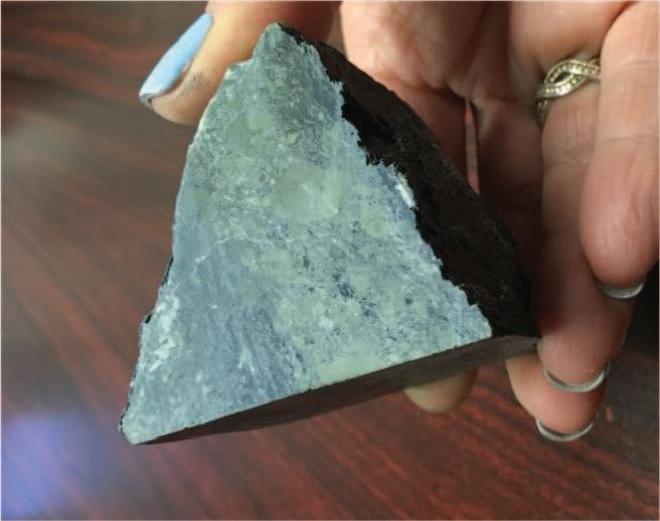
12 minute read
Does Exposure to Naturally Occurring Asbestos (NOA) During Dam Construction Increase Mesothelioma Risk?
from EEG Journal - February 2020 Vol. XXVI, No. I (2)
by Association of Environmental & Engineering Geologists (AEG)
DANIEL W. HERNANDEZ* Dragados USA, Inc., Flatiron West, Inc., Sukut Construction, Inc., Joint Venture (DFSJV), Calaveras Dam Replacement Project, 8022 Pinot Noir Court, San Jose, CA 95125
Key Terms: NOA, Amphiboles, Mesothelioma, Blueschist, Glaucophane, Particle Dimensions
ABSTRACT
The Calaveras Dam Replacement Project, a major construction project completed in 2019, involved hundreds of workers using heavy earth-moving equipment and mining operations, including blasting, drilling, rock crushing, and other operations designed to move millions of cubic yards of earth. Much of the material was composed of serpentinite, blueschist, and other rocks that contain chrysotile and a variety of amphibole minerals, including glaucophane, winchite, actinolite, tremolite, and other asbestos-related amphiboles. This article explores the unique characteristics of the blueschist that required extensive protective measures to be undertaken by the contractor to protect workers and surrounding sensitive receptors. This article will provide an overall summary of the dimensional characteristics of the airborne blueschist elongate mineral particles encountered during construction activities to compare and contrast current understanding of cleavage fragments versus asbestiform mineral fibers.
INTRODUCTION
Located to the east of the San Francisco Bay Area, the Calaveras Dam Replacement Project is substantially underlain by exhumed Franciscan mélange composed of a variety of rocks. Rocks encountered during construction included serpentinite, which contains chrysotile, blueschist, greenstone, and other rocks that contain various amphiboles. At least 20 species of amphiboles, including actinolite and glaucophane (considered naturally occurring asbestos [NOA] minerals [NIOSH, 2011]), were discovered within the project boundary. In addition, winchite (associated with the well-known Libby Amphibole [U.S. EPA, 2014]) was also determined to be present.
*Corresponding author email: risicaredan@gmail.com
The construction of the dam required the disturbance of over 13 million yards of these materials involving operations that included mining, drilling, blasting, sorting, sizing, dozing, excavating, loading, transporting, dumping, and compaction. Operations complementing the construction of the replacement dam included slope shaping, rock breaking, and rock crushing.
Early in the project, the contractor noted that countable fibers (fibers with a 3:1 or greater length:width aspect ratio, widths greater than 0.25 μm, and length greater than 5 μm) were substantially higher in personal breathing zone exposure samples collected during the disturbance of the blueschist, as compared to during the disturbance of other NOA-containing media (serpentinites). This was somewhat surprising, since the “blueschist rock” did not appear to be asbestiform, as in the classical definition of asbestos as “visually fibrous,” with individual fibers being separable by finger pressure (Figure 1).
Since the dam required the mining and processing of approximately one million cubic yards of blueschist for the upstream rock shell, the contractor conducted a thorough industrial hygiene investigation of blueschist operations. Based on thousands of air samples collected, it was determined that glaucophane (compositionally similar to crocidolite) and winchite were the dominant amphiboles emitted when disturbing blueschist materials. The blueschist amphibole mineral belongs in the solid solution series of gluacophaneriebekite, which is currently recognized by the National Institute for Occupational Safety and Health (NIOSH) as a non-asbestiform habit of an asbestos mineral and is considered “asbestos” if cleavage fragments derived from this material meet the definition of fiber, as viewed microscopically (NIOSH, 2011).
Considerable issues are associated with the disturbance of amphibole mineral rocks since the asbestiform crystalline habit is relatively rare in nature (Harper et al., 2008; Wylie, 2017), as compared to that of the coarsely crystalline non-asbestiform analog of the same mineral.. Disturbance of these non-asbestiform mineral analogs may result in the release of “cleaved” elongate mineral particles and/or
Mean % > 5 % > 10 Length (µm) (µm) (µm)
VO cleavage fragments 3.36 10.9 1.2 Cleavage fragments w/o riebeckite 3.2 7.9 0.75 VO asbestiform 11.99 55.4 30.3 Blueschist 1 4.2 23.8 1.6 Blueschist 2 5.4 42.4 7.1
VO = Van Orden.
Figure 1. Greenstone breccia with blueschist matrix.
individual non-asbestiform crystals that have countable morphologies under current counting rules (NIOSH, 2011). The primary controversy relates to the relative toxicity of these particles as compared to asbestos.
Research (Aust, 2011) has generally demonstrated that the potency of asbestos may be related to its fiber dimensions, its persistence in the lung, and the surface characteristics of the fibers. In addition, researchers (Harper et al., 2008; Van Orden et al., 2016) have also suggested that dimensional differences exist between asbestos and non-asbestos amphibole samples and that these differences can be used to identify true “asbestiform” fibers.
This article includes the examination of bulk material and emitted particle size distributions, with comparison of particle size characteristics to other research findings, as well as exposure data associated with the particle size distributions.
BLUESCHIST BULK SAMPLE PARTICLE SIZES
Various researchers have examined particle size distributions of non-asbestiform amphibole particles and asbestiform amphibole particles to determine differences and similarities in order to identify means which could be useful for differentiating each. Harper et al. (2008) suggested that a maximum width criterion of 1 µm for asbestos measurement purposes could adequately include asbestos while minimizing the counting of cleavage fragments.
In an extensive study, Van Orden et al. (2016) compared the particle size distributions of five regulated asbestiform species to their non-asbestiform analogs. The findings of this study indicated that very clear differences exist between length and width distributions of asbestiform versus non-asbestiform particles. However, overlapping dimensional similarities were also demonstrated.
To compare to the findings of Van Orden et al. and Harper et al., blueschist bulk samples collected from the dam’s blueschist mining area were disaggregated using a jaw crusher and pulverizer (Asbestos TEM Labs, Berkeley, CA). Using Van Orden’s counting rules, particle sizing was then performed by ALS Labs (Cincinnati, OH). Tables 1 and 2 compare the length and width characteristics of the blueschist amphibole to the average length and width characteristics of cleavage fragments and asbestiform particles reported by Van Orden et al. (2016).
As can be seen in Tables 1 and 2, blueschist bulk sample particle sizes are thinner and longer than the average cleavage fragment populations of the regulated non-asbestiform amphiboles investigated by Van Orden et al.. It is important to note from a health risk perspective that average cleavage fragment particle sizes reported in Tables 1 and 2 included non-asbestiform riebeckite (a sodic-amphibole), which biased average non-asbestiform population dimensions to longer lengths and thinner widths. Health risk implications are further discussed below.
HIGH-RISK ELONGATE MINERAL PARTICLE (EMP) DIMENSIONS
As previously mentioned, the potency of asbestos EMPs is generally related to particle dimensions, durability of the particle in the lung, mineral
Table 2. Width characteristics of bulk blueschist samples.
Mean % < 0.5 % < 1.0 Width (µm) (µm) (µm)
VO cleavage fragments 1.24 7.2 42.3 Cleavage fragments w/o riebeckite 1.35 1.8 33.8 VO asbestiform 0.244 94.3 100 Blueschist 1 0.52 71.8 88.7 Blueschist 2 0.54 65.9 87.2
VO = Van Orden.
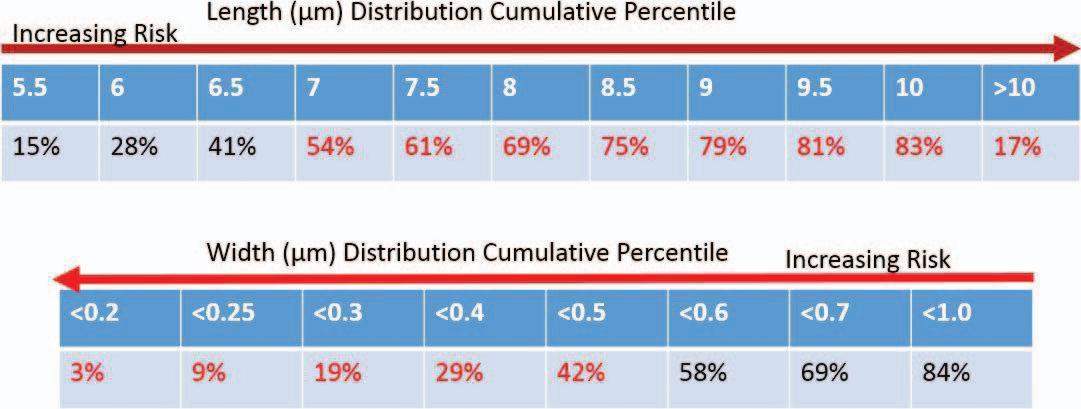
Figure 2. Cumulative length and width distribution of airborne blueschist EMPs > 5 µm in length emitted during construction operations. Length Distribution includes % of airborne EMPs with lengths greater than 10 um.
composition, and surface characteristics of the EMP. With respect to the non-asbestiform analogs of regulated asbestos minerals, particle dimensions are generally different than those of asbestos, and human, animal, and other evidence indicate lower potency (Gamble and Gibbs, 2008; Mossman, 2008; and Wylie, 2016). However, the relative difference in potency (if any) between an amphibole asbestos fiber and an individual non-asbestiform crystal, or a cleaved EMP from the same non-asbestiform mineral given the same dimensions, has not been resolved. Further complicating these issues, researchers (Gamble and Gibbs, 2008) have indicated that complete particle size data (length versus diameter) on distributions of cleavage fragments and asbestos fibers are extremely limited in number, making it difficult to compare length and width distributions.
Stanton et al. (1981) hypothesized that biological activity is related to the dimension and durability of the particle. He found that neoplastic responses correlated well with the dimensional distribution of fibers and that durable particles less than 0.25 µm in width and greater than 8 µm in length correlated most strongly. Other researchers (Wylie, 2016) have indicated that most mesothelioma-causing fibers are very thin and are greater than 10 µm in length and found that particles longer than 5 µm with widths of <0.33 µm and <0.4 µm have been shown to be retained by the lung and preferentially transported to the pleura. Berman et al. (1995) found that structures greater than 5 µm in length and less than 0.4 µm in width appear to contribute to lung tumor risk in laboratory rat inhalation studies.
Wylie et al. (1993) suggested that all fiber populations of similar width, length, and crystal morphology of asbestos should be viewed with caution and given deference with respect to biological testing. With respect to dimensional aspects, longer, thinner fibers are considered more potent than shorter, thicker fibers, and amphibole fibers are more bio-durable in lung tissues than are those of chrysotile. Other things to consider are that fiber width controls penetration into the lung and that fiber length may control residence time (Wylie, 2017).
PARTICLE SIZE ANALYSIS OF BLUESCHIST AIRBORNE EMP S
Perhaps the most important information gathered by the contractor at the project site is the enumeration and particle sizing of EMPs emitted into the breathing zone of workers disturbing the blueschist amphibole. To better understand potential health risks associated with disturbing the blueschist amphibole during construction operations, 17 breathing zone samples were collected from workers involved in the blueschist mining operations. Each sample was evaluated for total countable fibers in addition to particle size analysis. Structures greater than 1 µm in length and meeting a minimum length to width aspect ratio (AR) of 3:1 were counted and sized using transmission electron microscopy methods by ALS Labs. Analysis of each sample was terminated upon completion of the grid opening containing the 100th EMP. A total of 1,946 particles were sized.
All workers involved in these operations were equipped with air-purifying respirators and Tyvek protective coveralls. Water was sprayed continuously as a dust suppressant. Mining operations included sorting, sizing, dozing, and loadout of bulk blueschist rock
Figure 3. Relative frequency of the aspect ratios of airborne blueschist EMPs > 5 µm in length.
to be transported to the upstream rock shell of the dam. Phase Contrast Microscopy (PCM) results for these breathing zone samples ranged from 0.078 f/cc to 1.2 f/cc, with a geometric mean of 0.512 f/cc. The CAL/OSHA permissible exposure limit (PEL) for regulated asbestos is 0.1 f/cc (as an 8-hour time-weighted average).
Figure 2 depicts the length and width distributions of airborne EMPs from 17 breathing zone samples, and Figure 3 shows the relative frequency of aspect ratios of the airborne blueschist EMPs of >5 µm in length. As can be seen in Figure 3, 35 percent of airborne blueschist EMPs greater than 5 µm in length have aspect ratios greater than 20:1. Figure 4 depicts an electron micrograph of a single blueschist EMP with a width of 0.204 µm, length of 13.8 µm, and aspect ratio of 68:1. CONCLUSIONS
Bulk and air samples collected during mining of bulk blueschist rock show that blueschist EMPs at the dam construction site were found in the “Asbestiform Size Range”; thus, blueschist EMPs at this locality should be considered fibrous rather than cleavage fragments. The toxicity of the blueschist amphibole is unknown, but based on particle sizes found in exposure samples, at least 30 percent of the airborne EMPs should be considered very high risk, and perhaps up to 40 percent should be considered high risk.
As indicated by personal air monitoring results (reported greater than the CAL/OSHA PEL of 0.1 f/cc), disturbing this material required dust controls, personal protective equipment, and exposure monitoring
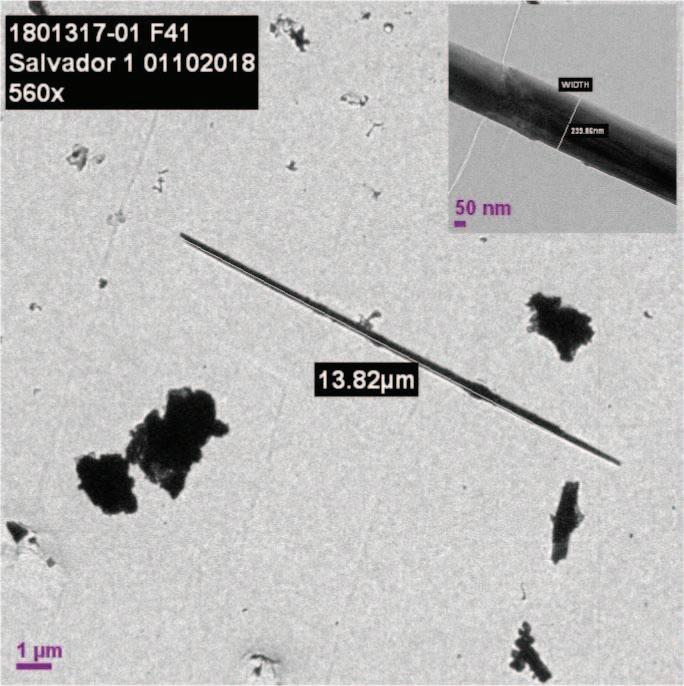
Figure 4. Electron micrograph of a single blueschist EMP.
to ensure that protective equipment ensembles were adequate for the tasks at hand.
REFERENCES
Aust, A. E., Cook, P. M., Dodson, R. F., 2011, Morphological and chemical mechanisms of elongated mineral particle toxicities: Journal Toxicology Environmental Health, Part B Critical Reviews, Vol. 14, No. (1–4), pp 40–75. Berman, D. W., Krump, K. S., Chatfield, E. J., Davis, J. M., Jones, A. D., 1995, The sizes, shapes, and minerology of asbestos structures that induce lung tumors in AF/Han rats following inhalation: Risk Analysis, Vol. 15, No. 2, pp. 181–195. Gamble, J. F. and Gibbs, G. W., 2008, An evaluation of the risks of lung cancer and mesothelioma from exposure to amphibole cleavage fragments: Regulatory Toxicology Pharmacology, Vol. 52, No. 1, pp. S155–S186. Harper, M., Lee, E. G., Doorn, S. S., Hammond, O., 2008, Differentiating non-asbestiform amphibole and amphibole asbestos by size characteristics: Journal Occupational Environmental Hygiene, Vol. 5, No. 12, pp. 761–770. Mossman, B. T., 2008, Assessment of the pathogenic potential of asbestiform vs. non-asbestiform particulates (cleavage fragments) in in vitro (cell or organ culture) models and bioassays: Regulatory Toxicology Pharmacology, Vol. 52, No. 1, pp. S200–S203. National Institute for Occupational Safety and Health (NIOSH), 2011, NIOSH Bulletin 62, April 2011: Asbestos Fibers and Other Elongate Mineral Particles: State of the Science and Roadmap for Research: Department of Health and Human Services, Centers for Disease Control and Prevention, National Institute for Occupational Safety and Health. Cincinnatti, Ohio. Revised edition, pp. 33–34. Stanton, M. F., Layard, M., Tergeris, A., Miller, E., May, M., Morgan, E., Smith, A., 1981, Relation of particle dimensions to carcinogenicity in amphibole asbestos and other fibrous minerals: Journal National Cancer Institute, Vol. 67, No. 5, pp. 965–976. U.S. Environmental Protection Agency (U.S. EPA), 2014, Toxicological Review of Libby Amphibole Asbestos: Integrated Risk Information, National Center for Environmental Assessment, Office of Research and Development, U.S. EPA, Washington, DC. Van Orden, D. R., Lee, R. J., Hefferan, C. M., Schlaegle, S., Sanchez, M., 2016, Determination of the size distribution of amphibole asbestos and amphibole non-asbestos mineral particles: Microscope, Vol. 64, No. 1, pp. 3–15. Wylie, A. G., 2017, Chapter 2 Asbestos and fibrous erionite. In Testa, J. R. (Editor), Asbestos and Mesothelioma, Current Cancer Research: Springer International Publishing. doi:10.1007/978-3-319-53560-92. Gewerbestrasse 11, 6330 Cham, Switzerland. Wylie, A. G., 2016, Amphibole dusts: Fibers, fragments, and mesothelioma: Canadian Mineralogist, Vol. 54, No. 6, pp. 1403–1435. Wylie, A. G., Bailey, K. F., Kelse, J. W., Lee, R. J., 1993, The importance of width in asbestos fiber carcinogenicity and its implications for public policy: American Industrial Hygiene Association Journal, Vol. 54, No. 5, pp. 239–252.