
16 minute read
Evaluating carbon reduction strategies for the University of Pittsburgh
Eli Brock a , Sabrina Nguyen a , Dr. Katrina Kelly-Pitou b , Dr. Robert Kerestes a a Electrical Engineering, University of Pittsburgh, Pittsburgh, PA b Dr. Katrina Kelly-Pitou was part of the Center for Energy at the University of Pittsburgh when the project kicked off, but now works for SmithGroup in Pittsburgh, PA
Eli Brock is a sophomore electrical engineering student. He is interested in power systems, mathematical modeling, and sustainable energy. He plans to pursue a PhD after graduating.
Eli Brock
Sabrina Nguyen is a senior electrical engineering student planned to graduate in the spring. Her interests lie in electric distribution systems, renewable energy, and sustainable energy practices. She plans to pursue a master’s degree in electric power systems and sustainability following her undergraduate career.
Sabrina Nguyen
Dr. Katrina Kelly-Pitou
Katrina Kelly-Pitou is an expert in energy systems and urban development. Currently, she acts as an Urban Systems Strategist at SmithGroup in Pittsburgh.Pulling together architects, landscape architects, engineers, and behavioral scientists, the Urban System’s group focuses on bridging the gaps between the technical and social development needed to future-proof cities across the globe. Katrina leads the interdisciplinary energy designs that are needed to transition society towards a zero-carbon future. In addition to her postdoctoral research in Electrical and Computer Engineering at the University of Pittsburgh, Katrina holds a PhD from the University of Nottingham in Energy Development Studies, a Masters in International Relations from Hult International Business School, and a BA/BSc from Duquesne University. She is a regular contributing to IEEE PES, IEEE Smart Cities, Energy Policy, and the European Economic Review.
Robert Kerestes received his Ph.D. from the Department of Electrical and Computer Engineering at the University of Pittsburgh in 2014. Prior to that he also received his M.S. (2012), and his B.S. (2010) all with a concentration in electric power systems at the University of Pittsburgh. Prior to his education, Robert served in the United States Navy as an interior communications electrician onboard the USS Constellation from 1998-2002 and then as a construction electrician in the Seabees Naval Construction Battalion from 2002-2006. Following his education, Robert worked at Emerson Automation Solutions (formerly known as Emerson Process Management) where he led the development and implementation of mathematical models for electric power applications related to embedded simulation. In 2016, Robert joined the University of Pittsburgh’s ECE Department where he focuses on the advancement of pedagogy and assessment in the field of electrical engineering, particularly in the areas of electric power distribution systems, distributed energy resources, and electromagnetism. Robert’s research interests are in the development of the next generation of electric power distribution systems and the integration of renewable energy resources into the distribution sector. Robert took on the role of Director of the Electrical Engineering Undergraduate Program in the Spring of 2017 and currently serves in that role. Robert Kerestes
Significance Statement
For an urban, cloudy campus like the University of Pittsburgh, unique factors come into play when deciding between carbon reduction strategies. For this university, this study finds on-campus, university-owned solar is a less effective initiative than green power purchase agreements.
Category: Computational research Keywords: urban, campus, electric generation, efficiency, solar, decarbonization
Abstract
The University of Pittsburgh has set goals to transition to renewable/low-carbon energy by 2030. As a large proportion of the University’s carbon emissions come from its purchased electricity, the purpose of this study is to explore methods Pitt could implement in order to reduce the emissions associated with its electricity consumption.
This study compares three main strategies for decarbonization: on-campus solar installations, power purchase agreements, and bulk purchases of renewable energy credits. The project expands on data collected by other researchers, specifically data regarding the location, placement, and potential output of solar panels on campus. This data served as the foundation for a costand-emission dual-optimized model, created by using Python to derive output efficiencies for each building and prioritize more efficient rooftops. Visualization with ArcMap and MATLAB facilitated comparison between the model and its alternatives. After assessing relative costs and emissions reductions, the analysis concludes that entering PPAs would be more financially viable than investing in solar power.
1. Introduction
In the University of Pittsburgh’s current Clean Energy Plan, there are two overarching goals. The first mandates that 50% of Pitt’s electricity be renewably sourced by 2030. The second calls for a 50% reduction in greenhouse gas emissions by 2030 from the 2008 baseline [1]. This analysis will focus on the latter goal because it more comprehensively addresses the challenge of carbon reduction.
Pitt is an urban school located in one of the cloudiest cities in the US. Lack of sunlight reduces the economic viability of solar panels; furthermore, urban areas have less room for solar panels and more shade from taller buildings. This university is not the only institution whose conditions are unfavorable for solar installation; however, with recent advances in photovoltaics, it seems possible that even installations in cloudy locations may offer cost-effective alternatives to traditional grid-paying fees. Therefore, a successful analysis of the production and cost impacts at Pitt can help to serve as a model for other universities in similarly cloudy locations. The University, like any climate-conscious economic actor, must weigh its benevolence against financial constraints and responsibilities. Therefore, the fundamental challenge of carbon reduction, and the purpose of this study, is to evaluate the cost efficiency of different strategies. Specifically, the decarbonization potential of the University’s purchasing power will be weighed against the prospects for on-site, university-owned solar generation. Producing energy on-site with university-owned assets offsets the cost of purchasing electricity but requires investment by the University. Conversely, purchasing green electricity simply requires restructuring electricity contracts, but Pitt must take care to ensure its actions are leading to emission reductions.
2. Methods
This study analyzes three alternatives for decarbonization of Pitt’s electricity consumption, which accounts for approximately half of its greenhouse gas emissions [2]. A HelioScope simulation conducted by university researchers in the summer of 2018 provides the foundation for analysis of the first alternative, onsite solar generation. Alternatively, the University could alter its purchasing practices to decarbonize its electricity consumption. While this approach could take several forms, this study will focus on two of the most common: entering into power purchase agreements (PPAs) with green power generation projects and purchasing voluntary renewable energy credits (RECs). A working model of the decarbonization potential and cost of solar panels will be juxtaposed with the price estimates for PPAs and RECs. 2.1 Working Solar Model
On-site solar generation, as a decarbonization strategy, can be simply but comprehensively modeled with a single metric: the annual reduction in carbon emissions associated with each dollar invested, in kilograms per dollar per year. As this is a marginal metric, it is given by a derivative:
(1)
where ME is marginal emissions avoided in E is total emissions avoided in and P is installation cost in $.
To go about finding E(P), the researchers began with the results of the HelioScope simulation. HelioScope is a solar panel simulation software which outputs data such as the panel capacity of a roof and its yearly energy output (in kWh), among other data. Due to location, shade, and other factors, some buildings are considerably more efficient than others (in other words, more energy production per installed watt). Assuming the University wants to optimize its investment, it should install panels on the most efficient buildings first. 2.2 Assumptions
Important assumptions were made in evaluating the efficiency of the buildings. In the University’s simulation, each building was simulated with one of three panels, with wattage ratings of either 320W, 310W, and 280W. Note that wattage ratings do not reflect actual output. As commercial solar installations are priced per installed watt, it is assumed that the wattage capacity per building is consistent; in other words, the number of installed watts is independent of which panel is used. This assumption is important because the University would likely purchase only a single panel model for all buildings. It is also assumed that all installed watts on the same rooftop are equally efficient.
2.3 Annual Energy Production of Each Installed Watt
With these assumptions, the data yielded a natural metric for the efficiency of a rooftop: Y W , the marginal increase in energy output associated with each installed watt. Y W is unique and constant for each building. Y W for a given building is given by:
where Y W is the energy output per installed watt in Y R is the energy output of the roof in kWh, W P is the respective wattage rating of the simulated panel, and N is the panel capacity of the roof.
2.4 Annual Energy Production as a Function of Watts Installed To find total annual energy production as a function of watts installed Y(N W ), the values of Y W were added for each consecutive watt, starting with the most efficient, until N W watts were reached. The value of Y W steps down each time N W exceeds the wattage capacity of the next most efficient building.
2.5 Total Emission Reductions as a Function of Investment E(P) was derived by scaling Y(N W ). The installation price is assumed to be $2/watt, per conversations with representatives of installation firms. The University’s emission factor (the mass of CO2 emitted per unit of electricity consumed) is 0.487546 kg/kWh as of 2017 [2]. Therefore,
(3)
2.6 Marginal Emissions Reductions as a Function of Investment ME(P), found by differentiating E(P), served as the model of on-site solar to be compared with other alternatives. As ME(P) is an annual figure, it was assumed for simplicity that the emissions factor will be constant through the foreseeable future; in reality, it is likely to decrease. 2.7 Working Market Price of Renewable Power-Purchase Agreements
An analysis of PPAs, specifically their prices, is difficult as their details tend to be confidential between the buyer and seller. A PPA is an agreement between an energy consumer, such as the University, and an energy generation project, usually one which has not been built yet. The consumer agrees to buy energy from the project for an agreed-upon price for an extended period. This price tends to be lower than the market price for electricity, thereby attracting the consumer, and the guaranteed demand for electricity draws in the project. These projects also tend to be renewable, usually wind and solar, as these sources can often offer electricity at a lower price and climate-conscious consumers create demand for them.
Given the difficulty of finding PPA price data, the researchers turned to the relatively transparent LevelTen PPA marketplace, which operates in areas served by five major independent system operators. Their 2019 Q1 PPA Price Index reports the 50th percentile price for wind and solar PPAs in these areas to be $29.50/MWh [3] (about 3 cents per kWh, compared with the University’s market price of 8 cents). It was assumed that LevelTen was approximately representative of the PPA market and this price was used for comparison with the other alternatives. While a rough estimate, it is consistent with other approximate PPA price indices, including the National Renewable Energy Laboratory’s Q1 2019 Solar Industry Update, which puts US solar PPA prices between 2 and 3 cents [4]. 2.8 Working Market Price of Voluntary Renewable Energy Credits
The final alternative, RECs, are the United States’ mechanism for establishing a green electricity market. One REC is created for each MWh of renewable electricity generated. The REC can then be bought and resold by utilities and consumers, and whoever “retires” the REC can claim to have used 1 MWh of renewable power. Many RECs are bundled with the electricity itself. Bundled RECs are transferred in PPAs and are also purchased and retired by utilities to ensure compliance with state Renewable Portfolio Standards (RPS), with the RPS/REC structure behaving as a kind of renewable subsidy. However, some RECs, often produced in states without RPSs, are known as “unbundled” or “voluntary” RECs. The price for voluntary RECs fluctuates but tends to be between $0.50 and $1.00 [5]. As a conservative estimate, $1.00 will be the price of unbundled RECs used for this analysis.
3. Results
Figure 1 is a map of approximately 70 buildings on the University of Pittsburgh’s Oakland campus, symbolized by economic efficiency (annual kWh production/$). This metric was derived by scaling E(P) by the inverse of the emission factor. As seen, there is a 28% difference between the most and least efficient buildings, a testament to the value of the building efficiency metric for decision-making.
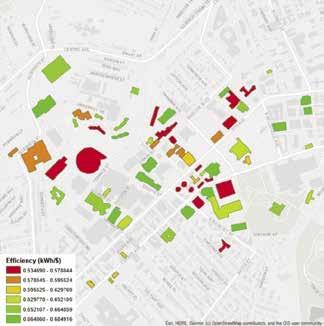
Figure 1: Pitt Buildings by potential solar efficiency
Figure 2 shows the annual carbon reduction per dollar spent as a function of installation cost, or ME(P) as derived in the “Methods” section. As would be expected, the marginal carbon reductions decrease as panels are installed on less efficient rooftops.
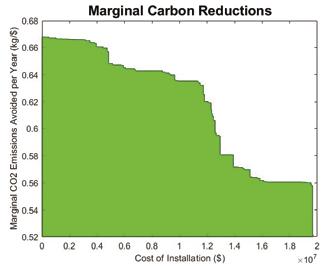
Figure 2: Marginal carbon reductions as a function of installation cost
University-owned solar, unlike other alternatives, represents an economic investment: for every unit of energy produced on-site, that unit does not need to be purchased from Duquesne Light Co., the utility servicing the University. As a result, over a certain time period, the panels will pay for themselves and save the University money. In Figure 2, the annual carbon reduction for any installation scale is given by the area under the curve up to the cost of installation. Dividing annual carbon reduction by the emissions factor and multiplying by the 8.1₵/kWh electricity price yields the annual savings by the university. Finally, dividing the installation cost by annual savings gives the payback period.
This process revealed that payback periods vary from 18 years to 23 years, with longer paybacks associated with larger-scale installation. Furthermore, even for a full-campus installation project, the annual energy production from on-site solar panels would max out at 12.5 million kWh, less than 6% of the University’s annual energy consumption [3].
Like on-site solar, power purchase agreements save the University money on a per-kWh basis; however, they do so without requiring an initial investment. The LevelTen price index, as established, is around 3₵/kWh and the University’s electricity costs are around 8₵/kWh, creating 5₵ of savings per kWh. By contrast, university-owned solar panels offset the entire cost—8₵—of purchased electricity. Accordingly, the monetary return per kWh associated with an average green PPA is around 60% of that associated with on-campus solar panels.
At the established price of $1, unbundled RECs would allow the University to claim 1 MWh of renewable energy, equivalent to 488 kg of emissions reductions per the 0.488 kg/kWh emissions factor. The same $1, invested in solar panels, would create only 0.67 kg of carbon reductions each year, assuming it was at the optimized end of the solar model (the far left of Figure 2). Even with this generous assumption, after the 25-year panel lifetime, that is only 16.75 kg of emissions reductions—29 times less than that of the unbundled REC.
However, most experts agree that unbundled RECs produce little to no additionality, meaning their purchase is unlikely to lead to new renewable generation capacity (this also explains their comparatively low price) [6]. As a result, while they may allow the University to claim environmental progress, their effectiveness as a decarbonization strategy is practically void.
4. Discussion
The most striking findings of the study are the two-decade payback period for distributed solar and its relatively small impact, with a maximum of 6% of current demand being met. PPAs, on the other hand, involve no initial investment and facilitate decarbonization on a much larger scale. In fact, the University will be getting 25% of its electricity from a PPA with a local hydropower plant starting in 2023. Considering these advantages, the lower yearly return is a worthwhile trade-off for PPAs.
Unbundled RECs, on the other hand, are an extremely cheap strategy to meet the University’s 50% renewable goal. However, in the context of the University’s second goal, which prioritizes carbon reduction instead of renewable penetration, purchasing voluntary RECs is not a viable solution. Directly purchasing renewable energy and the bundled RECs which accompany it leads to greater, but still imperfect, additionality. However, since they carry real market weight, bundled credits are more expensive. PPAs, since the project is unlikely to exist without the agreement, boast nearperfect additionality, as do on-site solar panels.
For reference, the University is currently engaged in purchasing-based sustainability initiatives. These include the aforementioned hydropower dam and REC purchases (bundled and unbundled) which equated 14% of Pitt’s energy consumption in financial year 2019. The solutions discussed in this study would, obviously, expand on these preexisting initiatives.
5. Conclusion
Several of the proposed solutions in this project are typical for institutions trying to restructure their energy profile. In the case of solar panels, the return on investment decreases as panels are added to inefficient buildings, becoming prohibitively low after a certain point. Furthermore, this investment would impact only a small proportion of the University’s total electricity consumption. Purchasing large quantities of RECs would change the University’s energy profile, but there would be little to no measurable reduction in carbon emissions because of the weak legal structure surrounding RECs. PPAs are more viable than onsite solar generation and more effective than RECs, thereby making them the best decarbonization strategy examined in this study.
As Pitt moves forward with sustainability initiatives, it will need to explore multiple strategies and implement the most viable ones
concurrently. This is especially true when considering solar panels: as detailed in the results, a full-scale solar installation project would represent only a fraction of the University’s energy profile. The inefficiency of such a solar installation relative to PPAs make them a poor investment on any scale. This study recommends that PPAs serve as the primary solution, and that any potential investment in rooftop solar panels be spent on more potent sustainability initiatives.
These other initiatives may include university-owned generation on a larger scale. For example, installing a solar park near campus would circumvent the building-by-building limitations and take advantage of the economies of scale which make PPAs so effective. Furthermore, advances in photovoltaics and associated drops in the price of solar panels will continue to make solar generation more affordable and accessible.
6. Acknowledgements
Funding was provided through the SSOE Summer Research Internship with advisors Dr. Robert Kerestes and Dr. Katrina Kelly. We would also like to thank Dr. Aurora Sharrard, who met with us to go over the specifics of the University’s decarbonization landscape.
7. References
[1] 2018 Pitt Sustainability Plan. University of Pittsburgh. 12.5.2017. Accessed 8.20.2019. https://issuu.com/upitt/ docs/2018_pittsustainabilityplan_final.
[2] M.M. Bilec, H. Gardner, Greenhouse Gas Inventory of University of Pittsburgh for FY 2017. 5.29.2018. Accessed 10.25.2019.
[3] LevelTen Energy Q1 2019 PPA Price Index. 5.8.2018. Accessed 10.25.2019. https://leveltenenergy.com/blog/ppa-priceindex/q1-2019/ .
[4] P4 2018/P1 2019 Solar Energy Update. NREL. 5.2019. Accessed 10.25.2019. https://www.nrel.gov/docs/fy19osti/73992. pdf.
[5] E. O’Shaughnessy, J. Heeter, J. Sauer. Status and Trends in the U.S. Voluntary Green Power Market (2017 Data). NREL. 10.2018. Accessed 10.25.2019. https://www.nrel.gov/docs/ fy19osti/72204.pdf.
[6] D. Roberts. “It’s easy to buy ‘green power.’ Making a difference is a little harder.” Vox. 11.6.2015. Accessed 10.25.2019. https://www.vox.com/2015/11/16/9744620/supportrenewable-energy.