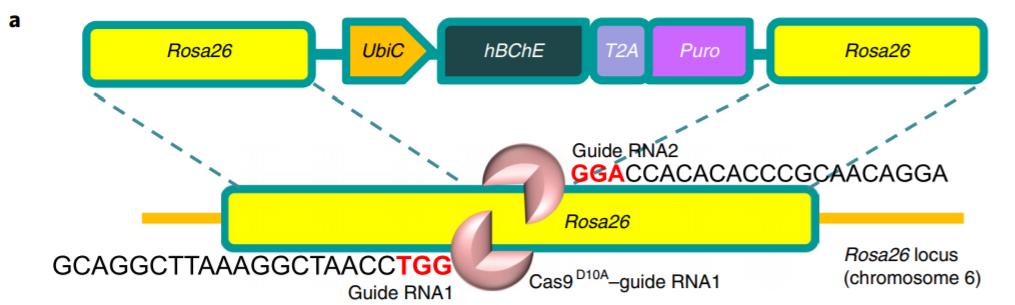
14 minute read
Genome edited Skin Transplants Offer a Safe and Enduring Gene Therapy Approach for Treating Drug Addiction
John Luu
Canonical treatments of cocaine dependence are primarily behavioural therapy-based as there are no approved drugs or gene-based therapies for treatment (Fischer et al. 2015). Human butyrylcholinesterase (hBChE) has been engineered for extremely high specificity and catalytic potential for hydrolysis of cocaine (Zheng et al. 2014), but prior delivery strategies using fusion proteins and viral vectors have produced insubstantial results or are prone to high expenses and risk for therapeutic application (Gilgun-Sherki et al. 2016; Naldini 2015). Li and colleagues (2019) demonstrate the viability of editing epidermal stem cells for expression of engineered hBChE and grafting edited tissues into mice for protection against cocaine-induced toxicity and behaviour. Mice with hBChE-releasing skin grafts exhibited protection against cocaine overdose as well as significant reductions in cocaine seeking, hyperactivity, and relapse. The results support a powerful gene therapy approach for expression of therapeutic proteins that circumvents the primary obstacle of the hBChE enzyme’s short half-life (Brimijoin 2011) by enabling continued expression long-term. However, the authors did not address the impact on cocaine relapse induced by environmental cues—powerful conditioned stimuli primarily responsible for chronic relapse behaviour (Lee, Milton, and Everitt 2006); the authors may employ a conflict model of cue-induced relapse to test this (Katzir et al. 2007).
Advertisement
BACKGROUND
Cocaine addiction initiates from overstimulation of reward pathways of the brain—specifically via ventral tegmental area (VTA) dopaminergic signaling to the nucleus accumbens and prefrontal cortex (Penberthy et al. 2010). Ensuing drug dependence is characterized by cue-induced cravings and hedonic homeostatic dysregulation which underlie compulsive drug seeking, abuse, and relapse behaviours despite socioeconomic, psychological, and physical burdens (Dackis and O’Brien 2001). Typical treatment approaches involve behavioural interventions which include cognitive behavioural therapy (CBT)—an individualized method teaching patients new mental and behavioural tools to cope with drug-related stress—and contingency management (CM)—providing reward incentives based on the principle of operant conditioning (Penberthy et al. 2010). There currently exists no approved pharmaceutical interventions and the collective literature on pharmacological and immune-based therapies remains unconvincing (Fischer et al. 2015). One potential therapeutic protein for treating cocaine abuse is human butyrylcholinesterase (BChE), an enzyme that endogenously functions to detoxify environmental toxicants— including hydrolysis of cocaine into inactive products—and in hydrolysis of acetylcholine (ACh) neurotransmitter (Lockridge 2015). Natural plasma BChE is limited in its therapeutic application by very low efficiency for hydrolysing cocaine, thus modified versions of human BChE (hBChE) have been engineered for drastically greater catalytic efficiency and greater specificity for cocaine (Zheng et al. 2014). A short half-life compared to wildtype hBChE and potentially inefficient parenteral delivery are the primary obstacles to therapeutic application of engineered hBChE (Brimijoin 2011). Past attempts at delivering recombinant hBChE via albumin-hBChE fusion proteins delivered intramuscularly failed to attenuate cocaine dependence in human participants (Gilgun-Sherki et al. 2016). Viral-mediated delivery methods are also plagued by challenges of precision cell targeting, efficient gene transduction, and avoidance of host immunity (Naldini 2015). In their 2019 publication, Li and colleagues demonstrate a delivery approach based on a 2017 study by Yue et. al utilizing epidermal stem cells edited ex vivo to express engineered hBChE which were grafted into a host for long-term protein delivery. A gene therapy approach based on skin benefits from great accessible of the organ enabling ease of extraction, monitoring, and removal. Furthermore, well-established protocols for growing skin implants in vitro for treating burns exist (O’Connor et al. 1981). Epidermal keratinocytes are also excellent candidates for transplantation due to low immunogenicity offered by the absence of MHC class II expression (Haniffa, Gunawan, and Jardine 2015). Previous experiments have proven successful in engineering skin cells for secretion of large proteins into circulation for therapeutic effect (Fakharzadeh et al. 2000). The authors successfully demonstrated cocaine clearance in mice grafted with engineered skin implants, in addition to attenuation of cocaine seeking, relapse, and acute systemic toxicity (Li et al. 2019).
MAJOR RESULTS CRISPR-mediated expression of hBChE in mice epidermal stem cells
Li and colleagues (2019) performed genome editing via clustered regularly interspaced short palindromic repeats (CRISPR) using vectors encoding Cas9 D10A nickase—functioning in pairs for double-stranded DNA cleavage (Ran et al. 2013). Additional vectors encoded two guide RNAs targeting the Rosa26 locus for ubiquitous expression (Chu et al. 2016). The targeting vector itself contained the expression cassette for homology directed repair (HDR)-mediated insertion of hBChE—encoding the enzyme hydrolyzing cocaine—and Puro—encoding puromycin resistance for selection (Fig. 1). Constitutive expression of cassette genes is driven by UbiC ubiquitin promoter. CRISPR elements were transformed into epidermal basal cells of newborn mice via electrophoresis.
Figure 1 Targeting vector containing expression cassette for CRISPR-mediated expression of engineered hBChE for cocaine hydrolysis. Figure adapted from: Li et al. (2019) Quantitative validation of hBChE secretion from genome-edited and wild-type epidermal progenitor cells via enzyme-linked immunosorbent assay (ELISA) revealed strong, detectable expression in genome-edited cells only (Fig. 2A). Additionally, the cocaine hydrolysis activity of secretions from cell cultures expressing hBChE and similarly engineered mouse BChE (mBChE) in comparison with wild-type control cultures was determined via clearance assay; only hBChE cultures showed significant clearance of cocaine (Fig. 2B). Thus, the engineered epidermal progenitors were successfully edited for cocaine degrading activity in vivo.
(A) (B)
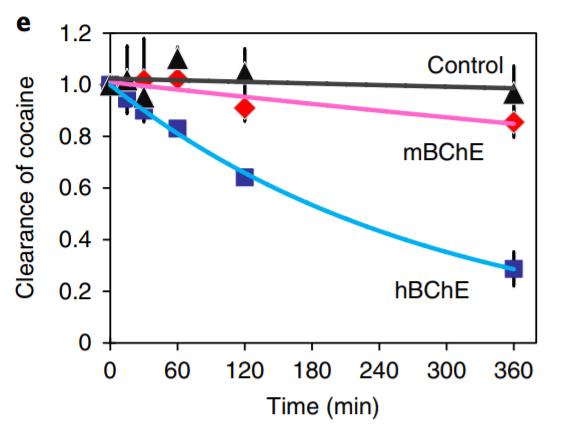
Figure 2 (A) Detection of serum hBChE levels via ELISA in control and hBChE-expressing mice. Detectable levels are only present in hBChE-expressing mice. (B) Cocaine clearance assay for control, mBChE-expressing mice, and hBChE-expressing mice. Significant clearance only seen for hBChE-expressing mice. Figure adapted from: Li et al. (2019).
Wild-type mice were grafted with skin organoids generated associated CPP acquisition only seen in GWT mice. (B) CPP
from in vivo differentiation and stratification of epidermal stem extinction in GWT and GhBChE mice. Cocaine associated
cells engineered to express hBChE (GhBChE mice) and compared to controls grafted with unedited skin organoids (GWT mice) (Li et al. 2019). ELISA-mediated detection of blood hBChE levels over a 10-week period revealed significant levels in GhBChE mice only. Extracellular cocaine and associated dopamine levels within the nucleus accumbens—involved in the reward pathway of the brain—from microdialysis collections revealed significant reductions in both cocaine and dopamine levels after cocaine injection in GhBChE mice when compared to GWT mice. Elevated locomotion induced by cocaine injection was attenuated in hBChE-producing mice compared to controls (Fig. 3A). GhBChE mice were also protected from cocaineinduced acute toxicity at cocaine levels that proved lethal in GWT mice (Fig. 3B).
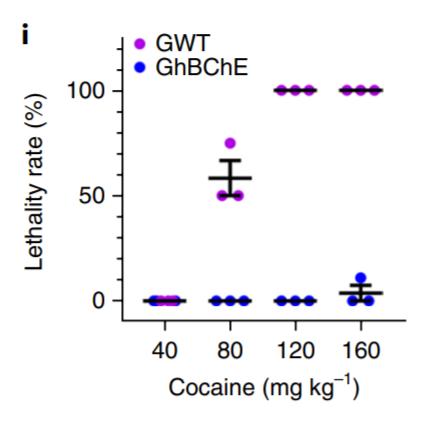
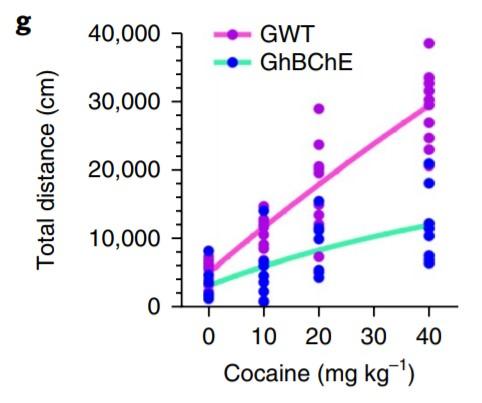
(A) (B) DISCUSSION
Li et al. (2019) successfully used CRISPR to edit mouse epidermal stem cells for expression of engineered hBChE for cocaine hydrolysis. Transplantation of hBChE-expressing skin organoids, originating from mice or humans, into mice demonstrated cocaine clearance after drug administration as well as protection from cocaine-induced acute systemic toxicity, or overdose. livery first presented by Yue et al. in 2017. This is also the first application of the approach for treating the effects of addictive
Figure 3
(A) GhBChE mice show reduced locomotion at all cocaine dosages compared to GWT mice. (B) GhBChE mice rescued from cocaine-induced lethality at dosages lethal to GWT mice. Figure adapted from: Li et al. (2019).
Transplantation of hBChE-expressing skin organoid diminishes
A conditioned place preference (CPP) paradigm was used to evaluate cocaine-seeking and relapse behaviour (Li et al. 2019). CPP acquisition following cocaine conditioning was only evident in GWT mice and not GhBChE mice (Fig. 4A). CPP re-acquisition following skin grafting and a CPP extinction period revealed reinstatement of CPP in GWT mice only, despite identical pregrafting CPP acquisition and post-grafting CPP extinction patterns between mice groups (Fig. 4B). Ethanol conditioning was used as a control, with no differences in CPP acquisition or reinstatement between mice groups.
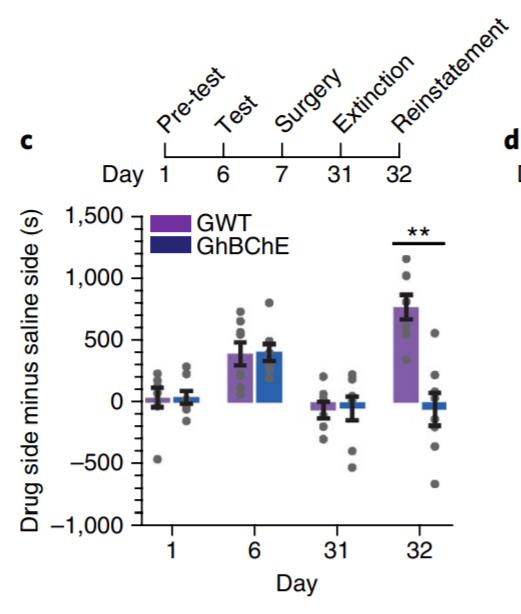
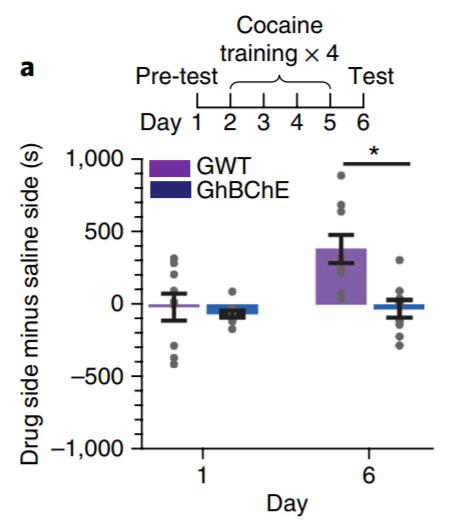
(A)
Figure 4 (A) Conditioned place preference (CPP) paradigm for cocaine conditioning in GWT and GhBChE mice. Cocaine paradigm for cocaine preference reinstatement following CPP reinstatement only seen in GWT mice; identical CPP acquisition between mice groups pre-surgery for skin grafting. Figure adapted from: Li et al. (2019).
CRISPR-mediated hBChE expression in human epidermal stem cells
The authors used a similar CRISPR approach as shown in Figure 1a for genome-editing of human epidermal keratinocytes from newborn foreskin for hBChE expression (Li et al. 2019). Significant hBChE secretions in the blood of nude mice grafted with hBChE-expressing human skin organoid was validated via ELISA in comparison to control mice grafted with unedited cells. Thus, CRISPR-edited human skin cells are a viable medium for delivering hBChE for treatment of cocaine addiction.
Most notably hBChE-expression in mice attenuated cocaineinduced behaviours including hyperactivity, cocaine seeking, and relapse. Thus, the experimental results further support the viability of cutaneous gene therapy for therapeutic protein de
cocaine-seeking and relapse in mice
stimulants affecting behaviour. GhBChE mice maintained stable hBChE levels in serum for more than 10 weeks, with the oldest mice living up to 6 months without signs of immune rejection (Li et al. 2019). The authors also reference the historical use of autologous skin grafts for the treatment of severe burns and skin diseases, including those generated from genome-edited stem cell cultures, as evidence for safe and long-lasting skin-based gene therapy. The therapeutic advantages of their cutaneous gene therapy approach are highlighted in the key cocaine-related toxicity and behavioural issues protected against by hBChE-producing skin grafts in mice. Therapeutic application is also made easier by extremely high specificity and catalytic activity for cocaine alleviating the need for individualization based on cocaine dosage. The cutaneous genome-edited transplantation strategy can be applied for treating abuse beyond cocaine as demonstrated by Yue et al. (2017) when they CRISPR-engineered mouse and human epidermal stem cells for secretion of glucagon-like peptide 1 (GLP1) for protection against obesity and diabetes induced by high-fat consumption.
CRITICAL ANALYSIS
A major challenge to overcoming cocaine and general drug dependence is recurrent relapse, despite long periods of abstinence, primarily mediated by drug-associated cues (Gawin and Kleber 1986). These cues function like conditioned environmental stimuli in a Pavlovian classical conditioning paradigm and thus drug-seeking and relapse responses are at least, in part, driven by involuntary psychological drives explaining drug abuse despite negative outcomes (Di Ciano and Everitt 2004). As such, it is worth investigating the effectiveness of hBChEexpressing skin implants for attenuation of cue-induced relapse behaviour. It is worth addressing if there is a cocaine dosage threshold for which the protective qualities of hBChE secretions are diminished. Cocaine esterase (COcE)—another cocaine hydrolysing enzyme derived from bacteria—failed to attenuate cocainereinforced responses at sufficiently high doses (Collins et al. 2009). However, this may be a non-issue as experimental mice have been shown to tolerate BChE dosages at 1500-fold greater than endogenous levels (Murthy et al. 2014), and thus it may be reasonable to just increase hBChE dosages to levels sufficient to combat high cocaine administration.
FUTURE DIRECTIONS
To evaluate the capacity of mice grafted with hBChE-expressing skin transplants to resist cue-triggered cocaine relapse, the authors should adapt the conflict model of cue-induced relapse outlined by Katzir and colleagues (2007). This paradigm better reflects the human experience of self-conflict between the impulsions to use a drug and the desire to avoid drug-associated negative outcomes. For this procedure, mice are conditioned to press a lever paired with a light-cue to receive cocaine after which an electrified barrier on the floor grows in intensity until animals avoid the lever for a few days straight. The following testing period involves random light-cue presentations with the active electrified barrier and lever presses are recorded. When testing mice grafted with unedited skin (GWT mice) and mice grafted with hBChE-expressing skin (GhBChE mice), it expected that more GhBChE mice will relapse in response to the lone light-cue compared to GWT mice by merit of hBChE-mediated clearance of cocaine drastically reducing hedonistic response to the drug in the first place. However, performing grafting surgery after cue-conditioning may show no differences between mice groups because the brain systems underlying contextual learning and cued learning are not identical. For instance, the hippocampus is necessary for contextual learning, which was studied when the authors used a CPP paradigm, but it is not necessary for associative learning of cues (Curzon, Rustay, and Browman 2009); the hippocampus should be unaffected by hBChE-expression post-cue conditioning.
10.
11.
12.
13.
14. Brimijoin, Stephen. 2011. “Interception of Cocaine by Enzyme or Antibody Delivered with Viral Gene Transfer: A Novel Strategy for Preventing Relapse in Recovering Drug Users.” CNS & Neurological Disorders Drug Targets 10 (8): 880–91. Chu, Van Trung, Timm Weber, Robin Graf, Thomas Sommermann, Kerstin Petsch, Ulrike Sack, Pavel Volchkov, Klaus Rajewsky, and Ralf Kühn. 2016. “Efficient Generation of Rosa26 Knock-in Mice Using CRISPR/Cas9 in C57BL/6 Zygotes.” BMC Biotechnology 16 (1): 4. https://doi.org/10.1186/s12896-016-0234-4. Collins, Gregory T., Remy L. Brim, Diwahar Narasimhan, Mei-Chuan Ko, Roger K. Sunahara, Chang-Guo Zhan, and James H. Woods. 2009. “Cocaine Esterase Prevents Cocaine-Induced Toxicity and the Ongoing Intravenous Self-Administration of Cocaine in Rats.” The Journal of Pharmacology and Experimental Therapeutics jpet.108.150029. 331 (2): 445–55. https://doi.org/10.1124/
Curzon, Peter, Nathan R. Rustay, and Kaitlin E. Browman. 2009. “Cued and Contextual Fear Conditioning for Rodents.” In Methods of Behavior Analysis in Neuroscience, edited by Jerry J. Buccafusco, 2nd ed. Frontiers in Neuroscience. Boca Raton (FL): CRC Press/Taylor & Francis. http://www.ncbi.nlm.nih.gov/books/NBK5223/. Dackis, Charles A., and Charles P. O’Brien. 2001. “Cocaine Dependence: A Disease of the Brain’s Reward Centers.” Journal of Substance Abuse Treatment 21 (3): 111–17. https://doi.org/10.1016/S0740-5472(01)00192-1. Di Ciano, Patricia, and Barry J. Everitt. 2004. “Conditioned Reinforcing Properties of Stimuli Paired with Self-Administered Cocaine, Heroin or Sucrose: Implications for the Persistence of Addictive Behaviour.” Neuropharmacology 47 Suppl 1: 202–13. https://doi.org/10.1016/j.neuropharm.2004.06.005. Fakharzadeh, S. S., Y. Zhang, R. Sarkar, and H. H. Kazazian. 2000. “Correction of the Coagulation Defect in Hemophilia A Mice through Factor VIII Expression in Skin.” Blood 95 (9): 2799–2805. Fischer, Benedikt, Peter Blanken, Dartiu Da Silveira, Andrea Gallassi, Elliot M. Goldner, Jürgen Rehm, Mark Tyndall, and Evan Wood. 2015. “Effectiveness of Secondary Prevention and Treatment Interventions for Crack-Cocaine Abuse: A Comprehensive Narrative Overview of English-Language Studies.” The International Journal on Drug Policy 26 (4): 352–63. https:// doi.org/10.1016/j.drugpo.2015.01.002. Gawin, F. H., and H. D. Kleber. 1986. “Abstinence Symptomatology and Psychiatric Diagnosis in Cocaine Abusers. Clinical Observations.” Archives of General Psychiatry 43 (2): 107–13. https://doi.org/10.1001/archpsyc.1986.01800020013003. Gilgun-Sherki, Yossi, Rom E. Eliaz, David J. McCann, Pippa S. Loupe, Eli Eyal, Kathleen Blatt, Orit Cohen-Barak, Hussein Hallak, Nora Chiang, and Shwe Gyaw. 2016. “Placebo-Controlled Evaluation of a Bioengineered, Cocaine-Metabolizing Fusion Protein, TV-1380 (AlbuBChE), in the Treatment of Cocaine Dependence.” Drug and Alcohol Dependence 166 (September): 13–20. https://doi.org/10.1016/j.drugalcdep.2016.05.019. Haniffa, Muzlifah, Merry Gunawan, and Laura Jardine. 2015. “Human Skin Dendritic Cells in Health and Disease.” Journal of Dermatological Science 77 (2): 85–92. https://doi.org/10.1016/j.jdermsci.2014.08.012. Katzir, Ayelet, Noam Barnea-Ygael, Dino Levy, Yavin Shaham, and Abraham Zangen. 2007. “A Conflict Rat Model of CueInduced Relapse to Cocaine Seeking.” Psychopharmacology 194 (1): 117–25. https://doi.org/10.1007/s00213-007-0827-7. Lee, Jonathan L. C., Amy L. Milton, and Barry J. Everitt. 2006. “Cue-Induced Cocaine Seeking and Relapse Are Reduced by Disruption of Drug Memory Reconsolidation.” The Journal of Neuroscience 26 (22): 5881–87. https://doi.org/10.1523/
JNEUROSCI.0323-06.2006.
Li, Yuanyuan, Qingyao Kong, Jiping Yue, Xuewen Gou, Ming Xu, and Xiaoyang Wu. 2019. “Genome-Edited Skin Epidermal Stem Cells Protect Mice from Cocaine-Seeking Behaviour and Cocaine Overdose.” Nature Biomedical Engineering 3 (2): 105–13. https://doi.org/10.1038/s41551-018-0293-z.
16.
17.
18.
19.
20.
21.
22. Lockridge, Oksana. 2015. “Review of Human Butyrylcholinesterase Structure, Function, Genetic Variants, History of Use in the Clinic, and Potential Therapeutic Uses.” Pharmacology & Therapeutics 148 (April): 34–46. https://doi.org/10.1016/
j.pharmthera.2014.11.011. Murthy, Vishakantha, Yang Gao, Liyi Geng, Nathan K. LeBrasseur, Thomas A. White, Robin J. Parks, and Stephen Brimijoin. 2014. “Physiologic and Metabolic Safety of Butyrylcholinesterase Gene Therapy in Mice.” Vaccine 32 (33): 4155–62. https:// doi.org/10.1016/j.vaccine.2014.05.067. Naldini, Luigi. 2015. nature15818. “Gene Therapy Returns to Centre Stage.” Nature 526 (7573): 351–60. https://doi.org/10.1038/
O’Connor, NicholasE., JohnB. Mulliken, Susan Banks-Schlegel, Olaniyi Kehinde, and Howard Green. 1981. “GRAFTING OF BURNS WITH CULTURED EPITHELIUM PREPARED FROM AUTOLOGOUS EPIDERMAL CELLS.” The Lancet, Originally published as Volume 1, Issue 8211, 317 (8211): 75–78. https://doi.org/10.1016/S0140-6736(81)90006-4. Penberthy, Jennifer K., Nassima Ait-Daoud, Michelle Vaughan, and Tasmin Fanning. 2010. “Review of Treatment for Cocaine Dependence.” Current Drug Abuse Reviews 3 (1): 49–62. https://doi.org/10.2174/1874473711003010049. Ran, F. Ann, Patrick D. Hsu, Chie-Yu Lin, Jonathan S. Gootenberg, Silvana Konermann, Alexandro Trevino, David A. Scott, et al. 2013. “Double Nicking by RNA-Guided CRISPR Cas9 for Enhanced Genome Editing Specificity.” Cell 154 (6): 1380–89. https://doi.org/10.1016/j.cell.2013.08.021. Yue, Jiping, Xuewen Gou, Cynthia Li, Barton Wicksteed, and Xiaoyang Wu. 2017. “Engineered Epidermal Progenitor Cells Can Correct Diet-Induced Obesity and Diabetes.” Cell Stem Cell 21 (2): 256-263.e4. https://doi.org/10.1016/j.stem.2017.06.016. Zheng, Fang, Liu Xue, Shurong Hou, Junjun Liu, Max Zhan, Wenchao Yang, and Chang-Guo Zhan. 2014. “A Highly Efficient Cocaine-Detoxifying Enzyme Obtained by Computational Design.” Nature Communications 5 (1): 3457. https://
doi.org/10.1038/ncomms4457.