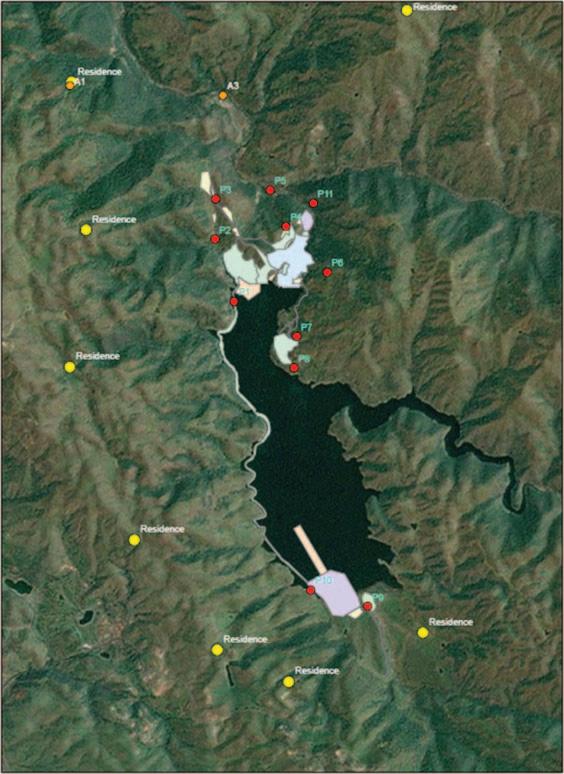
12 minute read
NOA Air-Quality Lessons Learned during Calaveras Dam Replacement Project
from EEG Journal - February 2020 Vol. XXVI, No. I (2)
by Association of Environmental & Engineering Geologists (AEG)
BART EKLUND* AECOM Corporation, 9400 Amberglen Boulevard, Austin, TX 78729
JOHN ROADIFER NOEL WONG MICHAEL FORREST AECOM Corporation, Kaiser Center, 300 Lakeside Drive, Oakland, CA 94612
Key Terms: NOA, Asbestos, Air Quality, Emission Controls
ABSTRACT
The Calaveras Dam Replacement Project (CDRP) pioneered technical approaches for addressing community exposure to naturally occurring asbestos (NOA) via the inhalation pathway. Over the course of the CDRP, approaches were developed for key issues, including determining the NOA particles of interest, defining the toxicity limits to apply to various types of NOA particles, establishing dust control, and creating appropriate feedback loops for using laboratory data. Specific issues of interest included whether to count only structures above a certain length and the inhalation unit risk value to use for amphiboles. The knowledge gained on the CDRP can and is being used to optimize NOA evaluation and control at other, similar projects.
INTRODUCTION
The original Calaveras Dam was constructed in 1925 in Alameda County, CA. At the time, it was the largest earth-fill dam in the world. The resulting reservoir was situated primarily in Santa Clara County and had a capacity of 100,000 acre-ft (120 million m 3 ) and a surface area of approximately 1,450 acres (590 ha). It was determined that the hydraulic fill dam was subject to liquefaction failure during seismic activity, and the capacity of the reservoir was restricted to approximately 40 percent capacity by California regulators in 2001.
To restore lost capacity, the San Francisco Public Utilities Commission (SFPUC) constructed a replacement dam located approximately 1,000 ft (300 m) downstream of the original dam. This was called the Calaveras Dam Replacement Project (CDRP).
* Corresponding author email: bart.eklund@aecom.com
Construction on the new earth- and rock-fill dam began in August 2011, and construction activities were completed in late 2018. The new dam is 220 ft (67 m) high and 1,180 ft (360 m) wide at the base. The volume of the dam is 3.5 million yd 3 (2.7 million m 3 ). About 11 million yd 3 (8.4 million m 3 ) of material was excavated during construction, some of which contained naturally occurring asbestos (NOA). A broad definition of NOA was applied (i.e., California Air Resources Board Asbestos Hazard Emergency Response Act [CARB-AHERA] structures), as discussed later in this paper.
Air-Quality Program
A comprehensive air-quality program was undertaken to address species of interest. Pre-construction air monitoring was performed to develop a baseline of local air quality. Twenty-four-hour, time-integrated samples generally were collected on a monthly basis. In total, 22 monthly monitoring events were performed from August 2008 through December 2009 and from May 2010 through November 2010.
Routine air monitoring began in January 2012 and continued through November 2018. The work was done in compliance with a Comprehensive Air Monitoring Program (CAMP) document that served as the test plan (URS, 2012). The CAMP document was amended about a dozen times between October 2013 and December 2016 as new information became available and the results-to-date were reviewed and evaluated.
The dam construction took place in a valley that primarily ran north-south. There were relatively few residents located within 5 mi (8 km) of the construction activities in either direction, but the largest number of potential receptors was to the north. The monitoring network included six community monitoring locations located to the north over an extended distance, and
about 12 perimeter monitoring locations located closer in and surrounding the construction activities.
One key element of the air-quality program was the development of target concentrations for NOA at the community and perimeter monitoring locations. Target concentrations were developed for both total asbestos and for amphiboles. These target concentrations were revised several times over the course of the program to account for changes in site activities (e.g., the addition of nightshift work) or changes in assumed exposures (e.g., whether or not receptors aged 0–5 years were present). The target concentrations for the community monitoring stations were based on total risk from all NOA sources, with the relative contribution from CDRP being up to 10 percent of the total risk.
The target concentrations for the perimeter monitoring stations were based on results of atmospheric dispersion modeling and were set to ensure that NOA concentrations further downwind would not exceed acceptable levels. An attenuation factor approach was used to set perimeter trigger levels, which resulted in the location of the perimeter stations being an important factor in how conservative the trigger levels were for evaluating potential exposure of receptors further downwind. The modeling addressed multiple emission areas, various types of construction equipment, fugitive dust from vehicle traffic, and emission sources that changed in elevation over time. The modeling involved complex terrain and “flagpole” receptors (i.e., receptors located above the elevation where air emissions were released).
Key Air-Quality Issues
There were six air-quality issues that posed technical or logistical challenges: 1. define what to measure; 2. select analytical approach; 3. define the acceptable ambient air concentration; 4. determine where to measure; 5. determine the feasible emission-control options; and 6. establish a process for tracking and evaluating airquality data. Each of these issues is discussed, in turn, below.
Define What to Measure
The original scope of work addressed NOA, inhalable dust (PM 10 ), and elements associated with particulate matter (arsenic, chromium, cobalt, copper, and nickel). It became apparent during the early stages of the regular air-monitoring effort that particulate matter and the various metals and arsenic were not an issue. Monitoring and reporting of these species eventually was discontinued, and over the later stages of the project, NOA was the only air-quality species of concern.
Select Analytical Approach
From the beginning of the project, it was deemed that Occupational Safety and Health Administration (OSHA) methods would not be sufficiently protective. Under OSHA, an asbestos fiber is defined as a particle that is 5 μm or longer, with a length-to-width ratio of 3:1 or longer (OSHA, 1988, revised 1997). Furthermore, the fibrous minerals defined as asbestos are those listed as commercially exploitable mineral fibers (i.e., chrysotile, crocidolite, amosite, tremolite, actinolite, and anthophyllite). Analysis is typically by phasecontrast microscopy (PCM), and results are typically reported in units of fibers per cubic centimeter (f/cc). For this project, CARB-AHERA structures were measured. The CARB-AHERA approach counts both asbestos structures visible by PCM and those up to an order of magnitude smaller. CARB-AHERA structures are defined as being 0.5 μm or longer, with an aspect ratio of 3:1 or greater, and largely parallel sides. Data were reported as structures per cubic centimeter (s/cc). The analysis included characterization of mineral type (chrysotile or amphibole). Two additional analyses were performed. In one analysis, fibers that met the OSHA definition, with fiber lengths 5 μm, were counted separately. In the second analysis, detailed fiber size ratio analysis and/or identification of specific minerals present were done for up to 5 percent of the samples.
Samples were analyzed using transmission electron microscopy (TEM). Approximately 40,000 samples were analyzed over the course of the project. The detection limit achieved was dependent on the air volume sampled and the NOA loading. The number of grid openings that were counted had an effect on the analysis cost and the turnaround time.
Define the Acceptable Ambient Air Concentration
The usual “gold standard” for risk-based inhalation concentrations is the U.S. Environmental Protection Agency (USEPA) IRIS database. The USEPA has published an inhalation unit risk of 0.23 (f/cc) −1 for asbestos in IRIS (USEPA, 1988). Stated another way, the one-in-a-million risk level is 4E−06 f/cc. This value applies to both chrysotile and amphiboles. For the CDRP, a more conservative approach was employed, where the EPA value for chrysotile was used but amphiboles were assigned 10 times greater risk
Figure 1. Perimeter monitoring locations in relation to work areas, where perimeter monitoring locations are shown as red dots, work areas are shown as shaded areas, and residences are shown as yellow dots.
than chrysotile. This entailed some risk of project shutdown, as it was not apparent early on whether the stricter target concentrations could be met.
Some additional uncertainty was present because the target concentrations were based on atmospheric dispersion modeling of particulate matter. Asbestos could not be modeled directly because there were no emission factors for asbestos (e.g., emission rate per hour of dozer operation).
Define Where to Measure
The perimeter monitoring network is shown in Figure 1. There were relatively few receptors near the work site, as previously discussed, so the community monitoring sites extended over several miles. One site eventually was moved to avoid a localized emission source (i.e., gravel parking lot) that contributed to anomalous results. One of the perimeter monitoring locations also was moved to avoid a localized emission source (i.e., re-entrainment from a vehicle
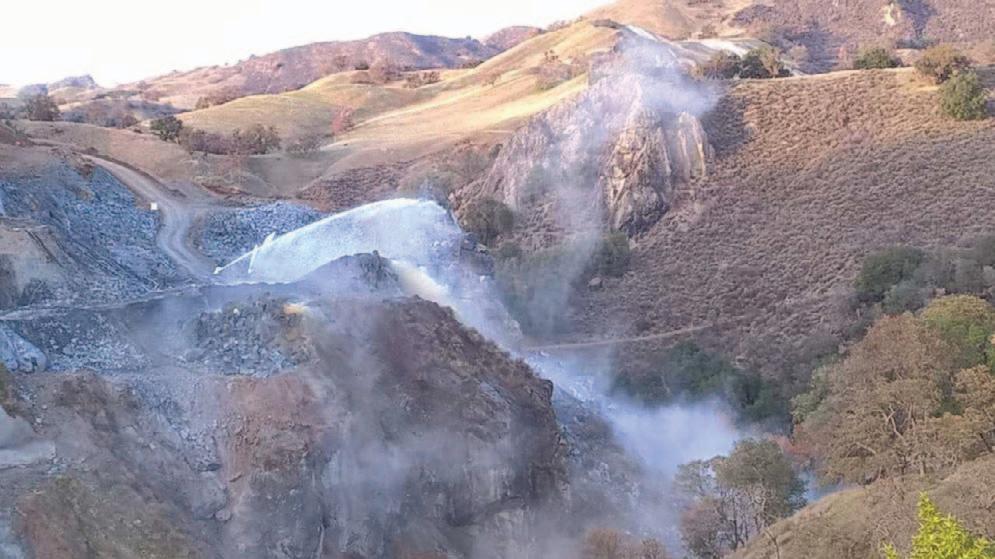
Figure 2. Air emissions of particulate matter near perimeter monitoring station P11 (note water spray used for dust control).
washing station). Some of the work activities took place quite close to the perimeter monitoring locations, and it is likely that the monitoring results were biased high on those days (relative to downwind concentrations). Figure 2 shows an example of work being performed adjacent to a perimeter monitoring station. The community monitors proved to be less useful than originally anticipated. The baseline air monitoring took place during an economic recession when home building and construction activities were at a low point. As the economy recovered, increased activity resulted in higher levels of NOA being generated from non-CDRP sources than were encountered during the baseline period. This became apparent, in part, through evaluation of air-monitoring data collected on days when there was no construction activity at the CDRP site (e.g., Sundays) and comparison of the specific minerals present for monitors near the site versus monitors farther away. Therefore, it was difficult to readily distinguish the CDRP contribution to the total NOA loading at distant locations.
Determine the Feasible Emission-Control Options
At the start of the project, there were no data available on the control options that would best address NOA and the level of effectiveness to expect. The available information was for the effectiveness of water sprays with and without additives for dust control (USEPA, 1992).
Water sprays were employed extensively at the site for dust control and for controlling serpentinites, but they were found to be of limited effectiveness for amphiboles. Increasing the water usage did not lead to a commensurate reduction in amphibole concentrations. Various water-delivery systems were tried (e.g., DustBoss, Kuma) in an effort to achieve better NOA control. It is known, however, that the water droplets need to be about the same size as the particles to be
controlled for best effectiveness. If the droplets are too big, the dust particles will tend to move around the droplet and not collide with the droplet. Given the very small size of NOA structures, we believe the size difference between the structures and the smallest mist or water droplets that could be generated was the reason why the effectiveness did not meet expectations.
Air emissions could be controlled or limited using standard options for construction sites, such as minimizing work under high wind conditions and adjusting the rate of production. These options were employed sparingly, given logistical and schedule constraints.
Establish a Process for Tracking and Evaluating AirQuality Data
The original plan was to use the off-site analytical results to guide on-site activities. This did not prove to be practical for several reasons. First, the time lag between sample collection and receipt of laboratory results meant that different activities might be under way at the site when results were received compared to when the data were generated. Second, the options for controlling NOA were limited, as discussed above. Knowing that NOA emissions were higher for a given activity was of limited help for addressing off-site, non-occupational exposures if there were not adequate means to mitigate those emissions.
At many sites, it has been found that visual observations and real-time measurements of dust proved to be the best tools for on-site management of particulate matter air emissions (Eklund et al., 2014). The off-site analytical results still serve the important purpose of documenting the air quality over time. For the Calaveras project, however, visual observations and real-time measurements proved to be less useful than at other sites, due to the small size of the NOA particles versus particle size fractions such as PM 2.5 or PM 10 , which can be visible and are more amenable to real-time measurement using opacity-based or beta attenuation monitors.
There was an intensive, on-going effort to evaluate the data as they were collected, and this proved to be crucial for understanding the fate and transport of NOA. This work demonstrated that activities such as blasting, which were expected to be major sources of NOA, were of too-limited duration to be significant in the overall accounting of air emissions. Although higher concentrations were measured downwind when blasting occurred, the blasting represented 1 percent of the overall time. Relatively early in the project, elevated NOA concentrations were measured during a brief period when site activities were at a high level and the meteorological conditions were very unfavorable for atmospheric mixing and dispersion. The on-going data evaluation helped to show that this time period was an outlier and unlikely to be repeated. The ability to predict future NOA concentrations became more refined over time and provided some assurance over the final years of the project that air quality was under control and would continue to be within acceptable limits. The emission predictions took into account the number of hours worked per week as well as the type of activity, the level of activity, and the location of work.
CONCLUSIONS
NOA can pose large challenges for maintaining acceptable air quality at major construction projects. CDRP expended significant resources to better understand the fate and transport of NOA. The state of knowledge was much greater at the end of the project than at the start because of these efforts.
It is not possible to achieve complete control of amphibole emissions for these types of large-scale construction projects. This needs to be taken into account during planning of future, similar work.
A multi-disciplinary team proved to be an effective approach for dealing with the multi-media, complex issues posed by this work. Expertise in asbestos, construction practices, dust control, air-quality monitoring, etc., was utilized from an early stage in the process.
REFERENCES
Eklund, B.; Fitzgerald, C.; Wade, M.; Wilder, R.; and LaMond, D., 2014, Controlling air toxics emissions from remediation by monitoring of surrogate parameters: Remediation, Vol. 24, No. 4, pp. 127–138. Occupational Safety and Health Administration (OSHA), 2019, Method No. ID-160. Asbestos in Air, July 1988 (Revised July 1997): Electronic document available at: https://www. osha.gov/dts/sltc/methods/inorganic/id160/id160.pdf. URS Corporation, 2012, Comprehensive Air Monitoring Program (CAMP), Calaveras Dam Replacement Project: Report to the San Francisco Public Utilities Commission, San Francisco, CA. U.S. Environmental Protection Agency (USEPA), 1988, Integrated Risk Information System (IRIS), Chemical Assessment Summary, Asbestos: CASRN 1332-21-4: USEPA. Washington, DC. U.S. Environmental Protection Agency (USEPA), 1992, Control of Air Emissions from Superfund Sites: USEPA, Center for Environmental Research Information Report EPA/625/R92/012.