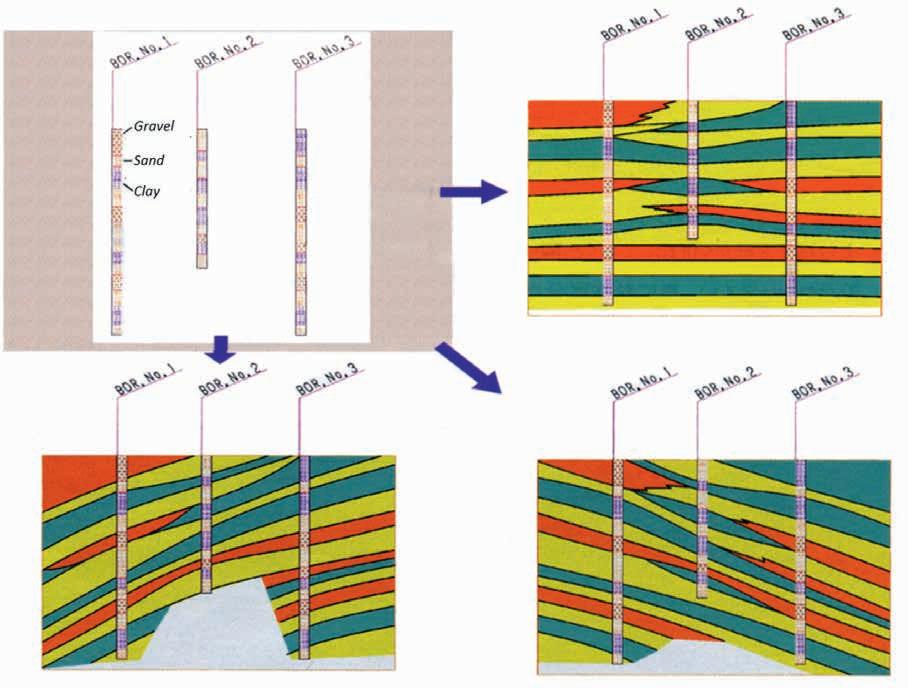
10 minute read
Use of geophysical surveys in geotechnical engineering practice — a geotechnical engineer’s perspective
Sugawara J.1,2,3* discusses the advantages of using a geophysical survey in geotechnical practice and key points that require attention.
Introduction
Advertisement
In geotechnical engineering practice, geotechnical investigation is one of the indispensable steps to identify the ‘fact’ of the subsurface ground conditions. Conventional geotechnical investigation includes boreholes, test pits, CPTs and field and laboratory tests. These days, geophysical surveys are also often used as a part of the geotechnical investigation to gain additional subsurface ground information that will contribute to the geotechnical analysis and design. Characterisation of subsurface ground conditions using geophysical survey techniques has been studied for a long time and there are many useful correlations between geophysical outputs and geotechnical items.
This paper describes typical situations where geotechnical engineers consider using geophysical surveys from the geotechnical engineering perspective. It also discusses advantages of using a geophysical survey and key points that require attention. The contents of this paper are based on the author’s experience across multiple sectors and most recently in a role with the Department of Transport and Main Roads, Queensland Government, Australia. The contents of this paper are purely based on the author’s opinion and experience.
Use of geophysical surveys in geotechnical engineering practice
Subsurface ground profiling
Prior to or during geotechnical analysis and design, geological and geotechnical sections / models are usually produced by interpolating and extrapolating discrete 1D geotechnical investigation results (eg. boreholes, test pits and CPTs) into the 2D or 3D space.
To increase the reliability of geotechnical design and the efficiency of construction works as well as to reduce geotechnical uncertainties, it is of importance to obtain a sufficient amount of subsurface ground information. The greater the amount of information gained, the better the understanding of the site is achieved, resulting in less conservatism in geotechnical design. However, due to resource, budget, time and technical constrains, it is often difficult to increase the quantity of geotechnical investigation.
For instance, most of the probe-type in-situ tests are not amenable to weathered rocks because of the presence of copestones and boulders in the decomposed matrix which obstruct or damage the probe when penetrating into the ground (Law 1979, Geotechnical Engineering Office 1988, Sugawara et al. 2004). The typical geotechnical investigation budget is approximately 4% of the overall project budget for small-to-medium size projects and could be up to around 8% for large projects in Australia. However, when the project budget is limited, geotechnical investigation could often be an easy target for budget saving. In such a case, the project may end up more costly due to unforeseen geotechnical risks not identified by the limited geotechnical investigation.
Furthermore, experience and good engineering judgment are pivotal when constructing a cross section with a limited quantity of geological and geotechnical data. This is because the subsurface ground conditions are inhomogeneous in nature, possibly causing significant variance within a short distance (eg. decomposition of weathered rocks). The final cross section can be significantly different depending on the interpretation of available information. Figure 1 (after Ohta 2002) shows an example of three cross sections drawn based on the data from three boreholes. These differences certainly have profound influence on the subsequent geotechnical analysis and design. For instance, foundation piles designed based on the inaccurate cross section can be too short or too long, the slope remediation design prepared based on incorrect slip surface could be too conservative or may not be effective at all.
In response to this fact, geotechnical engineers often consider the use of geophysical survey techniques when:
• Available geotechnical information is insufficient for producing an accurate cross section / model.
• A large area or long section needs to be investigated (such as road and rail projects) within a limited budget and timeframe.
• Access to the investigation location is restricted (eg. overwater or difficult terrain).
• No intrusive investigation is allowed due to existing underground structures or services, and / or environmental sensitivities.
The demand in the use of geophysical surveys has been increasing in geotechnical engineering practice. The author considers it would be further advantageous if:
• The geophysical survey can further classify geomaterials such as rock type (eg. igneous / sedimentary / metamorphic or even rock name) and soil type (eg. gravel / sand / silt / clay). Geotechnical engineers usually set up design parameters based on these categories because design parameters can be significantly different depending on material type.
• A 3D geophysical survey becomes more popular in geotechnical engineering space so that the 3D geophysical output can be directly transferred to a 3D geotechnical model. Although 3D output constructed by a compilation of 2D outputs is also useful, this still requires significant engineering judgment before use.
In addition, learning opportunities of geophysical survey techniques, ideally on an actual project, would be very beneficial for geotechnical engineers to understand the basics and advantages of geophysical surveys. The author observes that geotechnical engineers who have little or no exposure to the geophysical surveys often hesitate to incorporate the geophysical survey in the scope of geotechnical investigation works primarily due to their unfamiliarity with available techniques. Observation of actual geophysical survey works in the field could be an effective introduction to geotechnical engineers.
Classification of geomaterials and estimation of geotechnical parameters
In general geotechnical engineering practice, classification of geomaterials and setting of design parameters are based on results of geotechnical investigation (eg. boreholes, test pits, CPTs) and geotechnical field and laboratory tests. Depending on the purpose of geotechnical design, the mean or moderately conservative geotechnical design parameters may be selected. When the quantity of geotechnical investigations is limited due to various constraints, a geophysical survey can be used to supplement conventional geotechnical investigation.
The broad classification of geomaterials and parameter setting using geophysical results are well established (eg. The Society of Exploration Geophysicists of Japan 2014, US Army Corps of Engineers 2015, Caterpillar 2019), although these are typically based on empirical relationships of datasets with a large scatter. In the author’s opinion, these correlations are simple to use and are very useful tools to gain additional subsurface ground information. However, it is noted that in comparison to geotechnical parameters derived directly from the conventional geotechnical investigation, geophysical-derived geotechnical parameters are anticipated to have a larger degree of uncertaintiy due to the following reasons:
• While geotechnical parameters are often a direct measurement of geomaterials in the field and / or laboratory, geophysical survey usually requires the processing and interpreting of acquired raw data into meaningful geophysical parameters (such as P- and S-wave velocities). Therefore, the quality of geophysical outputs can be subject to the experience and skills of the person undertaking the data acquisition, processing and interpretation.
• Estimation of geotechnical parameters from geophysical outputs is often based on empirical relationships which usually contain scatter in the original database.
• In addition to the above-mentioned step, a few correlations may be required to obtain geotechnical design parameters from geophysical outputs. For instance, Standard Penetration Test (SPT) N values derived from P- or S-wave velocities may be used to estimate other geotechnical design parameters, such as shear strength and elastic modulus of soils, bearing capacity and pile capacity.
Therefore, care must be exercised when geophysically derived parameters are used in geotechnical analysis and design. It is always prudent to use geophysical data in combination with geotechnical data.
It would be advantageous if geophysical surveys can directly estimate typical geotechnical design parameters and relevant information such as unit weight, Atterberg limits, shear strength (drained and undrained), elastic modulus (drained, undrained, dynamic), consolidation parameters, stress history, shrink / swell potential, groundwater table, and location and shape of potential slip surface. However, this may not be an easy task and will require extensive research.
Investigation at access constrained site
Often geotechnical investigations are required to be undertaken in areas of standing water. However, depending on the site setting, the overwater drilling can be two to ten times more expensive than the land boreholes. In addition, such investigations are significantly affected by the weather, water depth, tide and availability of barges. Furthermore, it may take a long time to obtain required permits to undertake geotechnical investigation in watercourses as these are often in environmentally protected areas.
Similarly, borehole investigations are required as a part of slope remediation works in order to assess the subsurface conditions with particular regard to the geology and groundwater as well as the depth and nature of potential slip surfaces. However, the location of such borehole investigations could be limited to areas with relatively flat ground primarily due to the size of the commonly available drilling rigs and plant, Workplace Health and Safety requirements and general common practice and workplace culture are attributed to the accessibility of desired borehole locations. In addition, these borehole locations are often set outside of the landslide extent / slope failure (such as at or near the crest and / or toe of the slope) due to site access constraints. Since no subsurface ground and groundwater information can be often obtained along the sloping ground, the subsurface ground and groundwater information obtained from the investigation may not be representative of that which is found within the extent of the landslide / slope failure. Consequently, the designer often requires considerable interpolation / extrapolation of subsurface conditions, leading to a conservative slope remediation design. Furthermore. remediation works undertaken based on limited geotechnical information may encounter significant project delay and / or cost variation due to incorrect assumptions made in the remediation design.
Under such situations, the use of geophysical surveys could provide additional useful information required for geotechnical analysis and design within a relatively short timeframe. This would lead to more appropriate remediation design with greater certainty and without considerable interpolation / extrapolation of subsurface ground conditions as well as significant cost savings to construction and remediation works. Geophysical survey techniques have been successfully used to map landslides and identify the potential slip surface (eg. Sugawara et al. 2020, Komatina et al. 2020, Suto et al. 2018).
The author considers that the typical geophysical resolution of the depth scale which geotechnical engineers are interested in is usually appropriate for many geotechnical engineering applications, including identification of underground voids (Sugawara and Suto 2023) and the suitable socket layer for cast in place piles. However, the required resolution could be finer than the typical geophysical resolutions in some geotechnical applications. For instance, the thickness of the potential slip surface could be less than 10 cm for certain types of landslides (Figure 2). Higher resolution of such geophysical surveys would therefore be required to acquire these features.
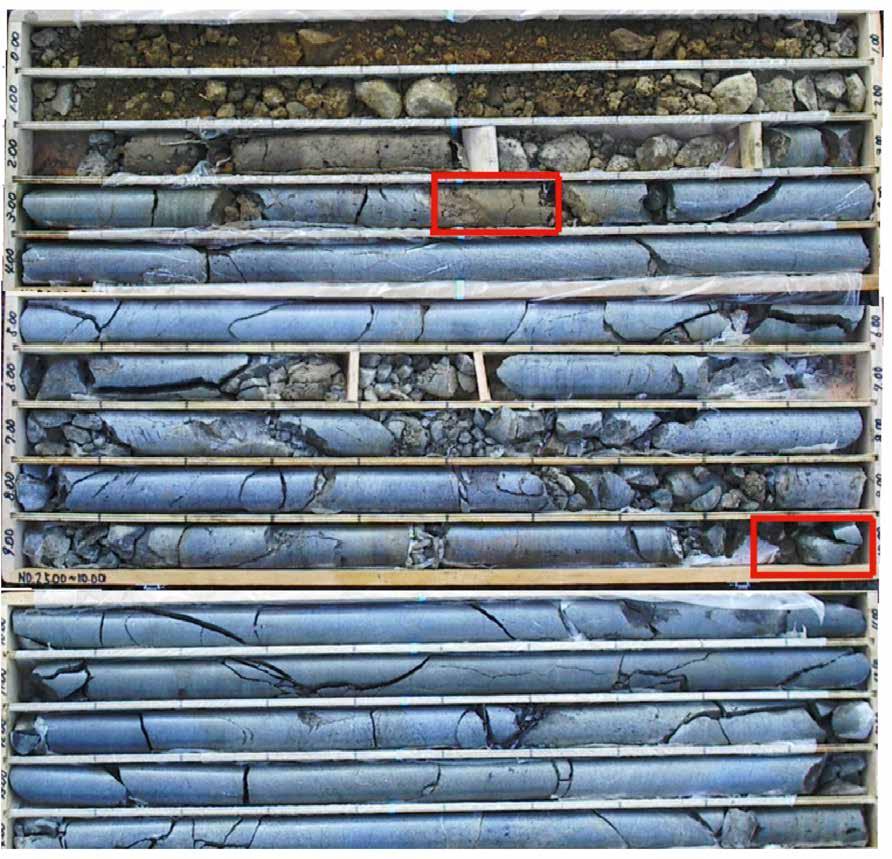
Confirmation of geotechnical requirements prior to the commencement of the geophysical survey would be therefore mandatory. In addition, the author considers that the use of geophysical surveys to identify the slip surface may not be effective in some geological settings (eg. the slip surface in fractured rock with little soil-like materials).
Excavation assessment
Selection of the most suitable machinery and equipment is of paramount importance in ground excavation works as it contributes to the maximum profitability of the project.
In geotechnical engineering practice, commonly used and well established excavatability assessment techniques include those proposed by Pettifer and Fookes (1994) and Tsiambaos and Saroglou (2010). These methods take into account the rock strength or weathering degree and spacing of discontinuities. These are used based on results of geological mapping, boreholes, test pits and laboratory tests.
In addition, the use of seismic velocity in these assessments is also popular (eg. Caterpillar 2019) and provides various advantages when:
• Rock outcrop is unavailable for geological mapping.
• Past geotechnical information is unavailable.
• Intrusive investigation is not allowed to be carried out.
• The subsurface ground conditions are significantly heterogenous.
• The large area or long section (such as linear corridor projects) needs to be investigated within a limited budget and timeframe.
The surface excavation work is one of the most common problems that may cause dispute between contractors and clients when they do not reach mutual agreement regarding the price of excavating rock and soil. This is because the terms ‘rock’ and ‘soil’ are loosely defined in the contract document (Mohamad et al. 2005). To minimise this risk, it is prudent that geotechnical / geological and geophysical outputs are calibrated to each other for better definition of subsurface ground conditions.
Summary
Geophysical surveys are very useful to supplement geotechnical investigation results that can be used in the subsequent geotechnical analysis and design. Geotechnical engineers consider using geophysical surveys under various constrained situations. While the correlations between various geophysical survey outputs and geotechnical data are generally based on empirical relationships with potentially large scatter in data, useful additional information on subsurface ground conditions can be obtained simply.
The demand of using geophysical surveys has been increasing in geotechnical engineering practice. In the author’s opinion, it would be further advantageous if:
• The geophysical survey can further classify geomaterials and estimate typical geotechnical design parameters and relevant geotechnical information.
• The 3D geophysical survey becomes more popular in the geotechnical engineering space.
• There are more project-based learning opportunities for geotechnical engineers to broaden their geophysical survey knowledge. This would increase geotechnical engineers’ confidence in using geophysical survey techniques as a part of geotechnical investigation works.
Acknowledgements
The author acknowledges support and advice of Mr Koya Suto from Terra Geophysica Australis during the preparation of this paper.
References
Caterpillar [2019]. Caterpillar Performance Handbook 49 Geotechnical Engineering Office. [1988]. Guide to rock and soil descriptions (GEOGUIDE 3), Geotechnical Engineering Office, Civil Engineering Department, Hong Kong Government.
Komatina S., Marković Juhlin M., Urošević M., Suto K. and Sugawara J. [2020]. An overview of multidisciplinary geophysical/geotechnical investigations of landslides in Serbia and B&H, First Break, 33-38, EAGE, August 2020.
Law, K.T. [1979]. Triaxialvane tests on a soft marine clay, Canadian Geotechnical Journal, 1979, 16(1), 1118.
Mohamad, E.T., Kassim, K.A. and Komoo, I. [2005]. An overview of existing rock excavatability assessment techniques, Journal Kejuruteraan Awam 17(2), 46-59.
Ohta, H. [2002]. Article, Nikkei Construction, 8(9), 2002, 70-75 (in Japanese).
Pettifer, G.S. and Fookes, P.G. [1994]. A revision of the graphical method for assessing the excavability of rock. Quarterly Journal of Engineering Geology, 27, 145-164.
Sugawara, J. and Suto, K. [2023]. Assessment of road failures using MASW technique, 2023, 5th Asia Pacific Meeting on Near Surface Geoscience & Engineering, Taipei, Taiwan (Accepted).
Sugawara, J. and Suto, K. [2020]. Landslide investigation using borehole and MASW techniques, 2020, 3rd Asia Pacific Meeting on Near Surface Geoscience & Engineering, Chiang Mai, Thailand.
Sugawara J., Yue Z.Q., Tham L.G., Lee C.F. and Law K.T. [2003]. Profiling of weathered rock slopes using drilling parameters. International Conference on Slope Engineering, December. Hong Kong, China.
Suto, K., Sugawara, J., Urosevic, M., Arsenovic, S. and Komatina, S. [2018]. Integrated geophysical and geotechnical analysis of landslide prone area – a case history in Lopare, Bosnia and Herzegovina, Near Surface Geoscience Conference and Exhibition 2018, European Association of Geoscientists & Engineers, September, Porto, Portugal. The Society of Exploration Geophysicists of Japan [2014]. Applications manual of geophysical methods to engineering and environmental problems, EAGE Publications BV, The Netherlands.
Tsiambaos, G. and Saroglou, H. [2010]. Excavatability assessment of rock masses using the Geological Strength Index (GSI), Bulletin of Engineering Geology and the Environment, 69, 13-27.
U.S. Army Corps of Engineers [2015]. Geophysical exploration for engineering and environmental investigations, U.S. Army Corps of Engineers.