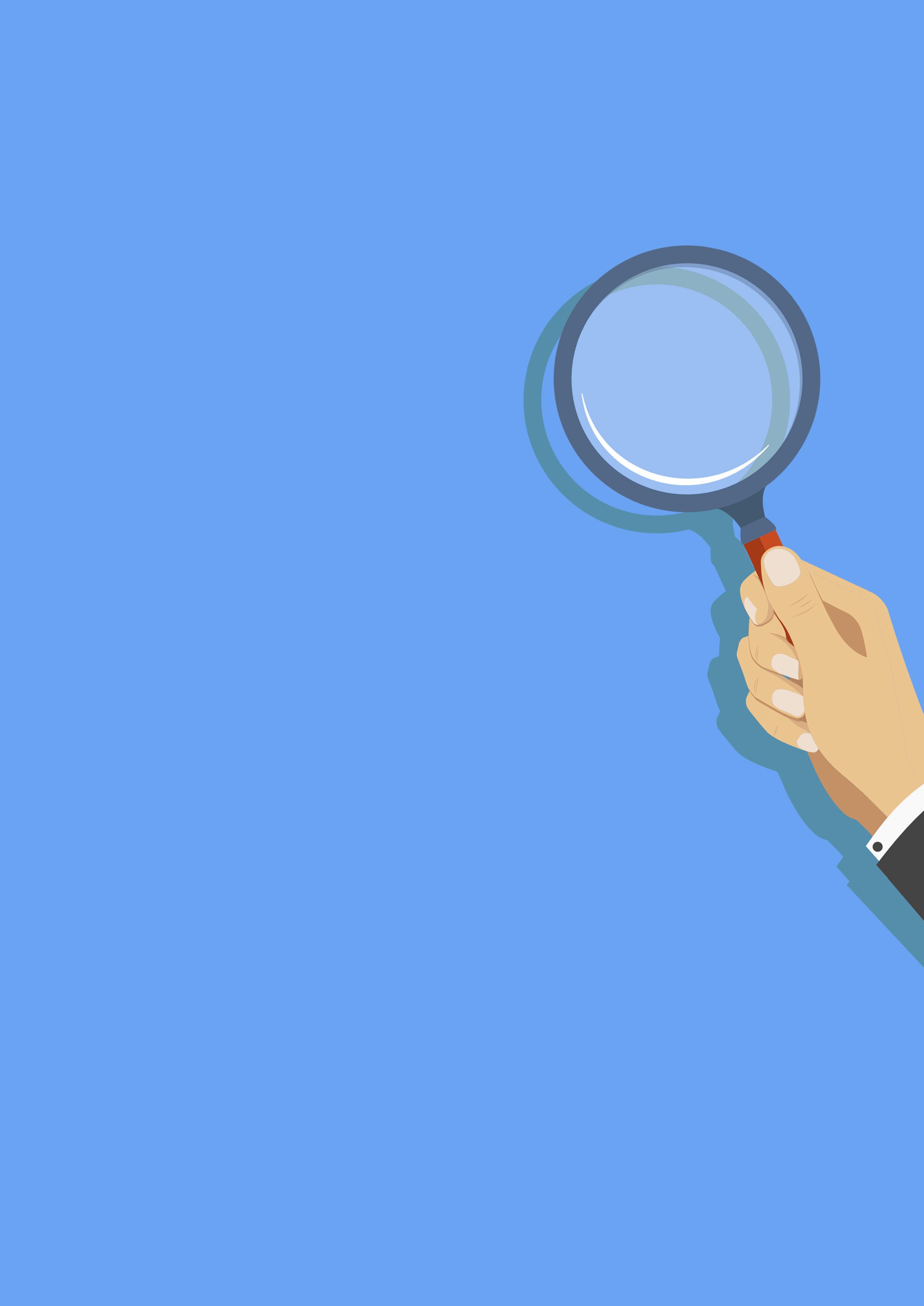
12 minute read
An inside look at blue hydrogen
Using key insights from the first wave of blue hydrogen projects, Pavan Chilukuri, Shell Catalysts & Technologies, the Netherlands, discusses the current status of blue hydrogen, and its role within the ongoing energy transition.
The International Energy Agency (IEA) has urged governments and companies to “seize the opportunity” presented by hydrogen as momentum behind this clean-burning fuel reaches unprecedented levels. More than 35 governments have, or are working towards, national hydrogen strategies, which reflects the potential for low-carbon hydrogen to significantly reduce carbon dioxide (CO2) emissions while contributing to energy security.
By 2050, low-carbon hydrogen is forecast to supply as much as 10% of all final energy consumption around the world.1 However, most current hydrogen production processes emit large quantities of CO2. That being said, hydrogen production does not have to be carbon intensive – a fact that is growing in importance as demand for low and zero-carbon hydrogen intensifies.
In 2020, Shell launched the Shell Blue Hydrogen Process (SBHP) which enables hydrogen to be produced from a variety of fossil fuel and renewable feedstocks with an almost 100% carbon capture rate, and offers many advantages over conventional hydrogen production and carbon capture technologies. 18 months on, the organisation has learned a great deal from the first wave of blue hydrogen projects.
This article will share what Shell has discovered to help advance this fast-moving and growing decarbonisation solution. The organisation has gleaned insights from more than 50 projects around the world to determine where the blue hydrogen market sits today, and how its technology can further advance low-carbon hydrogen production.
The hydrogen market
Demand for hydrogen is accelerating, driven by stronger national-level commitments to decarbonise energy systems, and by industrial actors seeking to align themselves with increasingly strict greenhouse gas (GHG) emission regulations.
Today, the IEA estimates that the annual demand for hydrogen is approximately 90 million t; by 2030, this figure is forecast to reach around 200 million t, and over 500 million t by 2050.
Meeting this demand will require an unparalleled transformation of how hydrogen is produced. Currently, most hydrogen is grey, and produced by converting natural gas into hydrogen and unabated CO2 by the steam methane reforming (SMR) process. However, this process is carbon intensive and is, according to the IEA, responsible for as much as 900 million tpy of CO2 emissions.2 As such, the energy industry cannot simply expand current grey hydrogen production if it is serious about achieving deep decarbonisation. Instead, it must rapidly transition to cleaner methods of hydrogen production, such as green and blue hydrogen (or even pink, purple, red, turquoise or yellow hydrogen – a full spectrum of hydrogen technologies that warrants an article of its own).
The latest insights from the Hydrogen Council show that the number of announced hydrogen projects rose by almost 60% in 1H21 to 359 large-scale projects worldwide – a 450% increase since the end of 2019. A global investment of US$500 billion has already been committed to low-carbon (blue) and zero-carbon (green) hydrogen projects through to 2030; this figure is set to rise as demand for cleaner hydrogen grows. Although Europe accounts for 80% of new projects, China is a rapidly emerging market with more than 50 announced new projects.
With these investments, green and blue hydrogen production capacity is set to exceed 10 million tpy by 2030: 70% of the installed capacity will be green and 30% will be blue.³ This is, however, far below the demand forecast for 2030, which leaves a considerable need for further projects and investments. That said, there are still uncertainties surrounding the relative growth of green and blue hydrogen production throughout the next decade, but hydrogen production costs will clearly be a big influence.
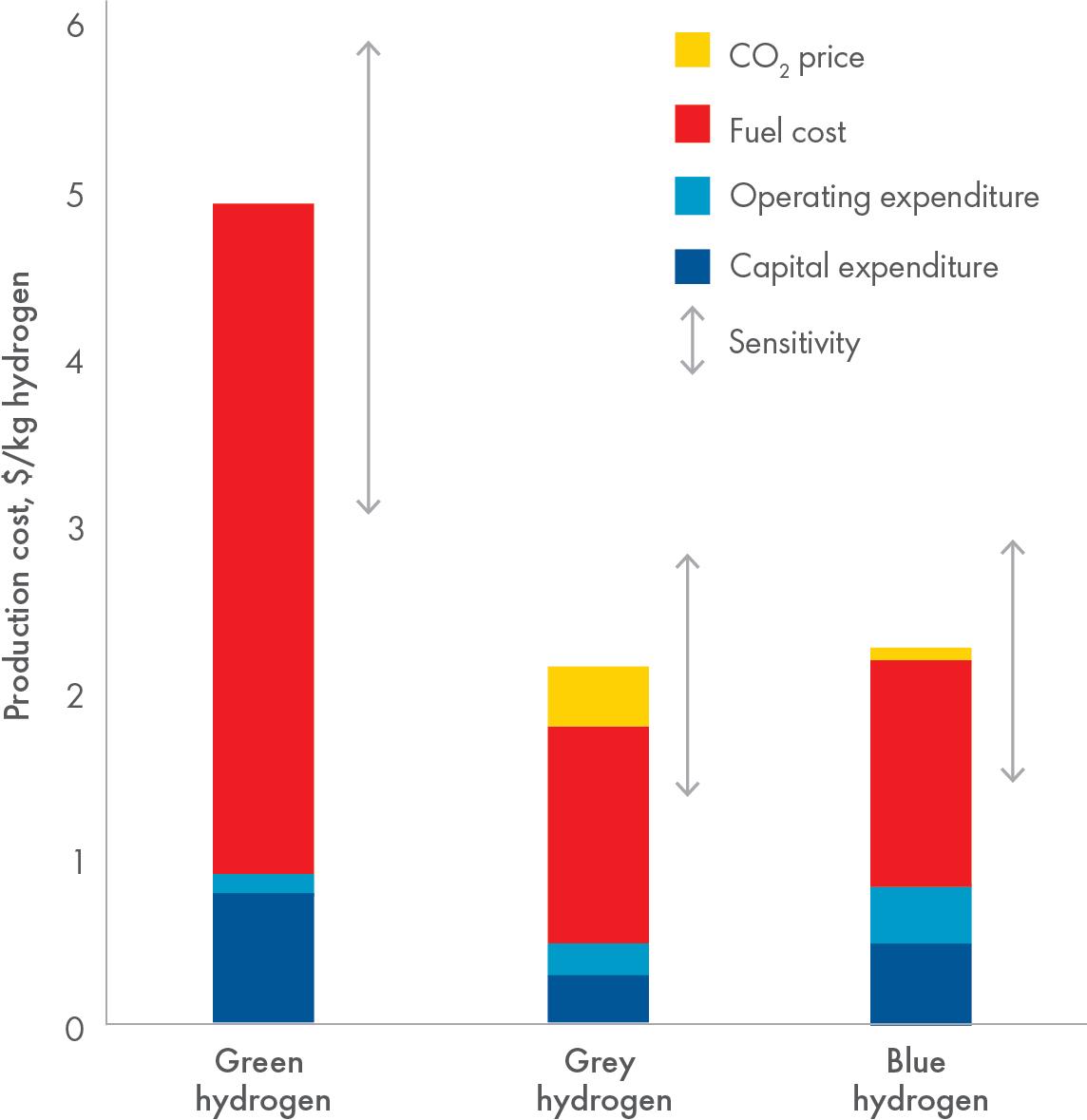
Figure 1. Hydrogen production costs in 2030.
The role of blue hydrogen
Blue hydrogen is similar to grey hydrogen except that the CO2 is captured and either utilised or stored underground. Although the amount of CO2 captured varies according to the project, blue hydrogen is widely regarded as low carbon. Green hydrogen is carbon free and is seen, in many situations, as the ideal solution to satisfy future hydrogen demand. So, why do we need blue hydrogen?
The reality is that most green hydrogen is currently much more expensive to produce than blue hydrogen. In fact, by 2030, forecasts indicate that green hydrogen may still be
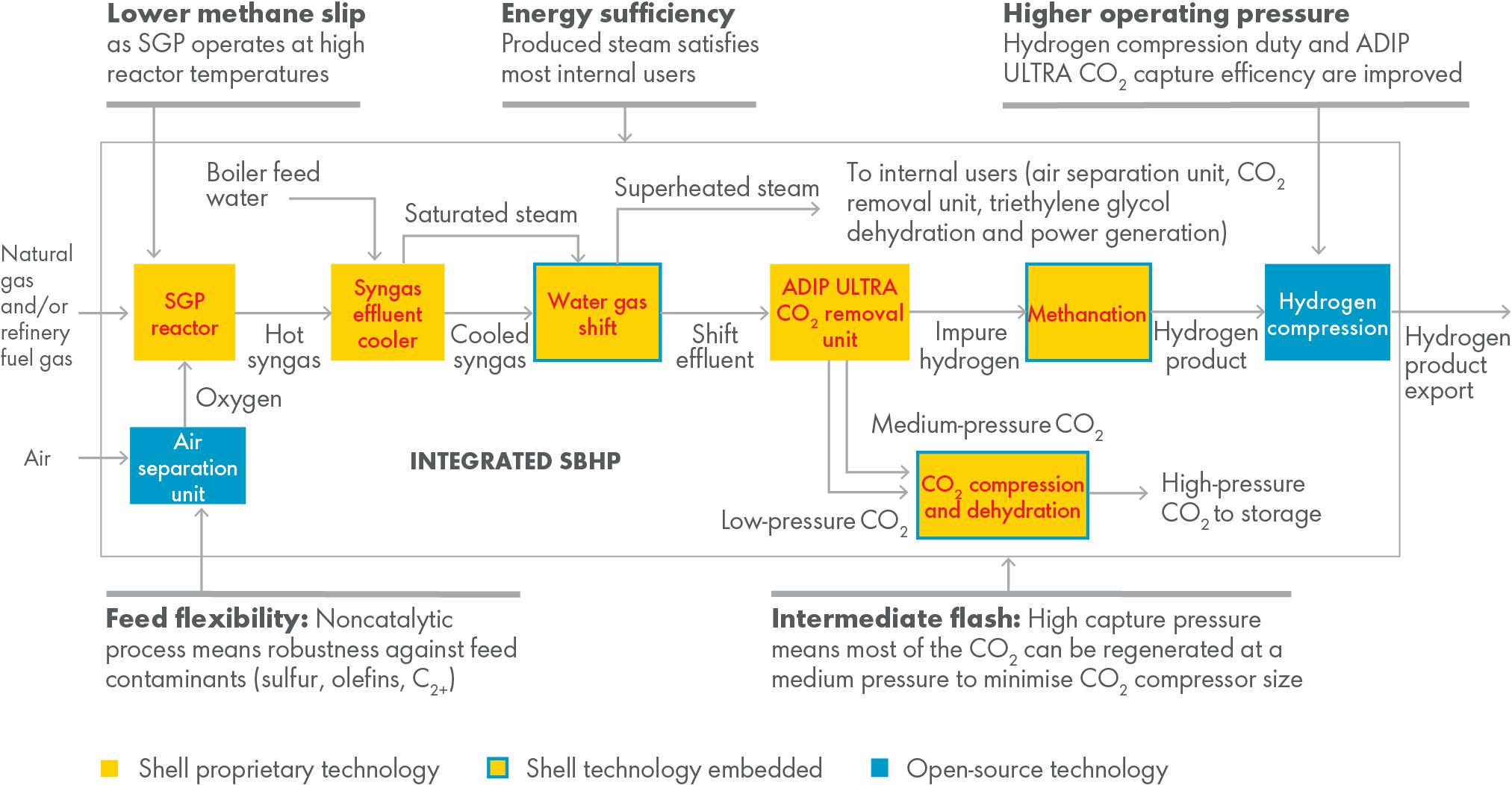
Figure 2. The SBHP and the advantages of integration with other technologies.
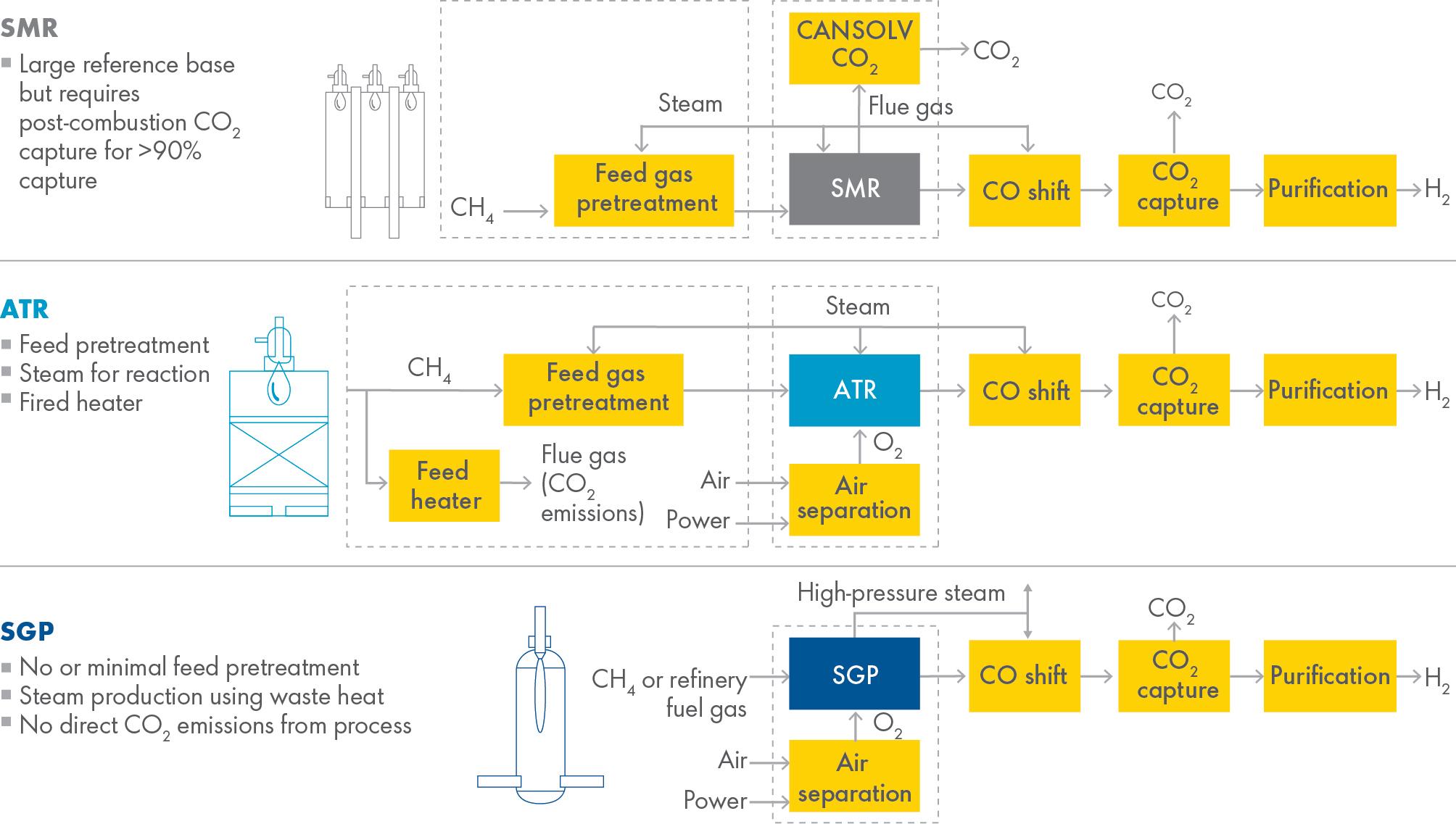
Figure 3. Comparison of the different shades of blue hydrogen technology.
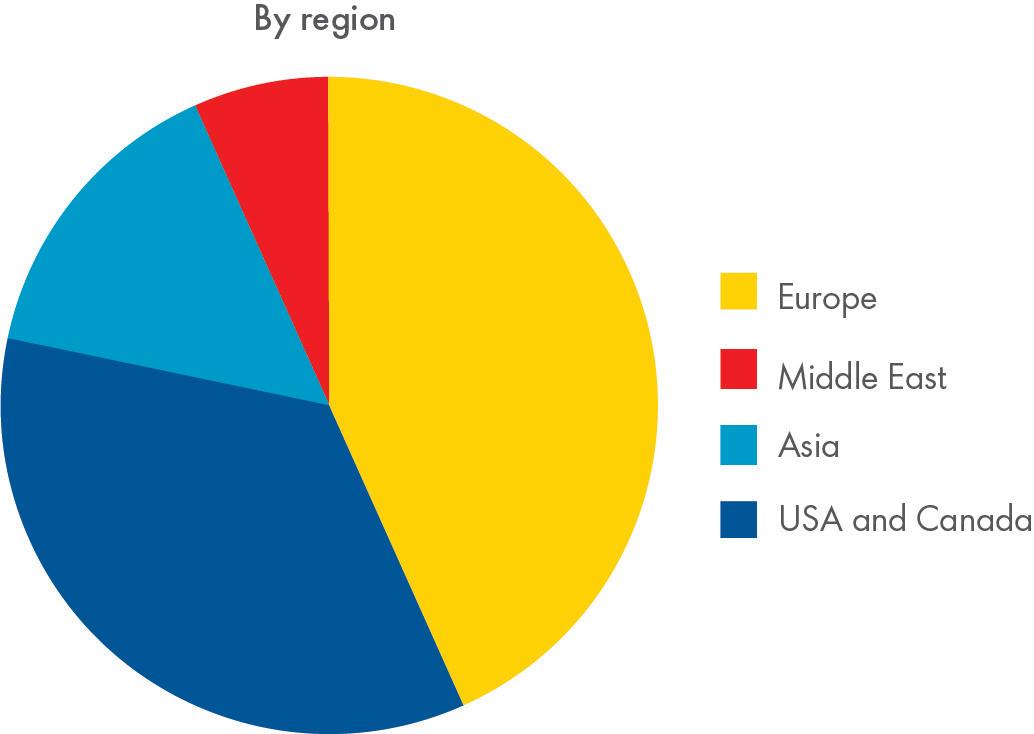
Figure 4. Location of the blue hydrogen projects studied.
double the price of blue hydrogen (see Figure 1) and that cost parity will not occur until about 2040. This will not be the case everywhere; in some regions, particularly those with already-developed renewable energy infrastructure, green hydrogen may already have the advantage. Where this is not the case, blue hydrogen has a key role to play.
To meet the growing demand for hydrogen and renewable power, blue hydrogen can provide an interim solution that can help to establish the hydrogen market while still lowering carbon emissions. Some, however, have queried the impact of blue hydrogen on the environment, as demonstrated in a recent paper by Cornell and Stanford Universities.⁴ However, this report is based on global assumptions that generate general conclusions, so should be treated with caution. A project-specific, case-by-case analysis of blue hydrogen projects will generate very different results, and Shell remains confident that blue hydrogen has a vital role to play in the energy transition.
Blue hydrogen will also play an important role in Shell becoming a net-zero-energy business by 2050 – a transition that requires reductions in both its own GHG emissions (Scope 1 and Scope 2) and those of its customers (Scope 3). Establishing a strong hydrogen market in preparation for the widespread introduction of green hydrogen will be key.
Which shade of blue hydrogen technology?
Blue hydrogen comes in different ‘shades’ according to the technology used. Until recently, project developers usually had the choice of two established blue hydrogen technologies: SMR or autothermal reforming (ATR). Now, there is a third option – one with the potential to provide superior cost and CO2 capture performance.
Shell’s SBHP is a new way to produce blue hydrogen from natural gas and other fossil-based and biomass feedstocks – one that integrates proven proprietary and third-party technologies in an end-to-end line-up (see Figure 2). The process is an oxygen-based, non-catalytic system that utilises the organisation’s proven Shell Gasification Process technology, based on gas partial oxidation, to manufacture syngas, combined with Shell ADIP ULTRA pre-combustion CO2 capture technology.
When compared with conventional SMR and ATR technologies (see Figure 3), the SBHP has four key advantages. First, for large-scale projects (200+ tpd), oxygen-based systems such as the SBHP and ATR have a significant cost advantage over SMR systems. Moreover, the SBHP provides a potential levelised cost of hydrogen advantage – 25% lower than both SMR and ATR.
Second, the SBHP can capture as much as 99% of the CO2 from high-pressure, single-source, pre-combustion gas streams, which makes it the preferred choice for greenfield applications that require high capture rates.
Third, unlike ATR, the SBHP is non-catalytic, so does not require expensive gas pretreatment and can utilise multiple feedstocks, including natural gas, fuel gas, bottom of the barrel hydrocarbons, and renewable biomass.
Lastly, the SBHP produces high-pressure steam from waste process heat, which can be used in the carbon monoxide (CO) shift unit, or to supplement power demand, thereby increasing facility efficiency.
Blue hydrogen insights
Since launching the SBHP as an alternative to SMR and ATR in 2020, Shell has gained important insights into the status of the global blue hydrogen landscape.
Most of the projects that the organisation has been involved with have been in Europe (including the CIS) and North America, with growing interest in Asia and the Middle East (see Figure 4). From these projects, Shell has defined four project archetypes that describe the key, current applications for blue hydrogen (see Figure 5). The SBHP has several important advantages for these projects when compared with SMR and ATR.
Blue hydrogen and the oil, chemicals and gas industries
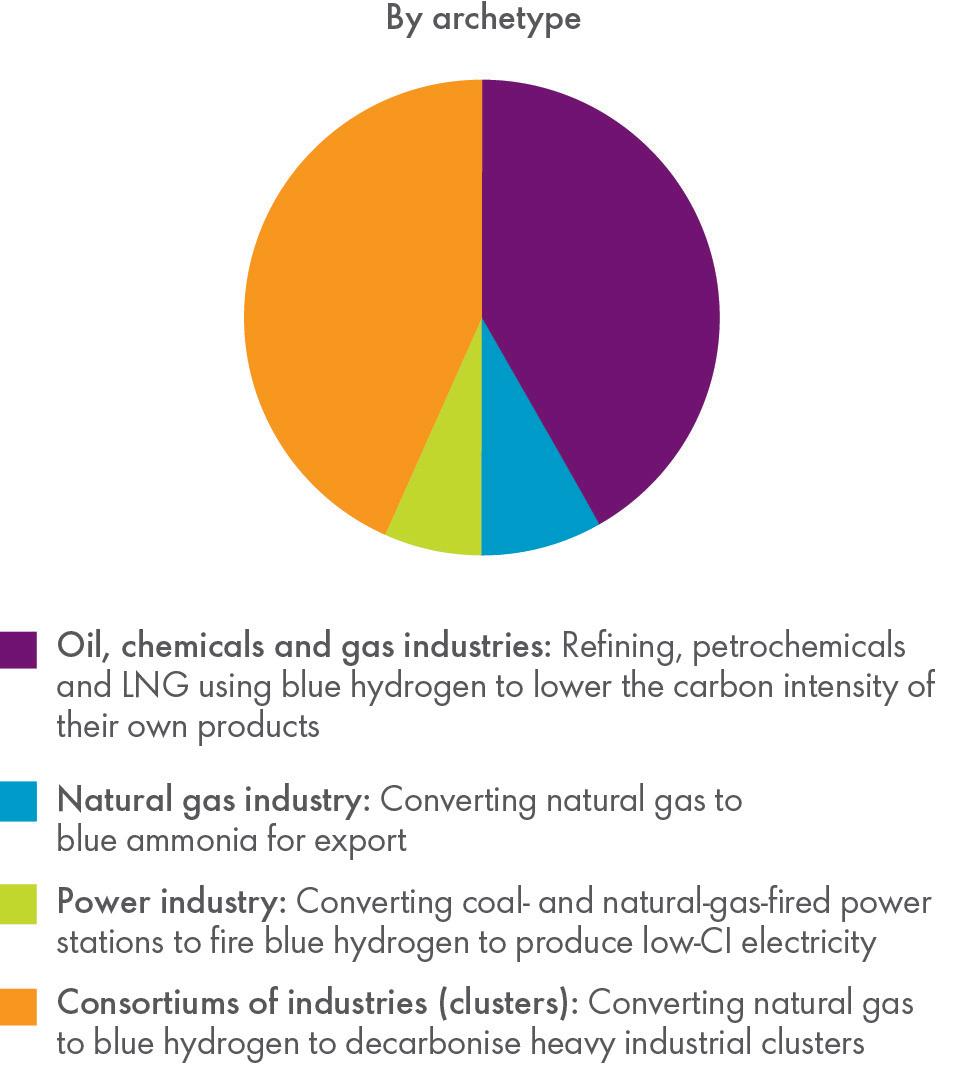
Conventionally, a refinery uses fuel gas or natural gas combined with post-combustion carbon capture technology to produce lower-carbon products. Post-combustion gases are, however, low pressure, must be captured from multiple locations, and typically have lower CO2 concentrations, thereby making carbon capture less efficient and more expensive.
Instead, companies are now looking to use conventional feedstocks in a centralised production facility to produce hydrogen that can be used to power furnaces and gas turbines, as well as directly in conversion processes. The advantage of this is that CO2 can be captured from high-pressure, pre-combustion gases from a single location, which is cheaper and can help to reduce Scope 1 emissions.
The SBHP has the advantage for this strategy because it: � Can capture CO2 from high-pressure, pre-combustion gas streams. � Can produce medium-pressure CO2 streams for easier transportation and storage. � Offers greater feed flexibility, including fuel gas, natural gas, biomass, and bottom of the barrel refinery products.
Blue hydrogen and the natural gas industry
Many natural gas exporters are looking to use their natural gas as a feedstock for blue ammonia, which is an ideal hydrogen carrier and provides a more efficient way of exporting hydrogen molecules to market. This strategy is particularly suited to locations where local natural gas production exceeds the local demand.
In this scenario, the SBHP has the advantage because it: � Can leverage high-pressure natural gas feeds and deliver high-pressure hydrogen that reduces the compression costs for ammonia production. � Is an oxygen-based technology, which means that the nitrogen produced by air separation during hydrogen production can be used to produce the blue ammonia. � Can capture as much as 99% of the CO2 emitted during hydrogen production, thereby lowering the carbon intensity of the ammonia and downstream products.
Figure 5. Top: the four blue hydrogen projects identified. Bottom: how the SBHP integrates with the four project archetypes.
Blue hydrogen and the power industry
Blue hydrogen demand from the power sector is currently relatively small. However, Shell expects large growth in this area as utility companies continue to seek lower-carbon solutions for power production. Consequently, companies
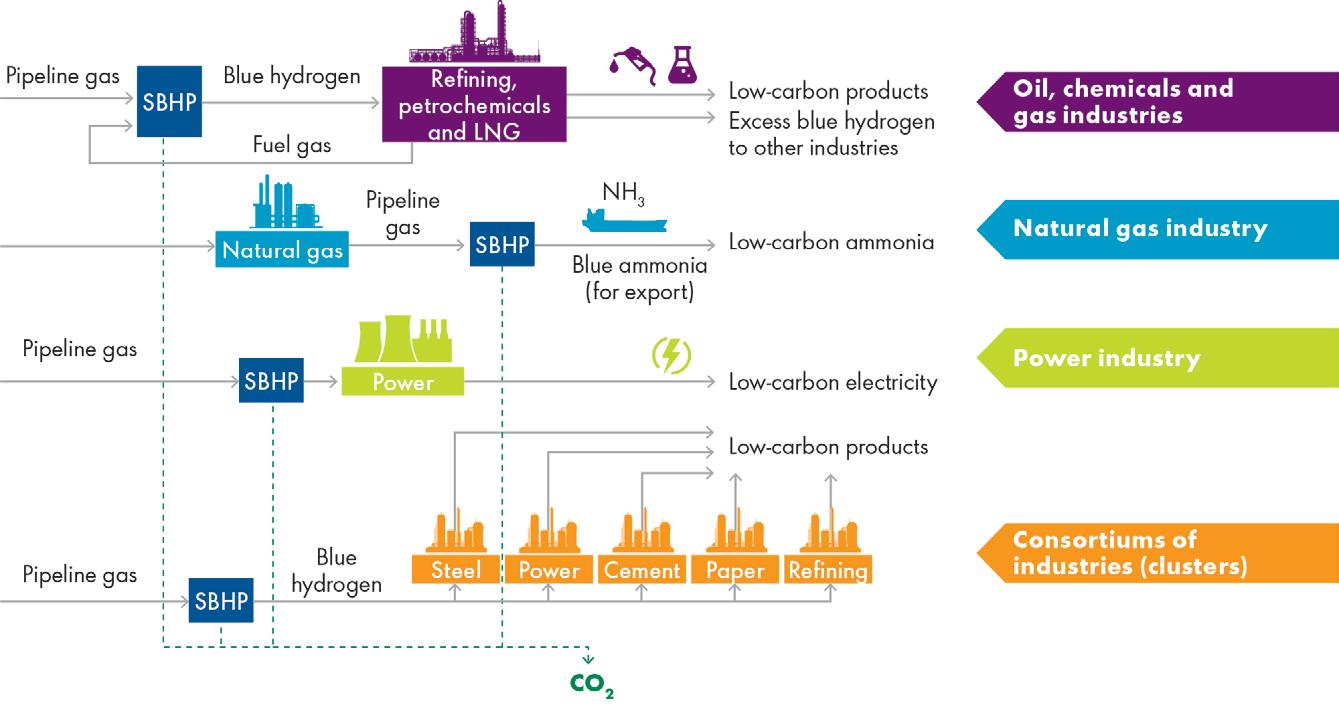
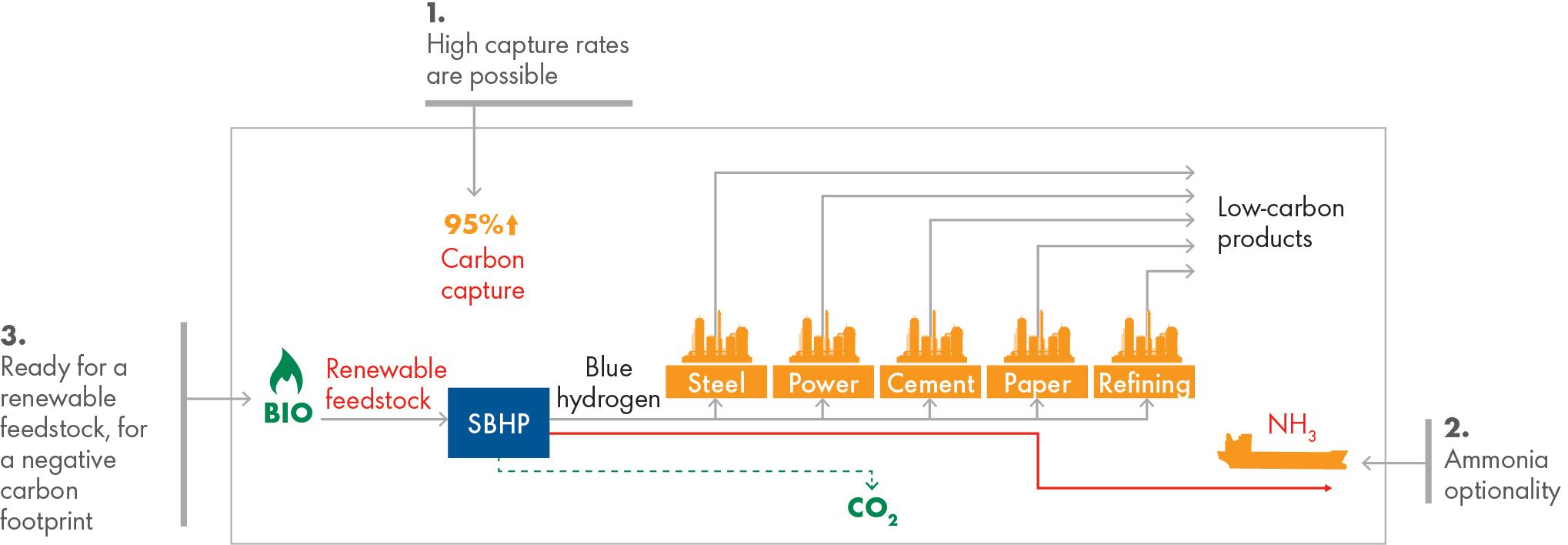
Figure 6. Three key roles that the SBHP can play in future-proofing industrial clusters.
are looking at blue hydrogen as an alternative to coal or natural gas.
The benefits of the SBHP for the power industry include: � The ability to produce hydrogen and capture CO2 at higher pressures and at the larger scales required to result in a lower levelised cost of hydrogen and electricity compared with SMR and ATR. � Improved power plant efficiency through the production and integration of valuable high-pressure steam into the power plant steam system.
Blue hydrogen and consortiums of industries
Industrial clusters, or hubs, are becoming an increasingly important concept as heavy industrial emitters look to develop collective, cost-effective decarbonisation pathways. For example, rather than each emitter developing its own blue hydrogen solution, clusters will develop a single, centralised hydrogen production unit that plugs into every facility.
The SBHP is well suited to this application for the following reasons: � The partial oxidation process on which it is based is proven on a large-scale, which reduces the project risks when substantial quantities of hydrogen are needed. � Its feed flexibility enables alternative feedstocks to be used, including waste gas generated by cluster partners. � It can leverage high-pressure gas feeds and deliver high-pressure hydrogen and CO2, which reduces the compression costs for transportation. � Its technology offers high process efficiency at high carbon capture rates that reduce the amount of CO2 produced per unit molecule of hydrogen. It also enables emitters to capture more CO2 at a lower cost.
Future-proofing industrial clusters
The SBHP can play three key roles in future-proofing blue hydrogen facilities (see Figure 6). First, it can help companies to remain aligned with increasingly strict carbon capture regulations. Currently, many projects require 90% carbon capture rates; however, in the near future, policymakers are expected to increase this requirement to 95%, and potentially more. Indeed, most of the new projects that Shell has been seeing are mandating capture rates of at least 95%.
Second, Shell’s technology provides the option for clusters to manufacture and export blue ammonia, thereby extending project life should the cluster switch to using green hydrogen.
And finally, the feed flexibility of the SBHP means that the natural gas feed can be replaced with a renewable feedstock such as biogas to create renewable (not green) hydrogen that is carbon negative.
Blue hydrogen and beyond
Shell is not just a technology provider and licensor; it is also a facility owner and operator with a commitment to reducing its impact on the climate. As such, it understands the challenges and concerns of plant owners and operators around the world. The hydrogen value chain is complex; however, Shell is well-positioned to play a leading role not only in supplying technology, but also in market and infrastructure development, and establishing robust supply chains and distribution networks.
The organisation is currently involved in and evaluating a number of hydrogen projects and solutions, both blue and green. A key part of this process is asking the right questions: what hydrogen capacity is needed? What is the quality of the feedstock? Does the facility need the flexibility to produce both hydrogen and ammonia to meet current and future market demands?
It may be several decades before zero-carbon, green hydrogen alone can meet demand. Nevertheless, Shell’s insights demonstrate that its blue hydrogen technology is ready to provide a cleaner and more cost-effective alternative to carbon-intensive grey hydrogen.
References
1. ‘The future of hydrogen: Seizing today’s opportunities’, IEA, (2019), https://iea.blob.core.windows.net/assets/9e3a3493-b9a6-4b7db499-7ca48e357561/The_Future_of_Hydrogen.pdf 2. ‘IEA Global Hydrogen Review 2021’, IEA, (2021), https://iea. blob.core.windows.net/assets/5bd46d7b-906a-4429-abdae9c507a62341/GlobalHydrogenReview2021.pdf 3. ‘Hydrogen Insights: A perspective on hydrogen investment, market development and cost competitiveness’, Hydrogen Council, (February 2021), https://hydrogencouncil.com/wp-content/ uploads/2021/02/Hydrogen-Insights-2021-Report.pdf 4. HOWARTH, R.W., and JACOBSON, M.Z., ‘How green is blue hydrogen?’, Energy Science & Engineering, Vol. 9, No. 10, (2021), pp. 1676 – 1687.