
In the original study, Delgado-Acevedo et al. (2019) conducted four behaviour tests and found increases in anxious and repetitive behaviours in transgenic EAAT3 overexpressing mice, EAAT3 glo /CMKII (Figure 1). In the open field test, EAAT3 glo /CMKII mice spends less time in the box center than the control mice control mice (Figure 1E). In the marble burying test, more marbles were buried by EAAT3 glo /CMKII mice than the control mice (Figure 1F). In the grooming analysis, EAAT3 glo /CMKII mice spends more time grooming than the control mice (Figure 1G).
Additionally, chronic treatment of antidepressants was found to decrease anxious and repetitive behaviours in EAAT3 glo /CMKII mice. Administration of fluoxetine or clomipramine were given EAAT3 overexpression may increase susceptibility to OCD.
ment to alleviate anxious and repetitive behaviours in OCD (Abramowitz et al., 2009).
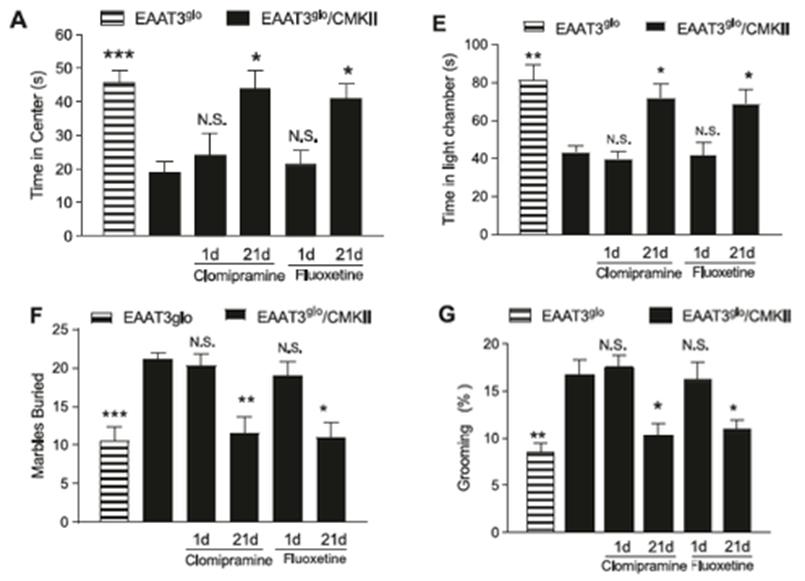
The original paper by Delgado-Acevedo et al. (2019) investigated the effect of EAAT3 overexpression on behaviour and synapses of OCD. Transgenic mice were used to overexpress EAAT3. The mice then underwent a series of behavioral tests and fear conditioning and extinction tasks. Subsequently, oral administration of antidepressants was given to the mice acutely or chronically. Corticostraital synapses were examined using electrophysiological and molecular analyses. An increase in anxious and compulsive behaviours as well as greater spontaneous recovery of fear were evident in EAAT3 overexpressed mice. Results from the analyses showed changes in NMDAR subunits in EAAT3 overexpressed mice. Overall, this study provides evidence on involvement of glutamatergic system at play in OCD and managing levels of EAAT3 as a possible new therapeutic target.
Figure 1.
The results of the four behaviour tests and the effect of antidepressants on overexpressing EAAT3 mice (EAAT3 glo /CMKII). EAAT3 glo is the control mice. Panel A shows the results from the open field test. Panel E shows the results from the light-dark exploration test. Panel F shows the result from the marble burying test. Panel G shows the results from the grooming analysis. Clomipramine and fluoxetine are the administered antidepressants. Figure adapted from Delgado-Acevedo et al. (2019).
In the study by Delgado-Acevedo et al. (2019), they also examined the performance of EAAT3 glo /CMKII mice in the fear conditioning and extinction tasks (Figure 2). One day after fear learning, EAAT3 glo /CMKII mice showed higher tendency to freeze from extinction retrieval than the control mice (Figure 2C). EAAT3 glo /CMKII mice also showed higher tendency to freeze from spontaneous recovering of fear than the control mice two weeks after fear learning (Figure 2D). This result supports welldocumented OCD characteristics of having elevated fear and trouble in extinction of fear (Fullana et al., 2014; Milad et al., 2013). Several SNP in SLC1A1 gene has been identified in OCD studies that correlate with increase SLC1A1 expression in the OCD brain (Liang et al., 2008; Shugart et al., 2009; S. Evelyn Stewart et al., 2007). Delgado-Acevedo et al. (2019) thereby hypothesized that expression of EAAT3 is positively correlated with OCD susceptibility. By using transgenic EAAT3 overexpressing mice (EAAT3 glo / CMKII), Delagto et al. 2019 was able to induce a series of OCDrelated behaviours. The results showed support to the proposed hypothesis. Further indirect evidences that reinforces the hypothesis was found in previous studies where baseline OCDrelated behaviours in EAAT3 knockout mice remained unaffected (González et al., 2017; Peghini et al., 1997; Quinlan et al., 1999; Zike et al., 2017). Grooming analysis was one of the behavioural tests carried out to examine presence of repetitive behaviours. Increased groom time was found in EAAT3 glo /CMKII mice (Figure 1G). However, the extent of grooming in EAAT3 glo /CMKII mice did not reach the point of injury. Conversely, knockout model of other OCD candidate genes, SAPAP3 and SLITRK5, showed excess grooming to used as a measure of validity for studies on OCD due to its prev
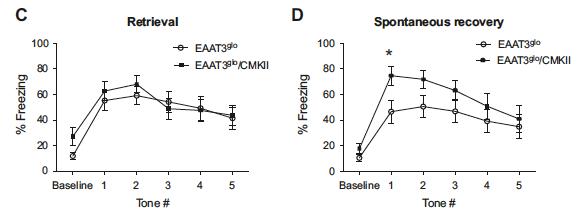
Figure 2. The results on extinction retrieval and spontaneous recovery of fear on overexpressing EAAT3 mice (EAAT3 glo /CMKII). EAAT3 glo is the control mice. Panel C shows amount of freezing during retrieval given the number of conditioned tone. Panel D shows the amount of freezing during spontaneous recovery of fear given the number of conditioned tone presented. Figure adapted from Delgado-Acevedo et al. (2019).
Overexpression of EAAT3 is associated with corticostriatal alternations
When Delgado-Acevedo et al. (2019) examined the corticostriatal synapses of EAAT3 glo /CMKII mice using western blot, it was found NMDAR that contains GluN2B subunit are more prevalent than GluN2A-containing NMDAR. High-frequency afferent stimulation that was carried out at the synapse failed to induce NMDA -dependent long-term depression in EAAT3 glo /CMKII mice. These results reinforces previous evidences on EAAT3 ability to regulate the function of NMDAR to promote hyperactivity and synaptic plasticity in CSTC that render the observed restless behaviours and thoughts in OCD (Bjørn-Yoshimoto & Underhill, 2016; Escobar et al., 2019). The results ultimately support evidences on involvement of the glutaminergic system in OCD through alteration in NMDAR composition (Brennan et al., 2013; the point of skin bleeding (Shmelkov et al., 2010; Welch et al., 2007). Expression level may differ among all the candidate genes that account for the spectrum differences observed in OCD phenotype. Additionally, the administration of antidepressants, clomipramine and fluoxetine, was used in the study to relieve anxious and repetitive behaviours like those of excess grooming observed in EAAT3 glo /CMKII mice. Antidepressants are mainly
Chakrabarty et al., 2005). alence as treatment for OCD (Chakrabarty et al., 2005; Coric et al., 2005).
EAAT3 glo /CMKII mice was also shown to have a greater spontaneous recovery of fear, an indicator of defect in retaining fear extinction that can be seen in OCD individuals and from previous OCD-related studies (Figure 2D)(Milad et al., 2013; Reimer et al., 2018). Ventromedial prefrontal cortex (vmPFC) and amygdala are areas of the brain involved in fear extinction (Burgos-Robles et al., 2007; Do-Monte et al., 2010; Milad et al., 2007, 2013). Previous studies on OCD has linked reduced activation in both vmPFC and amygdala to decreased ability to retain fear extinction (Milad et al., 2013; Phelps et al., 2004). Therefore, overexpression EAAT3 mice may have a low activation of vmPFC and amygdala that give rise to the observed fear extinction deficits.
DISCUSSIONS/ CONCLUSIONS
The study by Delgado-Acevedo et al. (2019) was able to demonstrate overexpression of EAAT3 increases repetitive behaviours, promote changes in corticostriatal synapses and decrease susceptibility to fear extinction. These findings are evidences in supporting connection between EAAT3 and OCD pathogenesis. Ultimately, the results implicated that EAAT3 can be a potential therapeutic target for OCD due to its ability to alter OCD-related behaviours from level of expression. Many studies have implicated that functions of NMDAR can differ depending on the subunit composition of the receptor (Das et al., 1998; Paoletti et al., 2013). Specifically, previous studies have found involvement of GluN2B-containing NMDAR in generating long term depression and subsequently represses conditioned fear response in fear extinction whereas GluN2Acontining NMDAR in generating long term potentiation (Dalton et al., 2012; Park et al., 2012). In one study, highly anxious rat was found to express more GluN2B-containing NMDAR in the prefrontal cortex and amygdala (Lehner et al., 2011). This is line with the results obtained by Delgado-Acevedo et al. (2019)
where elevated level of GluN2B-containing NMDAR in corticostriatal synapse of EAAT3 glo /CMKII mice which has been shown to exhibit anxious behaviours. The evidences above supported the role of EAAT3 on altering NMDAR function.
CRITICAL ANALYSIS
Little to no significant discrepancy was found in the literature that opposes the findings by Delgado-Acevedo et al. (2019). In line with the results from Delgado-Acevedo et al. (2019), similar results has been found examining other OCD candidate genes, SAPAP3 and SLITRK5 (Shmelkov et al., 2010; Welch et al., 2007). SAPAP3 and SLITRK5 along with EAAT3 are all expressed in the corticostriatal synapse (Delgado-Acevedo et al., 2019; Shmelkov et al., 2010; Welch et al., 2007). Grooming behaviours was explored in SAPAP3 knockout (SAPAP3 KO) mice, SLITRK5 knockout (SLITRK5 KO) mice, and EAAT3 overexpressing (EAAT3 glo /CMKII) mice (Delgado-Acevedo et al., 2019; Shmelkov et al., 2010; Welch et al., 2007). All three mice model showed prolonged grooming behaviour with SAPAP3 KO and SLITRK5 KO to the point of self-injury (Delgado-Acevedo et al., 2019; Shmelkov et al., 2010; Welch et al., 2007). Open field test in all three mice model also showed little time spend in the center of the box, indicating presence of anxiety in all the mice (DelgadoAcevedo et al., 2019; Shmelkov et al., 2010; Welch et al., 2007). Similar to EAAT3 glo /CMKII mice, marble burying test was explored in SLITRK KO mice where increase marble burying was also found (Delgado-Acevedo et al., 2019; Shmelkov et al., 2010). Light-dark exploration test was explored in both EAAT3 glo /CMKII mice and SAPAP3 KO mice and both showed longer time spent in the dark (Delgado-Acevedo et al., 2019; Welch et al., 2007). Overall, behaviour tests conducted among the three mice model all showed OCD-related behaviours. Upon chronic administration of antidepressant, all three model showed alleviation on OCD-related behaviours. Additionally, corticostriatal electrophysiology was also carried out on SAPAP3 mice (Welch et al., 2007). Similar to the electrophysiological results from EAAT3 glo /CMKII mice, activity of NMDAR activities were upregulated (Welch et al., 2007).
FUTURE DIRECTION
Future studies can incorporate positron emission tomography (PET) imaging techniques to visually observe areas of the EAAT3 glo /CMKII mice brain activity upon fear learning and extinction paradigm. Overexpression EAAT3 mice will first be transgenically generated. A synthetic radiotracers can injected into the mice. A signal will be generated by the radiotracers that can be measured by specialized equipment. Success of the experiment will show decreased brain activity in vmPFC and amygdala in extinction retrieval. Failure of the experiment will show decreased activity in other brain regions.
10.
11.
12.
13.
14.
15.
16. Abramowitz, J. S., Taylor, S., & McKay, D. (2009). Obsessive-compulsive disorder. The Lancet, 374(9688), 491–499. https:// doi.org/10.1016/S0140-6736(09)60240-3 Bjørn-Yoshimoto, W., & Underhill, S. M. (2016). The Importance of the Excitatory Amino Acid Transporter 3 (EAAT3). Neurochemistry International, 98, 4–18. https://doi.org/10.1016/j.neuint.2016.05.007 Brennan, B. P., Rauch, S. L., Jensen, J. E., & Pope, H. G. (2013). A critical review of magnetic resonance spectroscopy studies of obsessive-compulsive disorder. Biological Psychiatry, 73(1), 24–31. https://doi.org/10.1016/j.biopsych.2012.06.023 Burgos-Robles, A., Vidal-Gonzalez, I., Santini, E., & Quirk, G. J. (2007). Consolidation of Fear Extinction Requires NMDA Receptor-Dependent Bursting in the Ventromedial Prefrontal Cortex. Neuron, 53(6), 871–880. https://doi.org/10.1016/ j.neuron.2007.02.021 Chakrabarty, K., Bhattacharyya, S., Christopher, R., & Khanna, S. (2005). Glutamatergic Dysfunction in OCD. Neuropsychopharmacology, 30(9), 1735–1740. https://doi.org/10.1038/sj.npp.1300733 Coric, V., Taskiran, S., Pittenger, C., Wasylink, S., Mathalon, D. H., Valentine, G., Saksa, J., Wu, Y.-T., Gueorguieva, R., Sanacora, G., Malison, R. T., & Krystal, J. H. (2005). Riluzole augmentation in treatment-resistant obsessive-compulsive disorder: An open-label trial. Biological Psychiatry, 58(5), 424–428. https://doi.org/10.1016/j.biopsych.2005.04.043 Dalton, G. L., Wu, D. C., Wang, Y. T., Floresco, S. B., & Phillips, A. G. (2012). NMDA GluN2A and GluN2B receptors play separate roles in the induction of LTP and LTD in the amygdala and in the acquisition and extinction of conditioned fear. Neuropharmacology, 62(2), 797–806. https://doi.org/10.1016/j.neuropharm.2011.09.001 Das, S., Sasaki, Y. F., Rothe, T., Premkumar, L. S., Takasu, M., Crandall, J. E., Dikkes, P., Conner, D. A., Rayudu, P. V., Cheung, W., Chen, H.-S. V., Lipton, S. A., & Nakanishi, N. (1998). Increased NMDA current and spine density in mice lacking the NMDA receptor subunit NR3A. Nature, 393(6683), 377–381. https://doi.org/10.1038/30748 Delgado-Acevedo, C., Estay, S. F., Radke, A. K., Sengupta, A., Escobar, A. P., Henríquez-Belmar, F., Reyes, C. A., Haro-Acuña, V., Utreras, E., Sotomayor-Zárate, R., Cho, A., Wendland, J. R., Kulkarni, A. B., Holmes, A., Murphy, D. L., Chávez, A. E., & Moya, P. R. (2019). Behavioral and synaptic alterations relevant to obsessive-compulsive disorder in mice with increased EAAT3 expression. Neuropsychopharmacology, 44(6), 1163–1173. https://doi.org/10.1038/s41386-018-0302-7 Do-Monte, F. H. M., Kincheski, G. C., Pavesi, E., Sordi, R., Assreuy, J., & Carobrez, A. P. (2010). Role of beta-adrenergic receptors in the ventromedial prefrontal cortex during contextual fear extinction in rats. Neurobiology of Learning and Memory, 94(3), 318–328. https://doi.org/10.1016/j.nlm.2010.07.004 Escobar, A. P., Wendland, J. R., Chávez, A. E., & Moya, P. R. (2019). The Neuronal Glutamate Transporter EAAT3 in Obsessive-Compulsive Disorder. Frontiers in Pharmacology, 10. https://doi.org/10.3389/fphar.2019.01362 Fullana, M. A., Cardoner, N., Alonso, P., Subirà, M., López-Solà, C., Pujol, J., Segalàs, C., Real, E., Bossa, M., Zacur, E., Martínez-Zalacaín, I., Bulbena, A., Menchón, J. M., Olmos, S., & Soriano-Mas, C. (2014). Brain regions related to fear extinction in obsessive-compulsive disorder and its relation to exposure therapy outcome: A morphometric study. Psychological Medicine, 44(4), 845–856. https://doi.org/10.1017/S0033291713001128 González, L. F., Henríquez-Belmar, F., Delgado-Acevedo, C., Cisternas-Olmedo, M., Arriagada, G., Sotomayor-Zárate, R., Murphy, D. L., & Moya, P. R. (2017). Neurochemical and behavioral characterization of neuronal glutamate transporter EAAT3 heterozygous mice. Biological Research, 50(1), 29. https://doi.org/10.1186/s40659-017-0138-3 Grootheest, D. S. van, Cath, D. C., Beekman, A. T., & Boomsma, D. I. (2005). Twin Studies on Obsessive–Compulsive Disorder: A Review. Twin Research and Human Genetics, 8(5), 450–458. https://doi.org/10.1375/twin.8.5.450 Hajcak, G., & Simons, R. F. (2002). Error-related brain activity in obsessive-compulsive undergraduates. Psychiatry Research, 110(1), 63–72. https://doi.org/10.1016/s0165-1781(02)00034-3 Johannes, S., Wieringa, B. M., Nager, W., Rada, D., Dengler, R., Emrich, H. M., Münte, T. F., & Dietrich, D. E. (2001). Discrepant target detection and action monitoring in obsessive–compulsive disorder. Psychiatry Research: Neuroimaging, 108(2), 101–110. https://doi.org/10.1016/S0925-4927(01)00117-2
18.
19.
20.
21.
22.
23.
24.
25.
26.
27.
28.
29.
30.
31. Lehner, M., Wislowska-Stanek, A., Skorzewska, A., Maciejak, P., Szyndler, J., Turzynska, D., Sobolewska, A., Krzascik, P., & Plaznik, A. (2011). Expression of N-methyl-D-aspartate (R)(GluN2B)—Subunits in the brain structures of rats selected for low and high anxiety. Journal of Physiology and Pharmacology: An Official Journal of the Polish Physiological Society, 62(4), 473–482. Liang, K.-Y., Wang, Y., Shugart, Y. Y., Grados, M., Fyer, A. J., Rauch, S., Murphy, D., McCracken, J., Rasmussen, S., Cullen, B., Hoehn-Saric, R., Greenberg, B., Pinto, A., Knowles, J., Piacentini, J., Pauls, D., Bienvenu, O., Riddle, M., Samuels, J., & Nestadt, G. (2008). Evidence for potential relationship between SLC1A1 and a putative genetic linkage region on chromosome 14q to obsessive-compulsive disorder with compulsive hoarding. American Journal of Medical Genetics. Part B, Neuropsychiatric Genetics: The Official Publication of the International Society of Psychiatric Genetics, 147B(6), 1000–1002. https:// doi.org/10.1002/ajmg.b.30713 Mataix-Cols, D., Rauch, S. L., Baer, L., Eisen, J. L., Shera, D. M., Goodman, W. K., Rasmussen, S. A., & Jenike, M. A. (2002). Symptom Stability in Adult Obsessive-Compulsive Disorder: Data From a Naturalistic Two-Year Follow-Up Study. American Journal of Psychiatry, 159(2), 263–268. https://doi.org/10.1176/appi.ajp.159.2.263 Milad, M. R., Furtak, S. C., Greenberg, J. L., Keshaviah, A., Im, J. J., Falkenstein, M. J., Jenike, M., Rauch, S. L., & Wilhelm, S. (2013). Deficits in Conditioned Fear Extinction in Obsessive-Compulsive Disorder and Neurobiological Changes in the Fear Circuit. JAMA Psychiatry, 70(6), 608–618. https://doi.org/10.1001/jamapsychiatry.2013.914 Milad, M. R., Wright, C. I., Orr, S. P., Pitman, R. K., Quirk, G. J., & Rauch, S. L. (2007). Recall of Fear Extinction in Humans Activates the Ventromedial Prefrontal Cortex and Hippocampus in Concert. Biological Psychiatry, 62(5), 446–454. https:// doi.org/10.1016/j.biopsych.2006.10.011 Paoletti, P., Bellone, C., & Zhou, Q. (2013). NMDA receptor subunit diversity: Impact on receptor properties, synaptic plasticity and disease. Nature Reviews Neuroscience, 14(6), 383–400. https://doi.org/10.1038/nrn3504 Park, S., Lee, S., Kim, J., & Choi, S. (2012). Ex vivo depotentiation of conditioning-induced potentiation at thalamic input synapses onto the lateral amygdala requires GluN2B-containing NMDA receptors. Neuroscience Letters, 530(2), 121–126. https://doi.org/10.1016/j.neulet.2012.10.011 Peghini, P., Janzen, J., & Stoffel, W. (1997). Glutamate transporter EAAC-1-deficient mice develop dicarboxylic aminoaciduria and behavioral abnormalities but no neurodegeneration. The EMBO Journal, 16(13), 3822–3832. https:// doi.org/10.1093/emboj/16.13.3822 Phelps, E. A., Delgado, M. R., Nearing, K. I., & LeDoux, J. E. (2004). Extinction learning in humans: Role of the amygdala and vmPFC. Neuron, 43(6), 897–905. https://doi.org/10.1016/j.neuron.2004.08.042 Pittenger, C. (2015). Glutamatergic agents for OCD and related disorders. Current Treatment Options in Psychiatry, 2(3), 271–283. https://doi.org/10.1007/s40501-015-0051-8 Quinlan, E. M., Olstein, D. H., & Bear, M. F. (1999). Bidirectional, experience-dependent regulation of N-methyl-d-aspartate receptor subunit composition in the rat visual cortex during postnatal development. Proceedings of the National Academy of Sciences, 96(22), 12876–12880. https://doi.org/10.1073/pnas.96.22.12876 Reimer, A. E., de Oliveira, A. R., Diniz, J. B., Hoexter, M. Q., Miguel, E. C., Milad, M. R., & Brandão, M. L. (2018). Fear extinction in an obsessive-compulsive disorder animal model: Influence of sex and estrous cycle. Neuropharmacology, 131, 104–115. https://doi.org/10.1016/j.neuropharm.2017.12.015 Shmelkov, S. V., Hormigo, A., Jing, D., Proenca, C. C., Bath, K. G., Milde, T., Shmelkov, E., Kushner, J. S., Baljevic, M., Dincheva, I., Murphy, A. J., Valenzuela, D. M., Gale, N. W., Yancopoulos, G. D., Ninan, I., Lee, F. S., & Rafii, S. (2010). Slitrk5 deficiency impairs corticostriatal circuitry and leads to obsessive-compulsive-like behaviors in mice. Nature Medicine, 16(5), 598–602, 1p following 602. https://doi.org/10.1038/nm.2125 Shugart, Y. Y., Wang, Y., Samuels, J. F., Grados, M. A., Greenberg, B. D., Knowles, J. A., McCracken, J. T., Rauch, S. L., Murphy, D. L., Rasmussen, S. A., Cullen, B., Hoehn-Saric, R., Pinto, A., Fyer, A. J., Piacentini, J., Pauls, D. L., Bienvenu, O. J., Riddle, M. A., Liang, K. Y., & Nestadt, G. (2009). A family-based association study of the glutamate transporter gene SLC1A1 in obsessive-compulsive disorder in 378 families. American Journal of Medical Genetics. Part B, Neuropsychiatric Genetics: The Official Publication of the International Society of Psychiatric Genetics, 150B(6), 886–892. https://doi.org/10.1002/ ajmg.b.30914 Stewart, S. E., Geller, D. A., Jenike, M., Pauls, D., Shaw, D., Mullin, B., & Faraone, S. V. (2004). Long-term outcome of pediatric obsessive–compulsive disorder: A meta-analysis and qualitative review of the literature. Acta Psychiatrica Scandinavica, 110(1), 4–13. https://doi.org/10.1111/j.1600-0447.2004.00302.x
33.
34.
35. Stewart, S. Evelyn, Fagerness, J. A., Platko, J., Smoller, J. W., Scharf, J. M., Illmann, C., Jenike, E., Chabane, N., Leboyer, M., Delorme, R., Jenike, M. A., & Pauls, D. L. (2007). Association of the SLC1A1 glutamate transporter gene and obsessivecompulsive disorder. American Journal of Medical Genetics Part B: Neuropsychiatric Genetics, 144B(8), 1027–1033. https://doi.org/10.1002/ajmg.b.30533 Ting, J. T., & Feng, G. (2008). Glutamatergic Synaptic Dysfunction and Obsessive-Compulsive Disorder. Current Chemical Genomics, 2, 62–75. https://doi.org/10.2174/1875397300802010062 Welch, J. M., Lu, J., Rodriguiz, R. M., Trotta, N. C., Peca, J., Ding, J.-D., Feliciano, C., Chen, M., Adams, J. P., Luo, J., Dudek, S. M., Weinberg, R. J., Calakos, N., Wetsel, W. C., & Feng, G. (2007). Cortico-striatal synaptic defects and OCD-like behaviours in Sapap3 -mutant mice. Nature, 448(7156), 894–900. https://doi.org/10.1038/nature06104 Zike, I. D., Chohan, M. O., Kopelman, J. M., Krasnow, E. N., Flicker, D., Nautiyal, K. M., Bubser, M., Kellendonk, C., Jones, C. K., Stanwood, G., Tanaka, K. F., Moore, H., Ahmari, S. E., & Veenstra-VanderWeele, J. (2017). OCD candidate gene SLC1A1/ EAAT3 impacts basal ganglia-mediated activity and stereotypic behavior. Proceedings of the National Academy of Sciences, 114(22), 5719–5724. https://doi.org/10.1073/pnas.1701736114