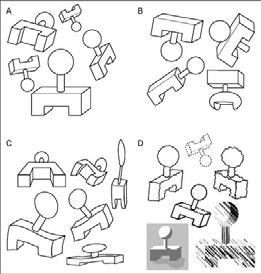
6 minute read
Constancia perceptiva y proporción aparente
Retomamos el tema de la invariancia perceptiva, que ya abordamos hace años, y su relación con el modo en que juzgamos las relaciones geométricas aparentes de los objetos
Cuando nuestra retina capta la imagen de un objeto, genera una señal que envía al cerebro para ser interpretada. Una idea simplista de la visión concebiría la visión como el resultado de un análisis sistemático de las propiedades geométricas de esa imagen (longitudes de los trazos, ángulos que forman, polígonos que describen, etcétera), para deducir de qué forma se trata. Pero si el objeto está en movimiento (por ejemplo, si lanzamos al aire una botella haciendo molinetes), se generarán toda una serie de imágenes geométricamente muy diversas. Y a pesar de ello, nuestra visión identifica sin problema, de forma continua e instantánea, el objeto como el mismo y no otro.
Es un ejemplo del principio de invariancia o constancia perceptiva de la forma, que ilustra la figura 1, y viene a revelar que nuestra visión se guía por un “sentido común”, aprendido a lo largo de nuestra evolución como especie: las cosas no se convierten en otras al lanzarlas al aire (en general), aunque su imagen en la retina cambie constan-
1
Prof. Rafael I. Barraquer Oftalmólogo Ophthalmologist
Figura 1. Un simple vistazo nos convence de que el grupo A está formado por copias del mismo objeto en diferentes posiciones y tamaños, al tiempo que identificamos los del grupo B como diferentes, pese a compartir ciertos elementos geométricos comunes. El reconocimiento de la identidad del objeto resiste también a deformaciones “elásticas” (grupo C) y a variaciones en el tipo de trazo o sombreado (grupo D). Se trata del principio de “invariancia” perceptiva, definido por la escuela de la Gestalt (imagen de S. Lehar, 2003).
Figure 1. A simple glance convinces us that group A is made up of copies of the same object in different positions and sizes, while at the same time we identify those in group B as different, even though they have certain geometric elements in common. The recognition of the identity of the objects also withstands “flexible” deformations (group C) and variations in the type of line or shading (group D). This is the principle of perceptual “invariance”, defined by the Gestalt school (image by S. Lehar, 2003).
Perceptual constancy and apparent proportions
We’re revisiting the topic of perceptual invariance, which we looked at some years ago, and its relationship with the way we judge the apparent geometric relationships of objects
Figura 2. En el dibujo original de Shepard (izda.), las mesas parecen claramente diferentes, pero si medimos sus lados mayor y menor (der.) comprobamos que los rectángulos son idénticos. Lo mismo ocurre si recortamos el sobre de una mesa y lo transportamos sobre la otra. When our retina captures the image of an object, it generates a signal that it sends to the brain to be interpreted. A simplistic idea of the vision would view it as a result of a systematic analysis of the geometric properties of that image (lengths of the lines, angles they form, polygons they describe, etc.), in order to deduce what shape it is. However, if the object is moving (for example, if we throw a bottle up in the air and it spins around), a whole series of very geometrically different images is produced. And despite that, our vision perfectly identifies—both continually and instantaneously—the object as it is and not as another.
It’s an example of the principle of invariance or perceptual constancy of a shape, shown in figure 1, and it reveals that our vision is guided by “common sense”, learnt throughout our evolution as a species: things do not become others once thrown in the air (in general), even though their image in the retina constantly changes. But this same principle can lead to illusions when we play with other aspects like perspective. One of the most well-known perspectives
2
Figure 2. In Shepard’s original drawing (left), the tables look vastly different, but if we measure their longest and shortest sides (right) we can confirm that the rectangles are identical. The same thing happens if we cut out the top of a table and we place it on top of the other.
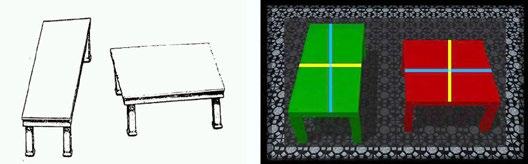
3
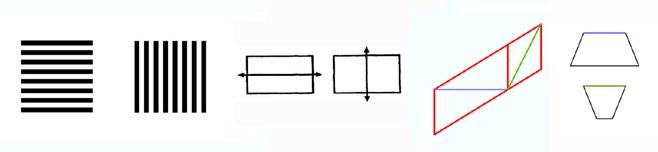
Figura 3. De izquierda a derecha: ilusiones de los cuadrados rayados (Helmholtz), de los rectángulos cruzados (Perelman), de las diagonales (Luckiesh y Sander) y de los trapecios. Figure 3. From left to right: lined squares illusion (Helmholtz), crossed rectangles illusion (Perelman) and diagonals illusion (Luckiesh and Sander) and the trapezoids illusion.
temente. Pero este mismo principio puede dar lugar a ilusiones cuando se juega con otros aspectos como la perspectiva. Una de las más conocidas es la de las mesas de Roger N. Shepard, psicólogo americano que hemos frecuentado en estas páginas (figura 2).
En realidad, nuestra percepción no se equivoca ya que, aunque los rectángulos en el dibujo sean iguales, las mesas vistas así en perspectiva serían diferentes en el mundo real. Esto confirma que nuestro cerebro no elabora la visión de forma maquinal, sino que crea una representación verídica comparando las imágenes retinianas con nuestro conocimiento previo de los objetos y las claves que vemos de su situación en el espacio tridimensional.
Otras ilusiones revelan variantes del mismo tema (figura 3). En los cuadrados rayados de Helmholtz (1866), el lado vertical parece mayor que el horizontal cuando está relleno de una rejilla horizontal, y viceversa. Los rectángulos cruzados de Perelman (1913) son idénticos, pero el primero parece más largo y estrecho que el otro, un efecto relacionado con la flecha que lo cruza, ya sea a lo largo o de forma transversal. Las diagonales (azul y verde) dentro de paralelogramos (Luckiesh y Sander, 1922) parecen claramente diferentes, pero en realidad son iguales, lo mismo que ocurre con el lado superior de los trapecios enfrentados de la derecha de la figura. Como ocurre con muchas ilusiones cognitivas geométricas, su aparente simplicidad esconde aspectos profundos de los mecanismos de la percepción visual. Dediquemos un tiempo a reflexionar sobre sus posibles explicaciones. ■ is tables by Roger N. Shepard, an American psychologist who we have previously addressed in this section (figure 2).
In fact, our perception is not mistaken as, though the rectangles in the drawing are the same, the tables, when seen like from that perspective, would be different in the real world. This confirms that our brain does not produce vision in a mechanical way; instead, it creates a truthful representation comparing the retinal images with our previous knowledge of objects and the keys we see in their situation in three-dimensional space.
Other illusions reveal variants of the same subject (figure 3). In Helmholtz’s lined squares (1866), the vertical side looks larger than the horizontal side when it is filled with horizontal bars, and vice versa. Perelman’s crossed rectangles (1913) are identical, but the first one looks larger and narrower than the other, an effect related to the arrow through it, be it longways or transverse. The diagonal arrows (blue and green) inside the parallelograms (Lukiesh and Sander, 1922) look clearly different, but in fact they are the same; the same thing that happens with the upper side of the trapeziums facing one another on the right of the figure. As occurs with many geometric cognitive illusions, their apparent simplicity conceals profound aspects about the mechanisms of visual perception. Let’s dedicate time to reflecting on their possible explanations. ■
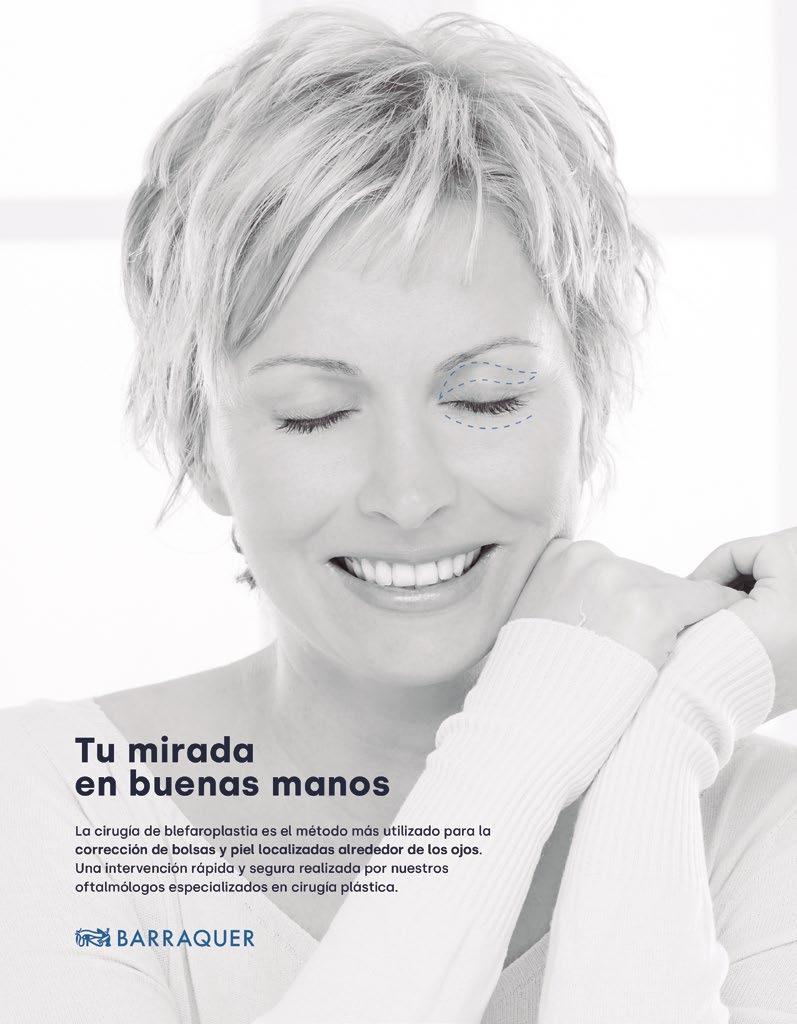
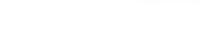
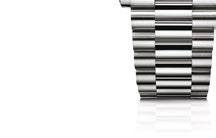
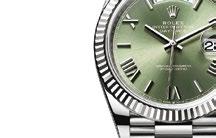


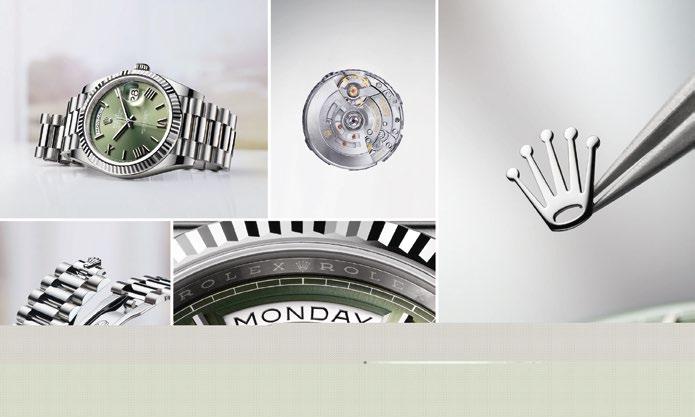