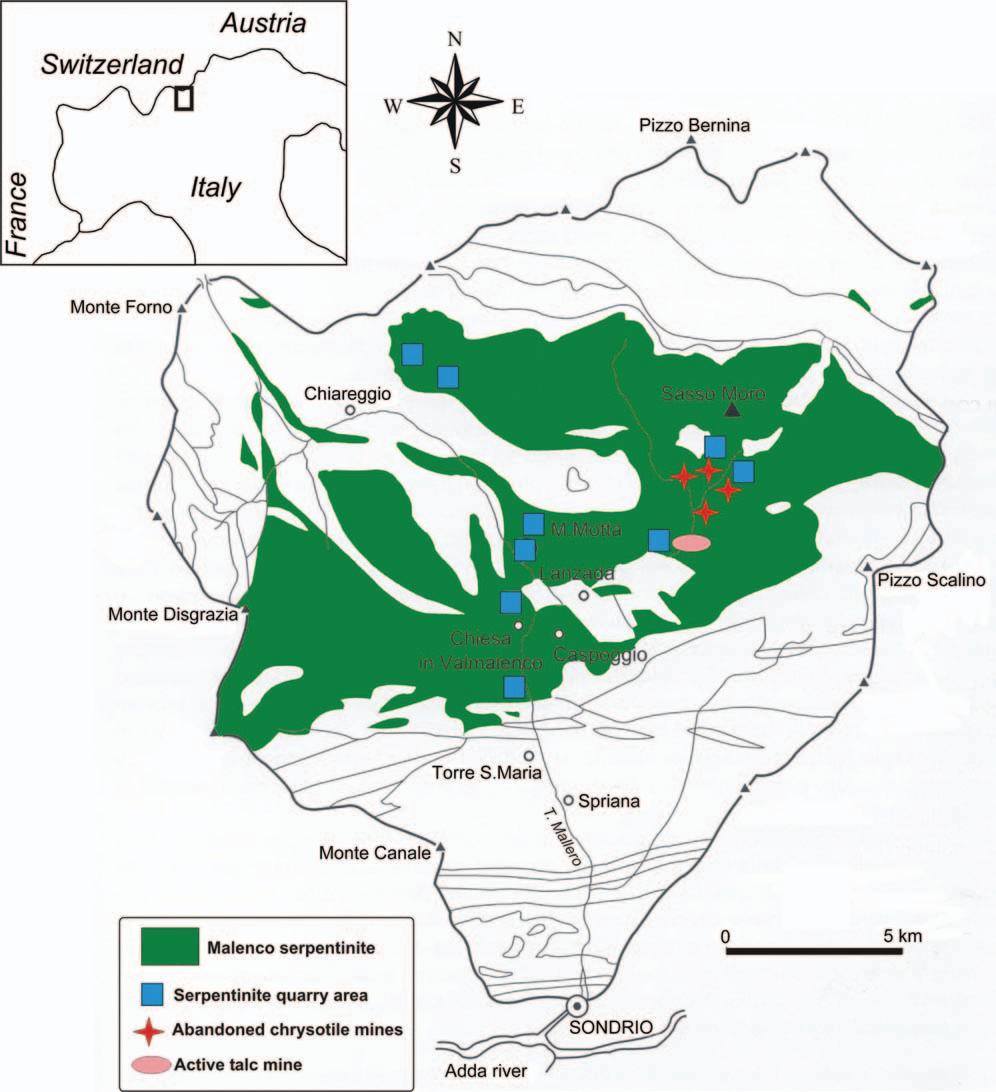
13 minute read
Naturally Occurring Asbestos in Valmalenco (Central Alps, Northern Italy): From Quarries and Mines to Stream Sediments
from EEG Journal - February 2020 Vol. XXVI, No. I (2)
by Association of Environmental & Engineering Geologists (AEG)
ALESSANDRO CAVALLO* Department of Earth and Environmental Sciences, University of Milano-Bicocca, Milano, Italy
JASMINE RITA PETRIGLIERI Department of Chemistry, University of Torino, Torino, Italy
Key Terms: NOA, Asbestos, Serpentinite, Quarries, Soils, Sediments
ABSTRACT
The Valmalenco area (central Alps, northern Italy) is an excellent case study for naturally occurring asbestos (NOA) because of the huge outcrops of serpentinites and widespread quarrying and mining activities. Extensive sampling of rocks, soils, stream sediments, and airborne asbestos has been in progress since 2004. The combined use of scanning electron microscopy and transmission electron microscopy has proven to be effective for the correct discrimination between asbestiform and non-asbestiform mineralogical varieties (but falling into the World Health Organization fiber definition), whereas phase contrast microscopy has not proven suitable because of the very small size of fibrils after strong mechanical fragmentation. The quantitative analysis of “massive” samples (rocks, soils, and sediments) requires accurate and representative sampling as well as specific counting and discrimination criteria to determine NOA. Over a decade of experience has allowed us to identify critical issues and adopt effective preventive measures.
INTRODUCTION
The Valmalenco area (central Alps, northern Italy) is characterized by huge outcrops of serpentinites of the Malenco nappe (lower crust–mantle complex) at the Penninic to Austroalpine boundary zone. The Malenco nappe covers an area of about 130 km 2 (Figure 1) and consists mainly of ultramafic rocks, especially schistose serpentinites, showing various degrees of deformation and serpentinization, ranging from massive, layered lherzolites to schistose, completely serpentinized rocks. The predominant
*Corresponding author email: alessandro.cavallo@unimib.it
rock type is a schistose serpentinite with nonpseudomorphic texture, and the rock-forming minerals are antigorite, olivine, diopside and minor magnetite, chlorite, and chrysotile (only in veins). The area is characterized by four main metamorphic events, and minerals such as antigorite, olivine, clinopyroxene, amphibole, and carbonates occur in several generations (Münterer & Hermann, 1996). Field data demonstrate that the Malenco ultramafics formed the lithospheric subcontinental mantle below the Margna basement during pre-Alpine, postVariscan times (Münterer et al., 2000).
Many different mining and quarrying activities have been active in the past or still are being undertaken (Figure 1). Long-fiber chrysotile asbestos, which occurs in discrete cross-fiber and slip-fiber veins (Figure 2), gave rise to widespread asbestos mining, particularly between the end of the 19th century and 1975, and was used mainly for weaving tablecloths or for candle wicks. A big boost to mining activity occurred during World War II and immediately thereafter, with annual production up to 670 tons and more than 400 workers employed, until mining operations ended completely in 1975, leaving huge amounts of mining waste and tailings.
The serpentinite is a well-known ornamental and building stone, a green “marble,” extracted at least since the 11th century, exported to Switzerland before the 18th century, and abundantly used in Sondrio and Valtellina since the 14th century. Today the Malenco serpentinite is appreciated and exported all over the world for its excellent chromatic and technical features. There are more than 20 quarrying enterprises in the Malenco valley, processing approximately 195 kt/yr of serpentinite, with more than 150 workers involved. The quarried serpentinites show various textures (schistose to massive) and color shades. The schistose variety is prevalently split in thin slabs for roof covering, whereas the “massive” serpentinite is processed in many ways: dressed, polished, bush hammered, and sandblasted. A
the Italian company IMI Fabi is the third-largest producer of talc in the world.
The huge outcrops of mafic and ultramafic rocks, as well as the important extractive activities, make this area an excellent case study of naturally occurring asbestos (NOA). Airborne fibers have been monitored systematically since 2004, especially in quarries and processing facilities (Cattaneo et al., 2012; Cavallo and Rimoldi, 2013), but there are few data about the serpentinite (dimension stone), the rock mass, soils, and stream sediments. The aim of this work is to increase knowledge of the distribution and abundance of asbestos in the environment. The Italian threshold for asbestos in rocks and soils is 1,000 mg/kg (or ppm; Italian D.Lgs. 152/06), whereas the occupational exposure limit for airborne asbestos in workplaces is 100 f/l of air (8-hour time-weighted average) and the environmental exposure limit is 2 f/l.
Figure 1. Simplified sketch map of the Valmalenco area, showing the extension of the Malenco nappe, the location of the serpentinite quarries, the abandoned chrysotile, and the active talc mine.
huge amount of waste products (irregular blocks, rock chips, and cutting sludge) derive from the quarrying and processing activities (Cavallo, 2018).
Steatite is also actively mined in the area: it is an impure talc, used mainly as a filler for rubber and plastics, that occurs in metric, sub-vertical lodes within the serpentinites and/or at the contact with dolomitic marbles. The extraction occurs in underground mines, and MATERIALS AND METHODS
Extensive sampling of rocks, soils, and stream sediments was conducted from 2004 to 2018, and airborne asbestos concentrations have been monitored at least once a year since 2004.
Airborne asbestos was analyzed mainly in the summer season in more than 250 personal air filters (41 phase contrast microscopy [PCM], 215 scanning electron microscopy/energy dispersive spectroscopy [SEM-EDS], and 11 transmission electron microscopy [TEM]) and 43 environmental air samples (SEM-EDS), collected from different quarrying and processing locations, at quarry property borders, and at the closest villages. The World Health Organization (WHO, 1997) method was the reference method
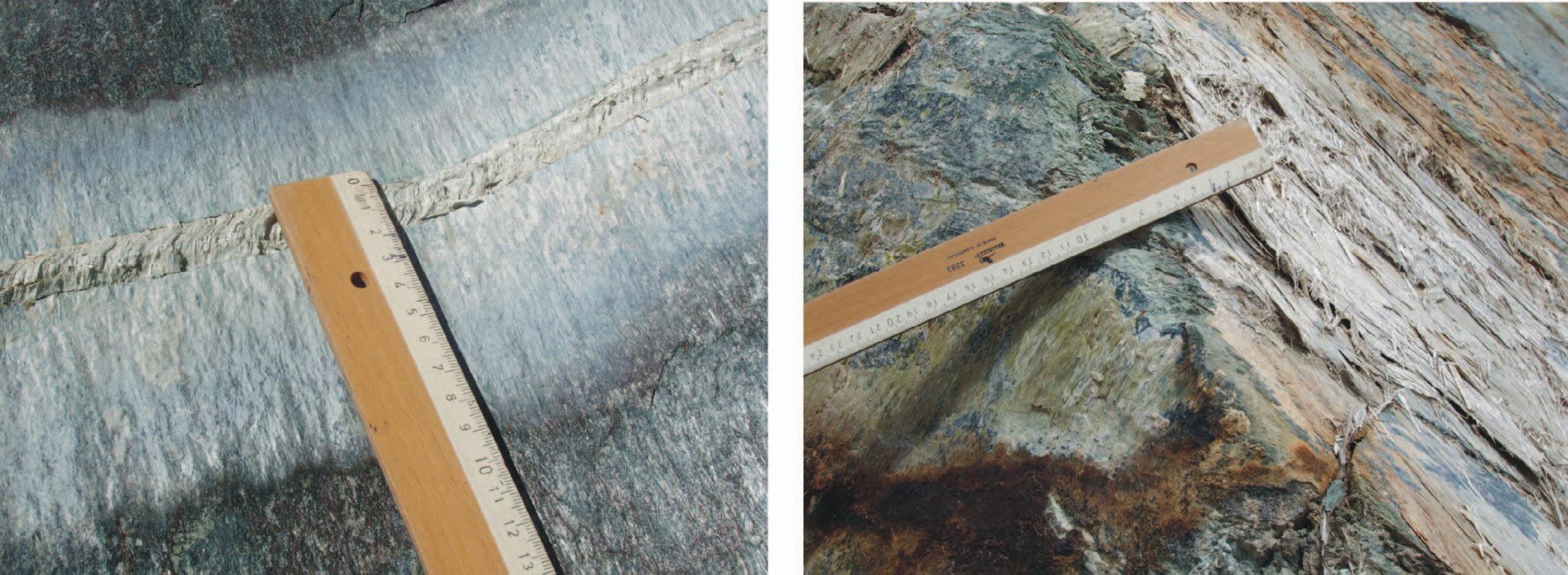
Figure 2. Cross-fiber (left) and slip-fiber (right) chrysotile vein with hydrothermal alteration selvedge.
Sampling Position Chrysotile (f/l, Mean and Range) Tremolite (f/l, Mean and Range)
Quarry, moving and handling 22.3 (<0.5–72) Quarry, drilling and blasting 28.6 (0.5–160) Quarry, diamond wire cutting 21.2 (<0.5–>200) Quarry border 0.6 (<0.3–5.3) Nearest town (1–5 km) 0.2 (<LOD–0.5) Processing: gang saw cutting 51.3 (0.5–>>200) Processing: hand splitting 3.3 (0.5 – 10) Processing: dry finishing 24.3 (1.9 – 92)
<LOD <0.1 (<LOD–0.5) <0.1 (<LOD–3) <LOD <LOD <LOD <LOD <LOD
for sampling and phase-contrast microscopy analysis (PCM), whereas SEM-EDS analysis was performed according to national legislation (Ministerial Decree 257, 1994) and Method 14966 of the International Organization for Standardization (2002). TEM analysis was carried out according to Method 7402 of the National Institute for Occupational Safety and Health (1994). The fiber definition criteria are those cited in the references above (length >5 µm, diameter <3 µm, and aspect ratio >3:1). Cellulose nitrate filters were used for PCM, whereas polycarbonate filters were used for SEM-EDS and TEM analyses.
A total of 140 rock, 38 soil, and 27 stream sediment samples (each 1.5–2 kg) were collected and characterized for NOA concentration. A preliminary evaluation of rocks and mineralized vein samples was carried out with a handheld Enspectr RaPort Raman instrument, equipped with a 532-nm laser at maximum output power of 30 mW, with a real spatial resolution in the range of a few cubic millimeter. Raman spectra were collected in a single recording up to 60 seconds, in the extended wavenumber range 120–4,000 cm −1 , with a spectral resolution of 8 cm −1 . The bulk mineralogy (limit of detection between 0.5 and 2 wt%, depending on crystallinity) was determined by X-ray powder diffraction using a Bragg–Brentano θ–θ PANalytical X’Pert PRO PW3040/60 diffractometer, and quantitative phase analysis was carried out running FULLPAT software (Chipera and Bish, 2002). Quantitative asbestos determination was carried out by SEM-EDS, following specific technical criteria for NOA (Italian D.Lgs. 152/06; Gualtieri et al., 2014, 2018; Belluso et al., 2017).
Airborne Asbestos
The analysis of airborne asbestos showed a complex environment, with significant analytical issues. The PCM data show quite low concentrations and poor agreement with SEM and TEM due to the abundance of very thin fibrils and/or fiber bundles, deriving from strong mechanical fragmentation and comminution (e.g., drilling, blasting, and diamond wire cutting), invisible under PCM (resolution 0.3 µm). Due to the abundance of lamellar antigorite with a high aspect ratio (especially schistose varieties), filters were heavily loaded with pseudo-fibrous antigorite splinters, falling into the WHO fiber definition. The distinction between chrysotile and pseudo-fibrous lamellar serpentine was based on both morphological and dimensional criteria (e.g., a cutoff diameter of 0.2 µm; Cattaneo et al., 2012; Belluso et al., 2017). TEM data showed good agreement with SEM, highlighting in some cases the abundance of chrysotile micro-fibrils (length <5 µm), not countable according to WHO criteria. Chrysotile and pseudo-fibrous lamellar serpentine were detected in almost all samples, whereas asbestiform tremolite was observed in very few cases. The assessed occupational exposure levels in quarries (Table 1) were mainly below 100 f/l (mean values 15–30 f/l), with the exception of drilling, diamond wire cutting, and block handling. Occupational exposure levels appeared to
Table 2. Asbestos concentrations (mean and range) in “massive” samples by SEM-EDS. Limit of detection (LOD) is approximately 50 ppm.
Material Chrysotile (ppm) Tremolite (ppm)
Serpentinite, finished product 80 (<LOD–250) <LOD Quarry waste 460 (120–1,700) 60 (<LOD–150) Processing waste 270 (60–2,300) <LOD Soils 310 (150–2,800) <60 (<LOD–180) Stream sediments 220 (60–560) <60 (<LOD–480) Chrysotile mine tailings* 2.3 wt% (0.2–5.6 wt%) 230 (80–750)
*For chrysotile in mine tailings, the value is given in wt%.
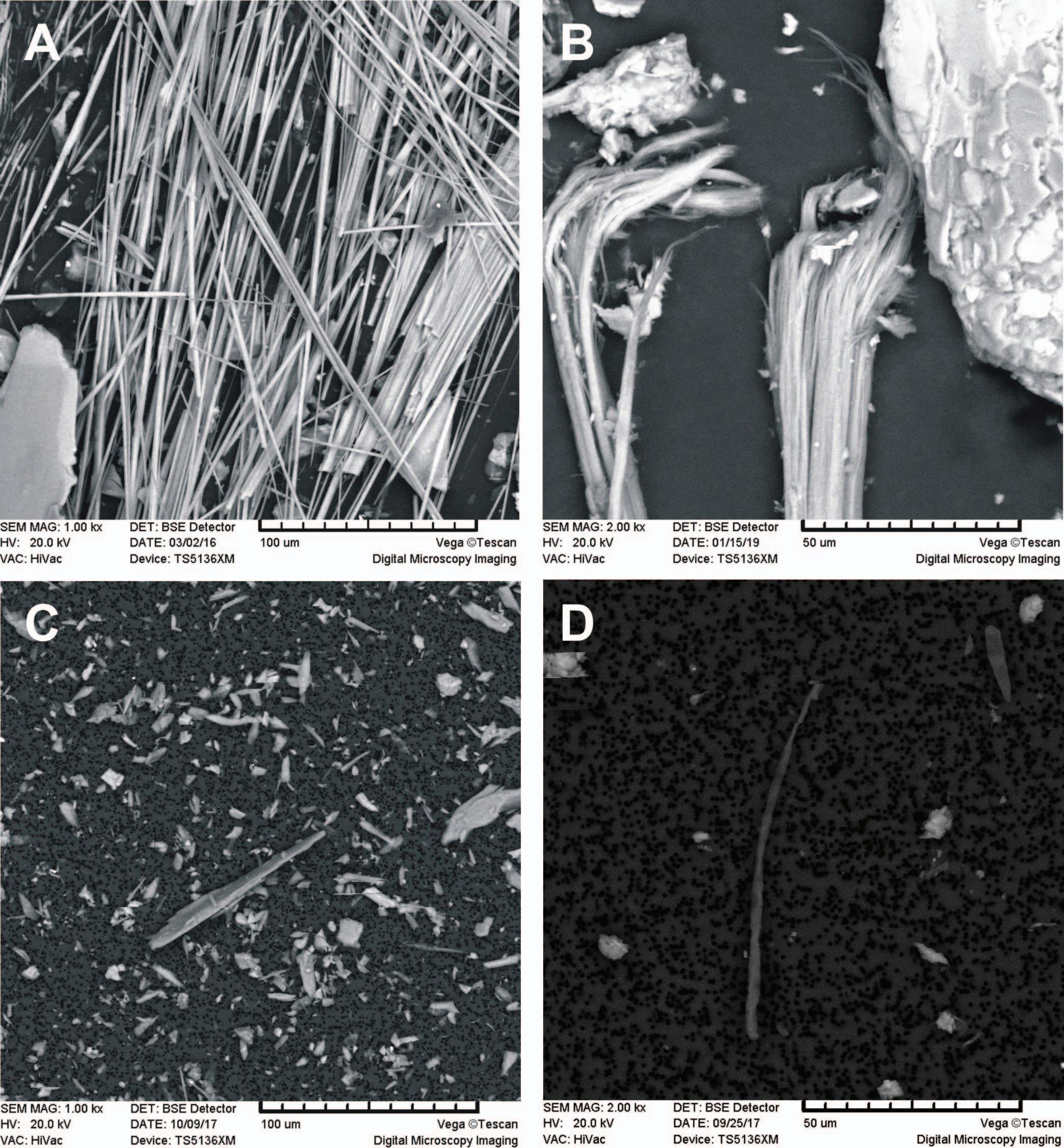
Figure 3. SEM back-scattered electron images. (A) Asbestiform tremolite. (B) Chrysotile fiber bundles. (C) Abundant lamellar, pseudo-fibrous antigorite fragments. (D) Organic fiber.
be linked with the interception of chrysotile veins, which in some cases coincide with quarry block external faces. Airborne monitoring in processing facilities identified some critical fiber exposure in the process of dry finishing and multi-blade gang saw cutting of blocks (values >>100 f/l). This can be explained by the chrysotile contamination of some external quarry block faces, emphasizing the importance of performing block squaring off directly in the quarry, removing all visible asbestos mineralization and accumulating
asbestos minerals in a safe area of the quarry for subsequent disposal. The preventive measures taken (e.g., quarry floor wetting, wet drilling, and block squaring) have proven to be effective because the airborne asbestos values have shown a statistically significant decrease over the years. Values measured at quarry property borders and at the nearest town (1–5 km from the quarry) were mostly below the environmental exposure limit (2 f/l), confirming scarce dispersion of the fibers from the extraction sites.
Rocks, Soils, and Stream Sediments
The evaluation of NOA contamination of rock masses requires detailed structural and petrographic surveys, supported by mineralogical analyses. The portable Raman device was a user-friendly efficient analytical strategy. The Raman device is able to discriminate the potential asbestiform fibers from non-harmful fragments (Petriglieri et al., 2015), especially for preliminary evaluation of rock debris, veins, and visible fibrous material. The rock mass quality in serpentinite quarries was always observed to be good, with chrysotile veins occurring systematically along specific discontinuities (fractures and faults). Hydrothermal alteration selvedges of chrysotile veins pose a problem, where contamination occurs up to 20 cm from the edge of the veins (Figure 2). The quantitative analysis of “massive” samples (SEM) requires special care, especially for sample selection and preparation (ensuring collection of a representative sample), and discrimination between asbestiform minerals and nonasbestiform polymorphs (e.g., pseudo-fibrous antigorite splinters). The commercial finished rock products (both “massive” and schistose serpentinite; Table 2) can be considered virtually asbestos free (in almost all cases no detectable asbestiform fibers), whereas special care must be taken with raw quarry blocks, sometimes with visible slip-fiber chrysotile mineralization. In some cases, the contamination of quarry floors and tracks is a concern due to the continuous accumulation of chrysotile debris and the fragmentation linked to the passage of trucks and excavators.
Small amounts of chrysotile (mean <400 ppm, range 150–2,800 ppm) were detected in soils and stream sediments, as were traces of asbestiform tremolite (mean <300 ppm, range <100–480 ppm). The highest concentrations of chrysotile were detected close to the abandoned mines, with peaks (up to 5 wt%; Table 2) in tailings, debris, and reclaimed mining landfills. While the presence of chrysotile was expected, asbestiform tremolite was a disturbing and widespread surprise (Figure 3): it is not reported in literature and is probably linked to talc veins and/or ophicarbonates occurring in both serpentinites and dolomitic marbles. Therefore, in-depth studies are needed to ascertain the extent of tremolite in the area.
CONCLUSIONS
The over 10-year experience in quarries and mines of the NOA environment in Valmalenco has highlighted the importance of the following: geological and structural field surveys, representative sampling, and accurate mineralogical analysis user-friendly analytical techniques (i.e., portable Raman) for easy and accurate preliminary evaluation of rock masses and fibrous material the definition of specific criteria and the combination of analytical techniques (e.g., SEM and TEM) for the correct identification, discrimination, and quantification of asbestiform minerals and nonasbestiform polymorphs or varieties in both airborne and “massive” samples the adoption of procedural and organizational solutions both in the quarries and in the processing facilities to easily recognize and reduce contamination the need of appropriate training of employers and workers under coordinated supervision of the local authorities (e.g., workers’ compensation authority) and the need for using the correct personal protective equipment
REFERENCES
Belluso, E.; Cavallo, A.; and Haltermann, D., 2017, Crystal habit of mineral fibers. In A. F. Gualtieri (Editor), Mineral Fibres: Crystal Chemistry, Chemical—Physical properties, Biological Interaction and Toxicity: EMU Notes in Mineralogy, 18. European Mineralogical Union and Mineralogical Society of Great Britain and Ireland, London, pp. 65–110. Cattaneo, A.; Somigliana, A.; Gemmi, M.; Bernabeo, F.; Savoca, D.; Cavallo, D. M.; and Bertazzi, P. A., 2012, Airborne concentrations of chrysotile asbestos in serpentine quarries and stone processing facilities in Valmalenco, Italy: Annals of Occupational Hygiene, Vol. 56, No. 6, pp. 671–683. Cavallo, A., 2018, Serpentinitic waste materials from the dimension stone industry: Characterization, possible reuses and critical issues: Resources Policy, Vol. 59, pp. 17–23. Cavallo, A. and Rimoldi, B., 2013, Chrysotile asbestos in serpentinite quarries: A case study in Valmalenco, Central Alps, Northern Italy: Environmental Science: Processes & Impacts, Vol. 15, pp. 1341–1350. Chipera, S. J. and Bish, D. L., 2002, FULLPAT: A full-pattern quantitative analysis program for X-ray powder diffraction using measured and calculated patterns: Journal of Applied Crystallography, Vol. 35, No. 6, pp. 744–749. Gualtieri, A. F.; Bursi Gandolfi, N.; Pollastri, S.; Rinaldi, R.; Sala, O.; Martinelli, G.; Bacci, T.; Paoli, F.; Viani, A.; and Vigliaturo, R., 2018, Assessment of the potential hazard represented by natural raw materials containing mineral fibres—The case of the feldspar from Orani, Sardinia (Italy): Journal of Hazardous Materials, Vol. 350, pp. 76–87.
Gualtieri, A. F.; Pollastri, S.; Bursi Gandolfi, N.; Ronchetti, F.; Albonico, C.; Cavallo, A.; Zanetti, G.; Marini, P.; and Sala, O., 2014, Determination of the concentration of asbestos minerals in highly contaminated mine tailings: An example from abandoned mine waste of Crètaz and Èmarese (Valle d’Aosta, Italy): American Mineralogist, Vol. 99, pp. 1233–1247. International Organization for Standardization, 2002, Ambient Air—Determination of Numerical Concentration of Inorganic Fibrous Particles—Scanning Electron Microscopy Method 14966: International Organization for Standardization, Geneva, Switzerland. Münterer, O. and Hermann, J., 1996, The Val Malenco lower crust-mantle complex and its field relations (Italian Alps): Schweizerische Mineralogische und Petrographische Mitteilungen, Vol. 76, pp. 475–500. Münterer, O.; Hermann, J.; and Trommsdorff, V., 2000, Cooling history and exhumation of lower-crustal granulite and upper mantle (Malenco, eastern Central Alps): Journal of Petrology, Vol. 41, pp. 175–200. National Institute for Occupational Safety and Health, 1994, Asbestos by TEM: Method 7402: Issue 2, August 15, 1994. Petriglieri, J. R.; Salvioli-Mariani, E.; Mantovani, L.; Tribaudino, M.; Lottici, P. P.; Laporte-Magoni, C.; and Bersani, D., 2015, Micro-Raman mapping of the polymorphs of serpentine: Journal of Raman Spectroscopy, Vol. 46, pp. 953–958. World Health Organization, 1997, Determination of Airborne Fiber Number Concentrations: A Recommended Method, by Phase Contrast Microscopy (Membrane Filter Method): World Health Organization, Geneva, Switzerland.