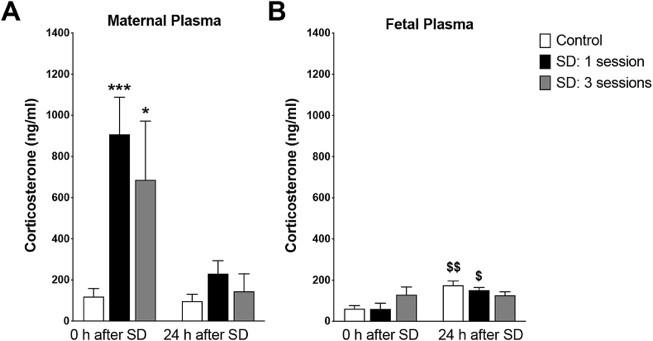
18 minute read
The Molecular Mechanisms Underlying Sleep Deprivation and Impaired Fetal Neurodevelopment
Pearse O’Malley
Sleep deprivation (SD) has been implicated in several short-term and long-term cognitive deficits in organisms. While most research has focused on adult organisms, there is increasing concern surrounding the effects of pregnant individuals’ SD on the neurodevelopment of their fetus. A relationship between sleep, stress, and the immune system has previously been established, but the role of SD in causing inflammation was unclear. Baratta et al. (2020) examined the molecular pathways affected by maternal SD and the consequences for the fetus by inducing SD in pregnant mice and obtaining tissue and plasma samples from the mother and fetus. Corticosterone and kynurenic acid (KYNA) were used as markers of stress and impaired metabolism due to SD, respectively, with greater corticosterone levels in the sleep-deprived mice that did not recover from SD and greater KYNA accumulation, correlating to increased KYNA accumulation in the fetal brain. The results indicate that the stress response elicited from maternal SD is related to fetal brain KYNA accumulation, which is associated with weakened memory and learning abilities in the offspring, thus highlighting the dangerous effects of maternal SD on fetal neurodevelopment.
Advertisement
Key words: Sleep deprivation (SD), kynurenine pathway (KP), maternal stress, corticosterone, pregnancy
BACKGROUND AND INTRODUCTION
Sleep deprivation (SD), or experiencing consistently inadequate sleep, has long been associated with cognitive and physiological dysfunction in organisms. While previous studies have demonstrated the observable effects of SD on adults—such as increased risky decision-making and decreased memory during recall tasks (Chen et al., 2017)—less is known regarding the increase in maternal corticosterone serum levels measured in the rats, with the rats whose plasma samples were taken im
molecular mechanisms through which hormone changes in pregnant individuals affect the developing fetus. Peng et al. (2016) established that SD in pregnant rats contributed to increased susceptibility of sleep disorders in the mothers, but noted that the biochemical pathways underlying the offspring’s subsequent cognitive impairment were unclear.
An association between SD in pregnant individuals and their serum pro-inflammatory cytokine levels was established by Okun & Coussons-Read (2006), who also suggest that the lack of sleep these individuals experience may contribute to increased cytokine levels and systemic inflammation. Nevertheless, the authors state that future research should consider how these elevated serum cytokine levels could affect the fetus, as their study focused only on the maternal serum levels (Okun & Coussons-Read, 2006). SD has further been known to induce a stress response in sleep-deprived individuals, which can be monitored by the serum corticosterone levels (WynneEdwards, Edwards, & Hancock, 2013). Lastly, kynurenine—a metabolite derived from the breakdown of tryptophan, an amino acid obtained from several foods—has been implicated in SD, but its role in inducing cognitive impairments, in the mothaccumulation in the fetal brain, leading to impaired learning and memory (Baratta et al., 2020). Together, these results corroborate the role of maternal SD in impaired fetal neurodevelopment.
MAJOR RESULTS Maternal corticosterone levels increase in sleep-deprived mothers
Corticosterone, a hormone involved in the stress and immune responses, was obtained from the maternal and fetal plasma and used as a marker in examining the adverse effects of SD. SD led to stress in the pregnant rats, as evidenced by the drastic er and fetus, had yet to be determined (Sforzini, 2019).
mediately after one or three SD sessions having the highest serum corticosterone (Baratta et al., 2020). The rats who were able to recover following SD had corticosterone levels that were comparable to the control rats that did not experience SD (Fig 1), indicating that the spike in serum corticosterone due to SD is a short-term consequence (Baratta et al., 2020). This result is consistent with other studies examining the effects of SD -induced stress, such as that of Ito et al. (2014), who also used serum corticosterone levels as a metric of stress in murine models. While an increase in maternal corticosterone plasma due to SD was observed, fetal corticosterone plasma did not seem to be greatly affected by SD (Baratta et al., 2020).
With the detrimental impacts that SD can have on individuals, a better understanding of the compromised molecular pathways is crucial to devise therapeutics for pregnant individuals experiencing SD and their newborns, or to advocate for prevention against SD in a public health context. Baratta et al. (2020) sought to elucidate how maternal SD was associated with fetal cognitive impairment, primarily through the kynurenine pathway (KP) through which tryptophan is degraded and did so by inducing SD in pregnant Wistar rats. Following zero, one, or three five-hour SD sessions where the rats' sleep is disturbed every 30 minutes, maternal and fetal plasma, brain, and placental tissue samples were obtained and examined for kynurenic acid (KYNA) accumulation, pro-inflammatory cytokine levels to understand the relationship between sleep and the immune system, and corticosterone levels as a measurement of the stress response in sleep-deprived mice (Baratta et al., 2020). Tissue samples of some mice were either taken immediately after the SD session, or 24 hours after the SD session, during which the mice were allowed to recover with uninterrupted sleep, resulting in a total of six treatment groups (Baratta et al., 2020). Analysis of the blood plasma and tissues indicated a greater stress response in the sleep-deprived mice with no recovery compared to the mice with recovery and control mice, which seemed to be associated with increased KYNA
Figure 1. Increased maternal serum corticosterone in sleepdeprived rats without recovery; SD treatment did not significantly affect fetal serum corticosterone. Figure adapted from Baratta et al. (2020)
Role of SD in KP and KYNA accumulation
Tryptophan is obtained from the diet and metabolized to kynurenine in the KY by tryptophan-2,3-dioxygenase, but a buildup of kynurenine from excess tryptophan leads to KYNA accumulation in the serum and brain (Baratta et al., 2020). Serum tryptophan levels are known to increase during SD (Davies et al., 2014), which is why the increased maternal tryptophan serum levels led to greatly increased fetal brain KYNA levels immediately following one session of sleep deprivation (Fig 2) (Baratta et al., 2020). Placental tissue analysis did not provide evidence of increased placental kynurenine or KYNA accumulation, but of most salience is the fetal brain KYNA, which is associated with impaired neurogenesis and decreased cognitive performance (Rudzki et al., 2019).
ment of fetuses following maternal SD, a molecule whose function in irregular fetal development had not previously been clear (Baratta et al., 2020). The authors do not implicate increased maternal corticosterone as a direct correlate to greater kynurenine metabolism and KYNA accumulation, but rather as a correlate to increased proinflammatory cytokine levels (Baratta et al., 2020). This aligns study by Baratta et al. (2020) is novel in its focus on the adverse effects that KYNA accumulation has on the fetus rather than the
Figure 2. Maternal brain KYNA levels are relatively unaffected by SD, while SD led to a drastic increase in fetal brain KYNA levels after one SD session, regardless of recovery time. Figure adapted from Baratta et al. (2020)
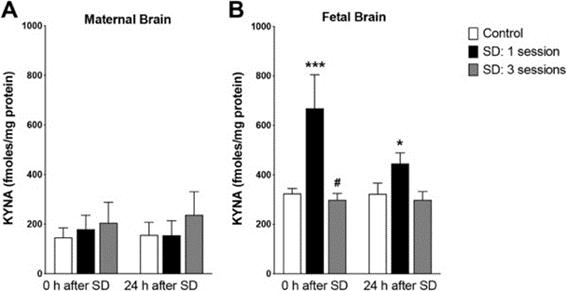
SD increases pro-inflammatory cytokine levels in the fetal and maternal brain
hypothesized, as this elevated immune response is associated with tryptophan breakdown through the KP (Baratta et al., 2020). Therefore, with this immune response elicited by SD, it was assumed that cytokine levels would increase in the sleepdeprived rats compared to the control, consequently increasing KYNA as a by-product of the KP. Placental tissue analysis showed increased IL-1β and IL-6 following SD, with recovery time and sleep condition having no main individual effects (Baratta et al., 2020). Fetal brain tissue analysis also showed a great increase in IL-1β and IL-6, with recovery time having no clear effect (Fig 3) (Baratta et al., 2020).
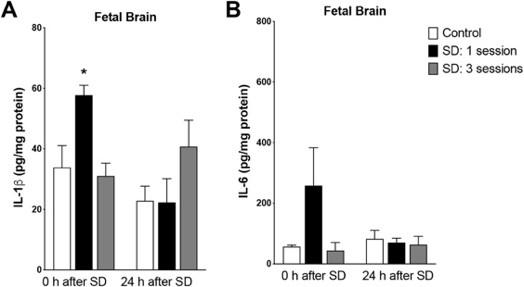
with other studies which assert that there is increased tryptophan degradation following activation of the immune system (Farhangi et al., 2018; Maes et al., 2002). Unlike other studies focusing on the KP and its effects on cognition, however, the An increase in pro-inflammatory cytokines following SD was
sleep-deprived organism itself.
KYNA accumulation due to SD is of particular concern, given the various consequences it has in individuals. KYNA has been identified as an antagonist to NMDA receptors, which are involved in synaptic plasticity and memory (Plitman et al., 2017); with an excess of KYNA in the brain—as seen in the fetuses of sleepdeprived mothers—glutamate, an important excitatory neurotransmitter, cannot bind to the NMDA receptors (Dornbierer et al., 2019). In addition to impaired memory and learning, the inactivation of the NMDA receptors is related to schizophrenia, so the identification of KYNA as an aggregate in the fetal brain may thus make these newborns more susceptible to these neuropsychiatric disorders (Koola et al., 2018; Wichers et al., 2005). This information could be used in researching therapeutics that degrade or block excess KYNA in the fetal brain from acting as an NMDA receptor antagonist and inducing these adverse effects (Koola et al., 2018).
CRITICAL ANALYSIS
Although the study by Baratta et al. (2020) is novel in examining pregnant model organisms, the study did not focus on the stage of pregnancy at which the mice were presently—all samples es of maternal SD for the fetus will vary by the extent of their development. For example, the effects of SD on the fetuses of
Figure 3. Fetal brain tissue analysis showed increased IL-1β and IL-6 following SD; recovery time and sleep condition had no main individual effect. Figure adapted from Baratta et al. (2020)
CONCLUSION/DISCUSSION
The results of this study indicate a positive association between maternal corticosterone and fetal brain KYNA levels, such that increased maternal serum corticosterone correlates to increased KYNA accumulation in the fetal brain (Baratta et al., 2020). Additionally, maternal corticosterone appears to be posiwere higher when maternal serum corticosterone was higher (Baratta et al., 2020). These relationships substantiate the authors’ hypothesis that the maternal stress and immune responses caused detrimental effects in the fetus, thus highlighting KYNA as a key mediator in the impaired neurodevelop
were taken for analysis on either the 18 th embryonic day (ED) or 19 th ED, or the duration of the rats’ pregnancy up to that point (Baratta et al., 2020). However, the severity of the consequenctively associated with increased placental IL-1β, as IL-1β levels
pregnant mice in their first trimester would likely be more severe than those in their third trimester (Lees et al., 2015), as the central nervous system (CNS) largely develops in the early embryonic stages of pregnancy and could be hindered by KYNA accumulation (Salihagic-Kadic et al., 2005).
The three treatment groups the authors had for each recovery time of zero or 24 hours following SD was used to distinguish between the effects of SD on rats that had not been sleep deprived (control), had been sleep deprived for a short amount of time (one SD session), and those that had consistently been sleep deprived (three SD sessions) (Baratta et al., 2020). How- 117
ever, three SD sessions may not be sufficient to represent consistent SD, as another study examining the effects of SD on memory formation used a range of one to seven nights of SD in subjects as a treatment (Lo et al., 2016). Additionally, a 24-hour recovery after SD may also be insufficient to represent the lasting effects of maternal SD on fetuses, despite the short gestation period in mice of 18-22 days (Murray et al., 2010). Given that Baratta et al. (2020) took the plasma and tissue samples only one day following the SD, they were unable to determine if the KYNA accumulation persisted. Therefore, a treatment with many more than three SD sessions and taking samples after the 24-hour recovery period may be required to examine the longterm effects of SD on both the mother and fetus, especially given that Baratta et al. (2020) did not find that the mice’s sleep condition independently had a significant effect on corticosterone, pro-inflammatory cytokine, or KYNA levels (Baratta et al., 2020). This study’s results would likely show that prowithout recovery after SD, Davies et al. (2014) found that plasma levels of metabolites, including tryptophan, remained high
inflammatory cytokine levels in the mice with recovery was similar to the control mice (Toulmonde et al., 2018), while the mice without recovery had significantly higher proinflammatory cytokine levels (Baratta et al., 2020). The lack of KYNA accumulation later in development may suggest that its effects on fetal neurodevelopment are not as severe as purported to be. Pregnant individuals who are consistently sleep deprived during their pregnancy may be so for multiple days or weeks, representing more sleep conditions in this study, which may have more severe effects on their fetus.
FUTURE DIRECTIONS
Future experiments should focus on understanding the effect, if any, of SD on rats at different stages of their pregnancy. As mentioned, SD could be more detrimental to organisms and their offspring earlier on in their pregnancy, as this is primarily when fetal neurodevelopment is occurring (Salihagic-Kadic et al., 2005). Similar to the original study, the proposed study must have a control and two other sleep conditions, with all three groups containing mice at the same stage of pregnancy; these conditions would allow the researchers to examine SD effects at multiple stages of pregnancy if there were three groups of mice for each trimester of pregnancy. It is anticipated that tissue analysis would show similar levels of KYNA accumulation across groups, but an underdeveloped CNS and lack of neurogenesis in the fetuses’ brains of mothers who were in their first trimester of pregnancy (Rajendiran et al., 2015). These results would indicate that maternal SD is more detrimental if it occurs earlier in the mother’s pregnancy. If the impaired neurodevelopment appears to be consistent across groups, then it is likely that the effects of SD are unrelated to ples could be taken from an additional group of mice who experience more than five SD sessions, as this will allow for comparison between groups on the basis of sleep condition (Khalyfa et al., 2015). Baratta et al. (2020) found that tissues in the group that underwent three SD sessions had comparable proinflammatory cytokine and KYNA levels, and lower levels than the mice that underwent one SD session, regardless of recovery, implying a compensatory effect in these mice with additional SD to maintain homeostatic cytokine and KYNA levels. Nevertheless, Rosansky et al. (1996) determined that SD does not lead to the body physiologically compensating for the observed decrease in blood pressure, so having more SD conditions may dispute that the results observed by Baratta et al. (2020) are due to compensation and instead due to a presently unknown molecular mechanism.
Finally, examining the long-term effects of maternal SD on the fetus is possible by performing the tissue and plasma collection and analysis more than 24 hours after recovery. If collected later into the mother’s pregnancy, lasting effects would be observed and may show that KYNA accumulation persists despite recovery, as another study examining embryonic exposure to nicotine demonstrated that nicotine-induced disruption to sleep homeostasis persisted in the offspring into adulthood (Borniger et al., 2017). While Baratta et al. (2020) found KYNA levels were comparable across groups for the mice with and the stage at which an organism is pregnant.
48 hours following SD. With more tryptophan, more KYNA accumulation will occur, potentially leading to further impaired fetal brain neurodevelopment, as Vohra et al. (2018) show that depleting KYNA in the brains of the Caenorhabditis elegans model improves memory and learning ability indicating KYNA’s role in impaired neurogenesis. If there is no clear disparity between mice that have more time to recover and those that do not, this would suggest that SD may not have the long-term fetal consequences that it is speculated to have. Regardless, this information is essential to establish public health policies and advocate against SD in pregnant individuals to offset its adverse fetal effects.
Given that the sleep conditions used by Baratta et al. (2020) did not have a significant main effect by themselves on KYNA accumulation, it is prudent to run the experiment with more sleep conditions to mimic the persistent SD that some pregnant individuals may experience. For example, tissue and plasma sam
1.
2.
3.
4.
5.
6.
7.
8.
9.
10.
11.
12.
13.
14. Baratta, A.M., Kanyucha, N.R., Coleb, C.A., Valafarb, H., Deslauriers, J., & Pocivavsek, A. (2020). Acute sleep deprivation during pregnancy in rats: rapid elevation of placental and fetal inflammation and kynurenic acid. Neurobiology of Stress, 2 (100204), 1-9. doi:10.1016/j.ynstr.2019.100204 Borniger, J. C., Don, R. F., Zhang, N., Boyd, R. T., & Nelson, R. J. (2017). Enduring effects of perinatal nicotine exposure on murine sleep in adulthood. American journal of physiology. Regulatory, integrative and comparative physiology, 313(3), R280–R289. https://doi-org.myaccess.library.utoronto.ca/10.1152/ajpregu.00156.2017 Chen, J., Liang, J., Lin, X., Zhang, Y., Zhang, Y., Lu, L., & Shi, J. (2017). Sleep deprivation promotes habitual control over goal -directed control: behavioral and neuroimaging evidence. The journal of neuroscience: the official journal of the society for neuroscience, 37(49), 11979–11992. https://doi-org.myaccess.library.utoronto.ca/10.1523/JNEUROSCI.1612-17.2017 Davies, S.K., Ang, J.E., Revell, V.L., Holmes, B., Mann, A., Robertson, F.P., Cui, N., Middleton, B., Ackermann, K., Kayser, M., Thumser, A.E., Raynaud, F.I., & Skene, D.J. (2014). Effect of sleep deprivation on the human metabolome. Proceedings of the National Academy of Sciences of the United States of America, 111(29), 10761–10766. https://doiorg.myaccess.library.utoronto.ca/10.1073/pnas.1402663111 Dornbierer, D.A., Boxler, M., Voegel, C.D., Stucky, B., Steuer, A.E., Binz, T.M., Baumgartner, M.R., Baur, D.M., Quednow, B.B., Kraemer, T., Seifritz, E., Landolt, H.P., & Bosch, O.G. (2019). NocturnalGamma-Hydroxybutyrate Reduces CortisolAwakening Response and Morning Kynurenine Pathway Metabolites in Healthy Volunteers. The international journal of neuropsychopharmacology, 22(10), 631–639. https://doi-org.myaccess.library.utoronto.ca/10.1093/ijnp/pyz047 Farhangi, M.A., Javid, A.Z., Sarmadi, B., Karimi, P., & Dehghan, P. (2018). A randomized controlled trial on the efficacy of resistant dextrin, as functional food, in women with type 2 diabetes: Targeting the hypothalamic-pituitary-adrenal axis and immune system. Clinical nutrition, 37(4), 1216–1223. https://doi-org.myaccess.library.utoronto.ca/10.1016/ j.clnu.2017.06.005 Ito, T., Maeda, T., Goto, K., Miura, T., Wakame, K., Nishioka, H., & Sato, A. (2014). Enzyme-treated asparagus extract promotes expression of heat shock protein and exerts antistress effects. Journal of food science, 79(3), H413–H419. https:// doi-org.myaccess.library.utoronto.ca/10.1111/1750-3841.12371 Khalyfa, A., Carreras, A., Almendros, I., Hakim, F., & Gozal, D. (2015). Sex dimorphism in late gestational sleep fragmentation and metabolic dysfunction in offspring mice. Sleep, 38(4), 545–557. https://doiorg.myaccess.library.utoronto.ca/10.5665/sleep.4568 Koola, M.M., Sklar, J., Davis, W., Nikiforuk, A., Meissen, J.K., Sawant-Basak, A., Aaronson, S.T., & Kozak, R. (2018). Kynurenine pathway in schizophrenia: Galantamine-memantine combination for cognitive impairments. Schizophrenia research, 193, 459–460. https://doi-org.myaccess.library.utoronto.ca/10.1016/j.schres.2017.07.005 Lees, C.C., Marlow, N., van Wassenaer-Leemhuis, A., Arabin, B., Bilardo, C.M., Brezinka, C., Calvert, S., Derks, J.B., Diemert, A., Duvekot, J.J., Ferrazzi, E., Frusca, T., Ganzevoort, W., Hecher, K., Martinelli, P., Ostermayer, E., Papageorghiou, A.T., Schlembach, D., Schneider, K.T., Thilaganathan, B., … TRUFFLE study group (2015). 2 year neurodevelopmental and intermediate perinatal outcomes in infants with very preterm fetal growth restriction (TRUFFLE): a randomised trial. Lancet, 385(9983), 2162–2172. https://doi-org.myaccess.library.utoronto.ca/10.1016/S0140-6736(14)62049-3 Lo, J. C., Chong, P. L., Ganesan, S., Leong, R. L., & Chee, M. W. (2016). Sleep deprivation increases formation of false memory. Journal of sleep research, 25(6), 673–682. https://doi-org.myaccess.library.utoronto.ca/10.1111/jsr.12436 Maes, M., Verkerk, R., Bonaccorso, S., Ombelet, W., Bosmans, E., & Scharpé, S. (2002). Depressive and anxiety symptoms in the early puerperium are related to increased degradation of tryptophan into kynurenine, a phenomenon which is related to immune activation. Life sciences, 71(16), 1837–1848. https://doi-org.myaccess.library.utoronto.ca/10.1016/s0024 -3205(02)01853-2 Murray, S. A., Morgan, J. L., Kane, C., Sharma, Y., Heffner, C. S., Lake, J., & Donahue, L. R. (2010). Mouse gestation length is genetically determined. PloS one, 5(8), e12418. https://doi.org/10.1371/journal.pone.0012418 Okun, M.L. & Coussons-Read, M.E. (2007). Sleep disruption during pregnancy: how does it influence serum cytokines? Journal of Reproductive Immunology. 73(2), 158-165.
15.
16.
17.
18.
19.
20.
21.
22.
23.
24.
25. Peng, Y., Wang, W., Tan, T., He, W., Dong, Z., Wang, Y.T., & Han, H. (2016). Maternal sleep deprivation at different stages of pregnancy impairs the emotional and cognitive functions, and suppresses hippocampal long-term potentiation in the offspring rats. Molecular Brain, 9(17), 1-10. https://dx-doi-org.myaccess.library.utoronto.ca/10.1186%2Fs13041-016-0197 -3 Plitman, E., Iwata, Y., Caravaggio, F., Nakajima, S., Chung, J. K., Gerretsen, P., Kim, J., Takeuchi, H., Chakravarty, M. M., Remington, G., & Graff-Guerrero, A. (2017). Kynurenic Acid in Schizophrenia: A Systematic Review and Meta-analysis. Schizophrenia bulletin, 43(4), 764–777. https://doi-org.myaccess.library.utoronto.ca/10.1093/schbul/sbw221 Rajendiran, S., Swetha Kumari A, Nimesh, A., Soundararaghavan S, Ananthanarayanan, P. H., & Dhiman, P. (2015). Markers of Oxidative Stress in Pregnant Women with Sleep Disturbances. Oman medical journal, 30(4), 264–269. https://doiorg.myaccess.library.utoronto.ca/10.5001/omj.2015.53 Rosansky, S.J., Menachery, S.J., Whittman, D., & Rosenberg, J.C. (1996). The relationship between sleep deprivation and the nocturnal decline of blood pressure. American journal of hypertension, 9(11), 1136–1138. https://doiorg.myaccess.library.utoronto.ca/10.1016/0895-7061(96)00300-7 Rudzki, L., Ostrowska, L., Pawlak, D., Małus, A., Pawlak, K., Waszkiewicz, N., & Szulc, A. (2019). Probiotic Lactobacillus Plantarum 299v decreases kynurenine concentration and improves cognitive functions in patients with major depression: A double-blind, randomized, placebo controlled study. Psychoneuroendocrinology, 100, 213–222. https://doiorg.myaccess.library.utoronto.ca/10.1016/j.psyneuen.2018.10.010 Salihagic-Kadic, A., Kurjak, A., Medić, M., Andonotopo, W., & Azumendi, G. (2005). New data about embryonic and fetal neurodevelopment and behavior obtained by 3D and 4D sonography. Journal of perinatal medicine, 33(6), 478–490. https://doi-org.myaccess.library.utoronto.ca/10.1515/JPM.2005.086 Sforzini, L. Nettis, M.A. Mondelli, V., & Pariante, C.M. (2019). Inflammation in cancer and depression: a starring role for the kynurenine pathway. Psychopharmacology, 236(10), 2997–3011. https://dx-doiorg.myaccess.library.utoronto.ca/10.1007%2Fs00213-019-05200-8 Toulmonde, M., Penel, N., Adam, J., Chevreau, C., Blay, J. Y., Le Cesne, A., Bompas, E., Piperno-Neumann, S., Cousin, S., Grellety, T., Ryckewaert, T., Bessede, A., Ghiringhelli, F., Pulido, M., & Italiano, A. (2018). Use of PD-1 targeting, macrophage infiltration, and IDO pathway activation in sarcomas: A phase 2 clinical trial. JAMA oncology, 4(1), 93–97. https:// doi-org.myaccess.library.utoronto.ca/10.1001/jamaoncol.2017.1617 Vohra, M., Lemieux, G. A., Lin, L., & Ashrafi, K. (2018). Kynurenic acid accumulation underlies learning and memory impairment associated with aging. Genes & development, 32(1), 14–19. https://doi-org.myaccess.library.utoronto.ca/10.1101/ gad.307918.117 Wichers, M. C., Koek, G. H., Robaeys, G., Verkerk, R., Scharpé, S., & Maes, M. (2005). IDO and interferon-alpha-induced depressive symptoms: a shift in hypothesis from tryptophan depletion to neurotoxicity. Molecular psychiatry, 10(6), 538–544. https://doi-org.myaccess.library.utoronto.ca/10.1038/sj.mp.4001600 Wynne-Edwards, K.E., Edwards, H.E., & Hancock, T.M. (2013). The human fetus preferentially secretes corticosterone, rather than cortisol, in response to intra-partum stressors. PLoS One, 8(6). https://dx-doiorg.myaccess.library.utoronto.ca/10.1371%2Fjournal.pone.0063684